Fig. 3.1
Normal cornea. Layers of the cornea include epithelium (ep), Bowman’s layer (bl), stroma (str), Descemet’s membrane (dm), and endothelium (et). The basement membrane (bm) of the corneal epithelium is a thin layer between the epithelium and Bowman’s layer, whereas the basement of the endothelium – the Descemet’s membrane – is thick and thickens with advancing age. Haematoxylin-eosin, bar = 50 μm
The cornea is both avascular and devoid of lymphatic drainage. It is supplied with glucose by diffusion from the aqueous humour. The central part of the cornea receives oxygen indirectly from the air via oxygen dissolved in the tear film, whereas the peripheral part receives oxygen by diffusion from the anterior ciliary circulation. Direct exposure of tears to the atmosphere is essential for oxygenation of the cornea. Aqueous humour provides the cornea with amino acids, ascorbic acid (vitamin C), and other vitamins, and also lactic acid. All these nutrients together with glucose are required for the normal metabolism of the cornea.
The cornea is one of the highly sensitive tissues of the human body. Density of nerve ending in the cornea is about 300 times that of the skin. The sensitivity of the cornea is 100 times that of the conjunctiva. An area of 0.01 mm2 of the cornea may contain as many as 100 nerve endings.
The cornea is thinnest at its centre, measuring about 550 μm, and thicker at the periphery, measuring about 700 μm. Because of tissue shrinkage, measurements taken from histological sections will be somewhat different.
3.2 Embryology, Anatomy, and Development
3.2.1 Embryology and Development
Soon after the lens vesicle has separated from the surface ectoderm (about fifth gestational week), the latter differentiates into a two-layered epithelium. By the end of week 6, junctional complexes appear between cells. During the next 1–2 weeks, the epithelium stratifies and becomes three to four cell layers thick, and the lens completes its formation and detaches from the surface ectoderm. Almost immediately after the separation of the lens, waves of neural crest cells migrate into the space between the lens and epithelium. These cells become the corneal endothelium and the stromal keratocytes [1]. The endothelium forms as a double layer of cuboidal cells. During week 8, they start to produce a basement membrane – Descemet’s membrane.
During month 3, fibroblasts and collagen fibrils appear. The fibroblasts start to synthesise a glycosaminoglycan-rich ground substance. Keratan sulphate production becomes apparent. Bowman’s layer is first identified during month 4; it develops as an extension of filaments from the basal lamina of the epithelium. It is also approximately this time that tight junctions form between the apices of the endothelial cells. Further development results in growth of the cornea and in dehydration of its stroma to form a transparent structure [2, 3].
3.2.2 Anatomy
The cornea consists of five layers: the epithelium, Bowman’s layer, the stroma, Descemet’s membrane, and the endothelium.
3.2.2.1 Epithelium
The corneal epithelium is composed of non-keratinised, stratified squamous epithelial cells. The thickness of the corneal epithelium is approximately 50 μm and makes up about 10 % of the total thickness of the cornea. It is constant over the entire corneal surface.
The epithelium consists of five to six layers of three different types of epithelial cells: two to three layers of superficial cells, two to three layers of wing cells, and a monolayer of columnar basal cells that adhere to the basement membrane overlying Bowman’s layer (Fig. 3.2).
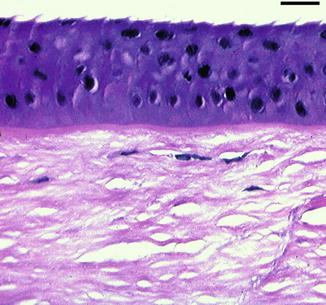
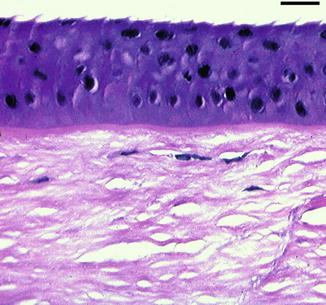
Fig. 3.2
Normal corneal epithelium. Haematoxylin-eosin, bar = 25 µm
Only the basal cells of the corneal epithelium proliferate. The daughter cells differentiate into wing cells and subsequently into superficial cells, gradually migrating to the corneal surface. This differentiation process requires 7–14 days, after which the superficial cells are desquamated into the tear film.
The epithelium and the tear film contribute to the maintenance of an optically smooth corneal surface. Another important physiological function of the corneal epithelium is to provide a barrier to external insults. The junctional complexes between adjacent epithelial cells prevent passage of chemical substances into the deeper layers of the cornea. Defects or loss of corneal epithelial cells result in penetration of fluid into the stroma, causing stromal edema [2, 4].
Cell-to-matrix and cell-to-cell communication are important for maintenance of the normal stratified structure and physiological functions of the corneal epithelium. Tight junctions are present mostly between cells of the superficial cell layer and, together with interdigitations, they provide an extremely effective barrier to prevent the penetration of fluid (tears). Zonulae adhaerentes and desmosomes are present in all layers of the corneal epithelium. However, gap junctions which allow the passage of small molecules between cells are found only in the wing cell and basal cell layers of the epithelium.
Components of the cytoskeleton, including actin filaments, microtubules, and intermediate filaments, contribute to the shape of all cells. Intermediate filaments are composed of specific types of acidic (type I) and basic (type II) cytokeratin molecules. The cytokeratin pair 3 and 12 (64 kDa keratin) is typically expressed in the epithelium of the cornea [2, 5].
Cellular components of the epithelium participate in corneal immunity. Dendritic cells of the epithelium, i.e. Langerhans cells, express human lymphocyte antigen (HLA) class II molecules and act by presenting antigens to T-cells. Langerhans cells are abundant in the peripheral corneal epithelium; the central corneal epithelium and stroma contain numerous dendritic cells, but the latter are HLA class II negative [6, 7].
3.2.2.2 Superficial Cell Layer
The superficial cell layer is two to three cells thick. The cells are flat and polygonal with a diameter of 40–60 μm and a thickness of 2–6 μm with horizontal nuclei. Their surface is covered with microvilli that form microplicae [8].
Two different types of superficial corneal epithelial cells are found by scanning electron microscopy: large dark cells and small light cells. The former are mature and have many microvilli, whereas the latter have fewer microvilli and are thought to be less mature.
Superficial cells are well differentiated; they do not proliferate, have a low metabolic activity, and contain fewer organelles and less RNA than the other types of corneal epithelial cells. The glycocalyx present at the anterior surface of the superficial epithelial cells interacts with the mucinous layer of the tear film and helps to maintain the normal trilayered structure of the tear film.
3.2.2.3 Wing Cell Layer
The wing cell layer constitutes the middle zone cells that are polyhedral with convex anterior surfaces and concave posterior surfaces (the characteristic wing-like shape) [2–4]. Their nuclei are oval to round. Multiple desmosomes attach the cells to their neighbours. The lateral borders of the cells show many interdigitations. The presence of numerous gap junctions permits free intercellular communication in this zone.
3.2.2.4 Basal Cell Layer
The deepest zone, basal cells are tall columnar cells that form a single layer resting on a basement membrane [2–4]. Of all corneal epithelial cells, only the basal cells show mitotic activity. Basal cells are the source of wing cells and superficial cells. Basal cells contain more intracellular organelles, free ribosomes, rough endoplasmic reticulum, mitochondria, centrioles, microfilaments, microtubules, and glycogen granules than do wing or superficial cells. Their lateral borders interdigitate with one another and are attached by desmosomes, gap junctions, and zonulae adhaerentes. Hemidesmosomes attach the basal cells to the basement membrane. Hemidesmosomes are joined with anchoring fibrils, composed of type VII collagen, that pass through the basement membrane and Bowman’s layer. After multiple branchings in the stroma, they form anchoring plaques together with type I collagen, a main component of the stroma. The anchoring fibrils are crucial for providing the adhesion of basal cells to the basement membrane and stroma.
3.2.2.5 Basement Membrane
Corneal basal epithelial cells secrete the components essential for basement membrane formation. Type IV collagen and laminin, which are both produced by basal cells, are the main components of the epithelial basement membrane. Transmission electron microscopy shows that the basement membrane (40–60 nm thick) is composed of two layers: a pale layer (lamina lucida) located just posterior to the cell membrane of the basal cells and an electron-dense layer (lamina densa). The corneal epithelial basement membrane contains type IV (α5) and VII, laminin-1, laminin-5, fibronectin, heparan sulphate proteoglycans, and fibrin.
3.2.2.6 Bowman’s Layer
Bowman’s layer lies directly beneath the basement membrane of the corneal epithelium, between the corneal epithelium and the corneal stroma proper. It measures 8–12 μm in thickness. It is an acellular structure consisting of randomly arranged collagen fibres and proteoglycans. The collagen fibres of Bowman’s layer are primarily type I and III collagen and measure 20–30 nm in diameter. These fibres are finer and more randomly arranged than those in the corneal stroma proper. Bowman’s layer is considered to be the anterior border of the corneal stroma. It does not regenerate after injury [2, 4].
3.2.2.7 Stroma
The stroma forms the largest portion, more than 90 % of corneal thickness. It consists of extracellular matrix, keratocytes (corneal fibroblasts), and nerve fibres. The cellular components form only 2–3 % of the total volume of the stroma [9]; the remaining space is occupied mostly by the extracellular matrix components: collagen and glycosaminoglycans. Collagen makes up more than 70 % of the dry weight of the cornea. The stroma is composed mainly of type I collagen, with smaller amounts of types III, V, and VI [10]. Stromal collagen fibres are identical in diameter (22.5–35 nm) and the distances between them are also identical (41.4 nm) [9]. The direction of the collagen fibres in any given lamella is the same, but they run at right angles to those of adjacent lamellae. Such a regular distribution of collagen fibres and lamellae is the main determinant of corneal transparency. Recently, the existence of a distinct pre-Descemet’s layer was proposed [11].
Various glycosaminoglycans can be found between the collagen fibres of the corneal stroma. Keratan sulphate constitutes about 65 % of the total glycosaminoglycan content and is the most abundant glycosaminoglycan in the cornea. The other glycosaminoglycans are chondroitin sulphate and dermatan sulphate.
Glycosaminoglycans bind to core proteins to form proteoglycans, which are thought to modulate collagen fibrillogenesis. Lumican, keratocan, and mimecan (osteoglycan) are present in the corneal stroma as keratan sulphate proteoglycans and decorin as a chondroitin sulphate or dermatan sulphate proteoglycan. These core proteins first accumulate as low-sulphate glycoproteins in the embryonic stroma and subsequently bind glycosaminoglycans to form proteoglycans in the adult cornea.
Keratocytes form the main cellular component of the corneal stroma and are thought to turn over about every 2–3 years. Keratocytes have a spindle shape and they are scattered between the lamellae of the stroma. These cells extend long processes that are connected with processes of neighbouring cells by gap junctions [4].
Keratocytes are similar to fibroblasts and have an extensive intracellular cytoskeleton, including prominent actin filaments [5]. This allows the cells to contract and may be responsible for the maintenance of corneal shape and for the packed structure of collagen in the stroma [4].
Some cellular components of the corneal stroma play an important role in corneal immunity. Recent studies have revealed that the corneal stroma is endowed with significant numbers of resident inflammatory and antigen-presenting cells. This includes bone marrow-derived dendritic cells and macrophages. These dendritic cells present in the stroma do not express HLA class II molecules [6, 7].
3.2.2.8 Descemet’s Membrane
Descemet’s membrane is the basement membrane of the corneal endothelium and lies on the posterior surface of the stroma. It gradually increases in thickness from birth (3 μm) to adulthood (8–10 μm). Histological analysis shows Descemet’s membrane to be stratified into a thin (0.3 μm) non-banded layer adjacent to the stroma, an anterior banded zone (2–4 μm), and a posterior amorphous, non-banded zone (less than 4 μm) that represents up to two-thirds of the thickness of the membrane and is deposited over time [4]. Descemet’s membrane is composed mainly of type IV collagen fibrils arranged in a hexagonal pattern. It contains also laminin and fibronectin.
Descemet’s membrane adheres tightly to the posterior surface of the corneal stroma and reflects any change in the shape of the latter. If the corneal stroma swells, Descemet’s folds can be observed clinically. Descemet’s membrane as such does not regenerate, but if endothelial cells migrate over the bare stroma after a Descemet’s tear, new membrane will be deposited, and once it covers the injured area, stromal edema decreases [2, 4, 7].
3.2.2.9 Endothelium
The corneal endothelium consists of a single layer of flattened, uniformly 5 μm thick and 20 μm wide, polygonal (mostly hexagonal) cells, arranged into a mosaic pattern. Endothelial cells contain a large nucleus, numerous mitochondria, a prominent endoplasmic reticulum, free ribosomes, and a Golgi apparatus, which indicates that the cells are metabolically active and secretory. The anterior surface of endothelial cells is flat and adheres to Descemet’s membrane. The posterior surface forms microvilli and marginal folds that protrude into the anterior chamber, thus maximising the surface area exposed to aqueous humour. The endothelial cells contain various junctional complexes: zonulae occludentes, maculae occludentes, and maculae adhaerentes, but not desmosomes. Gap junctions allow the transfer of small molecules and electrolytes between the endothelial cells.
Endothelial cells play a major role in controlling the normal hydration of the cornea, both by a permeable barrier function, limiting access of water from the aqueous humour to the corneal stroma, and by an active transport mechanism. Essential to this energy-determined process is the role of Na/K-ATPase and carbonic anhydrase. Bicarbonate ions produced by the action of carbonic anhydrase are translocated across the cell membrane, allowing water to passively follow. Loss or damage of endothelial cells results in increased absorption of water by the corneal stroma [2, 4].
Corneal endothelial cells do not proliferate in humans (unlike in rabbits). Thus, in the normal healthy cornea, the endothelial cell density decreases with age [12]. Between the ages of 20 and 80 years, the reduction in cell density averages 0.6 % per year, with concomitant increases in polymegethism (variation in size) and pleomorphism (variation in shape).
3.2.2.10 Blood Supply and Lymphatic Drainage
Under normal conditions the cornea does not contain any blood vessels. It is also devoid of lymphatic drainage.
3.2.2.11 Nerve Supply
The cornea is one of the highly sensitive tissues of the human body. It is primarily innervated through one of three ophthalmic branches of the trigeminal nerve. The ophthalmic division of the trigeminal nerve has three parts: the frontal nerve, the lacrimal nerve, and the nasociliary nerve. The nasociliary nerve provides sensory innervation to the cornea, mainly through the long ciliary nerves. The long ciliary nerves enter the eye around the optic nerve, then run anteriorly in the suprachoroidal space. A short distance from the limbus, they pierce the sclera, divide, connect with each other and conjunctival nerves, and form the pericorneal (annular) plexus of nerves.
Approximately 60–80 myelinated branches enter the corneal stroma. Further division occurs, and the fibres lose their myelin sheaths. Next they unite to form the stromal plexus located in the midstroma. Subsequently, nerve fibres pass anteriorly and form the subepithelial plexus. Fine terminal branches pierce Bowman’s layer and pass between the epithelial cells to form the intraepithelial plexus. Specialised nerve endings are absent. The axons are bare and devoid of a Schwann cell sheath [2, 13].
3.2.2.12 Limbal Stem Cells
Corneal epithelial cells renew rapidly and repeatedly in order to maintain a stratified squamous epithelium. The maintenance of the corneal epithelial cells is accomplished by a defined population of unipotent stem cells located in the basal epithelium of the corneoscleral limbus within so called “palisades of Vogt”. The limbus (corneoscleral junction) is a specific and unique area, which is highly innervated, vascularised, and protected from potential damage from ultraviolet light by the presence of melanin pigmentation [7, 14]. Limbal stem cells are supported by a unique stromal microenviroment called the “stem cell niche”, which consists of certain extracellular matrix components, cell membrane-associated molecules, and cytokines [15].
Limbal stem cells are the precursors for all other cells of the corneal epithelium and have a self-maintaining population. In vivo, they show slow cycling, but in vitro they demonstrate a high potential to proliferate [8, 14]. Stem cells in the limbus divide to produce a daughter stem cell and a transient amplifying cell. The transient amplifying cells migrate within the cornea to reside in the basal layer of corneal epithelium. Further cellular divisions of the transient amplifying cells produce post-mitotic cells that lie in the suprabasal layers. Progressive differentiation of post-mitotic cells produce terminally differentiated cells, which lie in the superficial corneal epithelial layers. These terminally differentiated cells are non-keratinised, stratified, squamous corneal epithelial cells. These cells are continually sloughed off the corneal surface and replaced by maturing, underlying cell layers [7, 14]. A number of molecular markers have been used to characterise limbal stem cells: ABCG2, p63, and cytokeratins 14/5 and 19 [5, 7].
3.3 Congenital Abnormalities
3.3.1 Abnormalities of Corneal Size
3.3.1.1 Microcornea
By definition, a cornea with a diameter less than 11 mm is designated microcornea.
3.3.1.2 Megalocornea
By definition, a cornea with a diameter more than 13 mm is designated megalocornea.
3.3.2 Abnormalities of Corneal Shape
3.3.2.1 Cornea Plana
Definition
Cornea plana, literally flat cornea, is an inherited bilateral abnormality characterised by a reduced corneal curvature.
Epidemiology
A very rare congenital anomaly.
Etiology and Genetics
Clinical Findings
Histopathology
Prognosis
CNA1 is mild but CNA2 is severe. Nonprogressive, but carries a risk for glaucoma and, rarely, corneal decompensation.
3.3.2.2 Sclerocornea
Sclerocornea is a poorly defined congenital condition in which the cornea is replaced by whitish tissue resembling sclera in texture and curvature [24]. It is not a single entity and can be associated with a number of different defined and undefined anomalies of the eye and other organs. It was recently proposed that this diagnosis should no longer be used as a primary diagnosis [25].
3.3.3 Abnormalities of the Anterior Segment of the Eye with Corneal Involvement
3.3.3.1 Axenfeld-Rieger Syndrome
Definition
Axenfeld-Rieger syndrome is an inherited disorder of the morphogenesis of the peripheral cornea, the iris, and the chamber angle often associated with glaucoma.
Epidemiology
A rare congenital anomaly.
Etiology and Genetics
The syndrome results from autosomal dominant mutations in PITX2 (RIEG1, OMIM #180500) encoding paired-like homeodomain transcription factor 2, an unknown gene on chromosome 13 (RIEG2; OMIM %601499) or FOXC1 (RIEG3; OMIM #602482) encoding forkhead box C1, a transcription factor [26, 27], which controls corneal vascularisation in mice [28]. Axenfeld-Rieger syndrome may result from a late developmental arrest of neural crest-derived anterior ocular structures.
Clinical Characteristics
A combination of an anteriorly displaced Schwalbe’s line (posterior embryotoxon) and iris bands attached to the peripheral cornea (Axenfeld’s anomaly), associated glaucoma from a defective trabecular meshwork (Axenfeld’s syndrome), associated iris and pupillary abnormalities (Rieger’s anomaly), and associated hypoplasia of the maxilla and dental anomalies including anodontia, oligodontia, microdontia, or peglike incisors (Rieger’s syndrome) is variably present [29, 30]. Cardiac, craniofacial, and pituitary abnormalities also may be present [27, 31].
Histopathology
Prognosis
Nonprogressive, but expressivity varies, and often complicated with chronic glaucoma.
3.3.3.2 Peters’ Anomaly
Definition
Peters’ anomaly is a developmental defect of the cornea, the iris, and the lens and may be associated with additional ocular anomalies.
Epidemiology
A rare congenital anomaly.
Etiology and Genetics
Peters’ anomaly (OMIM #604229) results from either mutated paired box 6 (PAX6), paired-like homeodomain transcription factor 2 (PITX2), cytochrome P450, subfamily I, polypeptide 1 (CYP1B1), forkhead box C1 (FOXC1) or forkhead box E3 (FOXE3) gene [25], or fetal alcohol syndrome. Peters-plus syndrome (OMIM #261540) adds distinctive facies, cleft lip and palate, short hands and feet, and other anomalies and results from mutated beta-1,3-galactosyltransferase-like (B3GALTL) gene [32]. Inheritance is autosomal recessive, but rare dominant transmission is also reported.
Clinical Features
In type 1, a centrally located, avascular corneal opacity involves the posterior stroma, Descemet’s membrane, and endothelium and is associated with iridocorneal adhesions [24, 33–35] that are best resolved with anterior segment optical coherence tomography [36]. In type 2, the lens has failed to separate and is adherent to or incarcerated within the cornea, which is vascularised (Fig. 3.3a–c).
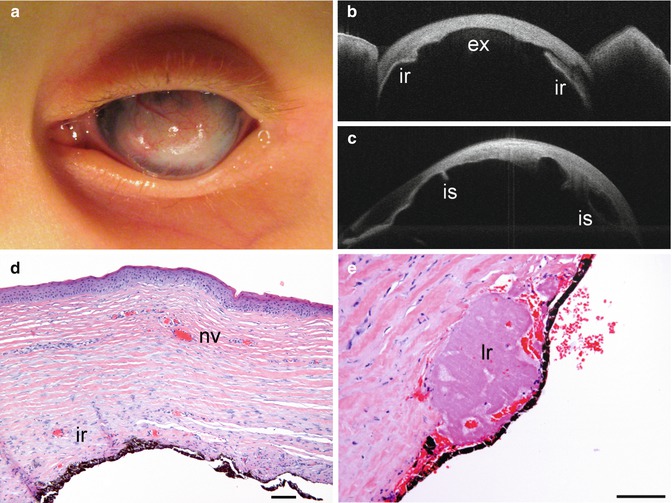
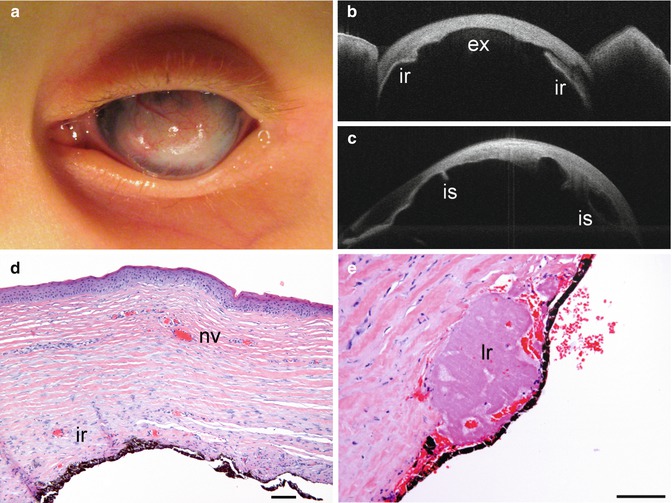
Fig. 3.3
Peters’ anomaly. Congenitally opaque, vascularised cornea that prevents evaluation of iris structures (a). Anterior segment optical coherence tomography reveals a concave posterior corneal excavation (ex), a partly adherent iris (ir) (b), and iris strands (is) that attach to the cornea (c). Corneal epithelium is keratinised from exposure, Bowman’s layer is absent, the loosely arranged stroma has neovascular vessels (nv), and the iris (ir) adheres to the corneal stroma without an intervening Descemet’s membrane (d). A lens remnant (lr) is incarcerated within the posterior corneal stroma and under a rudimentary iris epithelium; note haphazard corneal stromal lamellae with abundant extracellular material (e). Haematoxylin-eosin (d, e), bars = 100 μm
Histopathology
A concave defect of the posterior layers of the cornea is present and the stroma borders directly to the anterior chamber within this area without Descemet’s membrane or endothelium and shows irregular collagen lamellae with plump fibroblasts (Fig. 3.3d, e) [37–40]. The anterior central stroma is cloudy and swollen. Bowman’s layer ranges from abnormally thick to absent. Iridocorneal adhesions often are found at the rim of the corneal defect.
3.3.3.3 Posterior Keratoconus
3.3.3.4 Congenital Anterior Staphyloma
Definition
Congenital anterior staphyloma is a developmental defect of the anterior ocular segment characterised by a cloudy, bulging, vascularised, staphylomatous cornea.
Synonyms
Congenital corneal staphyloma.
Epidemiology
A very rare congenital anomaly.
Etiology
An early developmental arrest of neural crest-derived anterior ocular structures is presumed. No underlying gene has been identified.
Clinical Features
The anterior segment abnormalities include a central corneal opacity, a sclerocornea, a hypoplastic iris that adheres to the cornea, a hypoplastic ciliary body, and a rudimentary or absent lens (Fig. 3.4a, b) [45–52]. The posterior segment is normal but the fellow eye is usually microphthalmic. Extraocular anomalies are rarely associated with this condition.
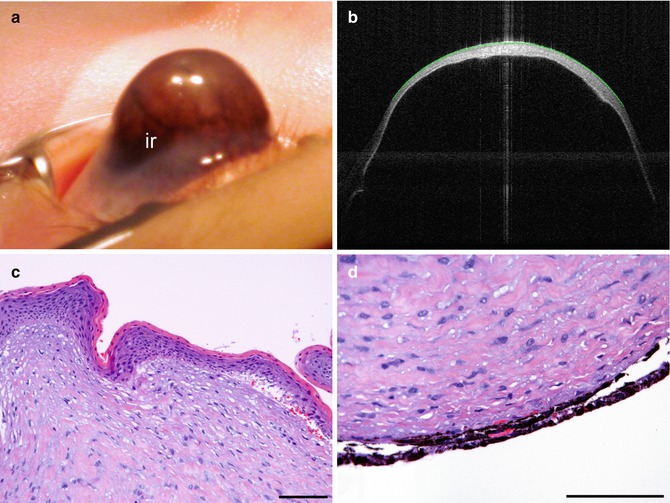
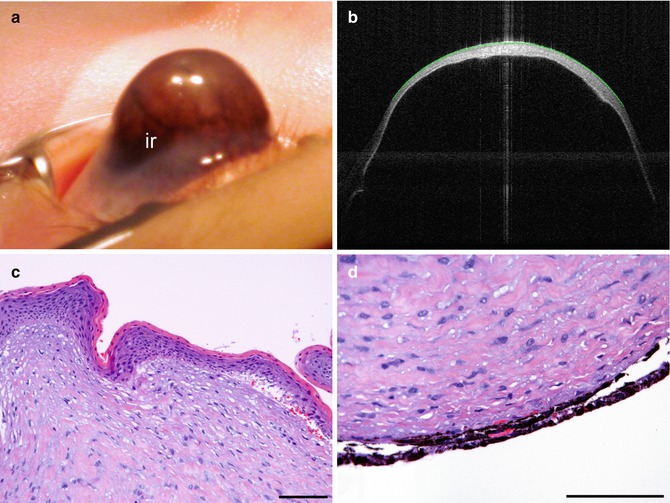
Fig. 3.4
Congenital anterior staphyloma. Grossly bulging cornea with attached stretched iris (ir) on its posterior surface in a newborn baby (a). Anterior segment optical coherence tomography reveals extreme thinning of the cornea with swelling in its centre (b). Corneal epithelium is keratinised from exposure, Bowman’s layer is absent, and corneal stroma is replaced by haphazardly arranged fibroblasts and thin, wavy collagen strands (c). Descemet’s membrane and iris stroma are absent, and the primitive iris epithelium adheres to the cornea; note inconspicuous corneal lamellae with abundant intervening fibroblasts (d). Haematoxylin-eosin (c, d), bars = 100 μm
Histopathology
The cornea ranges from thin to thick, depending on stretching of the staphyloma [45–52]. The corneal epithelium is hyperkeratotic and the stroma swollen with variable degrees of inflammation from exposure. Bowman’s layer and Descemet’s membrane are typically absent (Fig. 3.4c, d). The stroma has irregular lamellae and plump fibroblasts. The neuroepithelial layers of the iris adhere to the cornea with little or no intervening iris stroma.
Prognosis
Corneal transplantation may enhance cosmesis and partially restore vision, but long-term prognosis is guarded.
3.3.3.5 Congenital Keratectasia
Congenital keratectasia may resemble congenital anterior staphyloma but differs from it by the presence of normal corneal layers and absence of adherent iris. It is postulated to be secondary to intrauterine keratitis or nutritional deficiency.
3.4 Inflammation
3.4.1 General Considerations
Corneal inflammation is a non-specific result of tissue damage. Direct injury from foreign bodies and chemical or thermal burns can elicit inflammation. The two most common etiologies, however, are microbial infections and various immunologic conditions [53]. Macrophages that process antigens and polymorphonuclear leucocytes with destructive proteolytic enzymes are relevant factors that initiate the inflammatory response. Antigens lead to an immune response, and B- and T-lymphocytes are responsible for subsequent antibody and cytotoxic reactions, respectively. Leucocytes migrate toward the site of the initiating inflammatory stimulus, following interlamellar pathways of the corneal stroma, and produce irregularities in the alignment of its lamellae [54].
Stromal swelling typically accompanies inflammation and leads to an increase in corneal thickness from accumulation of fluid within and between the stromal lamellae, swelling of keratocytes, and influx of inflammatory cells. Histopathologically, artifactual separation of lamellae should not be mistaken for stromal edema; true swelling actually tends to decrease such separation. The swollen stroma is less eosinophilic and less birefringent under polarised light. The source of the fluid varies: tears in superficial lesions, leaking limbal vasculature in anterior and midstromal lesions, and aqueous humour in posterior lesions [54].
The initial vascular reaction to corneal inflammation is perilimbal injection, which may extend around the entire limbus. The limbal vasculature contains anastomotic connections between the superficial vessels, derived from the conjunctival circulation, and the deep vessels, derived from the anterior ciliary-episcleral circulation connected to the iris vasculature. Therefore, reflex dilatation of iris vessels, iritis, and hypopyon formation in the anterior chamber can accompany keratitis.
The combination of swelling, cellular infiltration, and lamellar distortion interferes with light transmission, and, hence, with transparency of the cornea [54].
3.4.1.1 Corneal Ulceration and Sequelae
Corneal stromal ulceration is of great clinical significance as it is frequent, and difficult to treat, and leads to considerable ocular morbidity and sight-threatening sequelae. Relevant cellular events and interactions include a preceding epithelial defect, inflammatory cell migration, release of collagenases and other hydrolases by injured corneal epithelium, keratocytes and polymorphonuclear leucocytes, activation of latent collagenases, tissue necrosis, and failure of normal wound healing processes [55].
Scar Formation (Macula and Leucoma)
Healing of a corneal wound begins when epithelial cells migrate into the ulceration from its margins. Limbal vessels and reactive stromal fibroblasts grow beneath the newly formed epithelium; macrophages assist in removing cell debris. A connective tissue scar, known as a macula or a leucoma depending on its extent, begins to form, which interferes with transparency. On microscopic examination, clinically visible scars may not be evident at all or will only correspond to minor irregularities in stromal fibre arrangement. Routine haematoxylin-eosin stain shows keratocytes with large, darkly staining nuclei and a more eosinophilic cytoplasm than those among older corneal fibres. Bowman’s layer in deeper scars is often replaced by connective tissue [56].
Keratectasia
A keratectasia, an ectatic corneal scar, is defined as bulging of a thinned, scarred cornea. It differs from a staphyloma in that uveal tissue does not line the scar.
Descemetocele
Sloughing of the inflamed, sometimes infected, corneal stroma may lead to severe thinning of the stromal layers. Descemet’s membrane, on the other hand, is quite resistant to inflammation and necrosis and will often remain intact after loss of most of the stroma (Fig. 3.5a). A herniation of this extremely elastic membrane through a corneal ulcer is known as a descemetocele that may be covered by fibrinous exudate, a few posterior stromal lamellae, and corneal epithelium (Fig. 3.5b). It may rupture as a result of intense stretching, enzymatic digestion, or sudden increase in intraocular pressure [56].
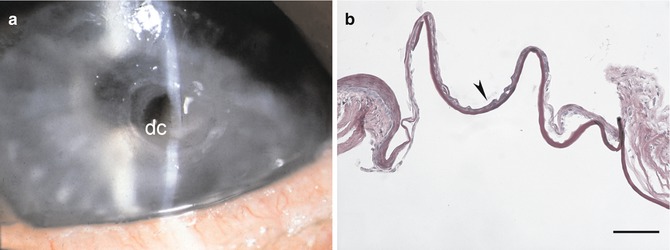
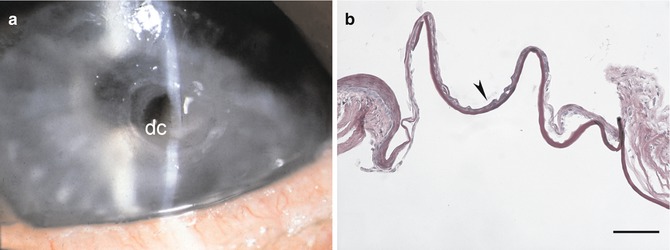
Fig. 3.5
Descemetocele. Sterile descemetocele (dc) in a patient with rheumatoid arthritis (a). Histopathology of descemetocele with remnants of epithelium (arrowhead), nearly complete loss of stroma, and bulging of Descemet’s membrane that is devoid of endothelial cells (b). Periodic acid-Schiff, bar = 100 μm
Adherent Leucoma
After penetrating corneal injury or spontaneous perforation of an ulcer, an adherent leucoma can form. It typically consists of a dense corneal scar with adherent intraocular tissue such as iris, lens material, vitreous, or even retina.
3.4.1.2 Corneal Neovascularisation
The cornea is one of the few tissues which actively maintain an avascular state, i.e. the absence of blood and lymphatic vessels (corneal [lymph]angiogenic privilege). Neovascularisation of the cornea can follow numerous inflammatory diseases of the anterior segment including trachoma, luetic and viral interstitial keratitis, microbial keratitis, and the immune reaction elicited by corneal transplantation. Diapedesis of leucocytes into the stroma with extravasation of fibrin and other serum proteins is followed by vascular endothelial migration and proliferation from the limbus adjacent to the site of inflammation. Subepithelial vascular growth (superficial neovascularisation) is typically present with superficial lesions. In deep neovascularisation, buds of endothelial cells emerge from limbal capillaries, extend between stromal lamellae, and become canalised thereafter (Fig. 3.6) [56]. The extent of neovascularisation depends on the severity and extent of the inflammatory focus and its duration. As the inflammation subsides, the vascular channels regress and are seen clinically as non-perfused “ghost vessels”.
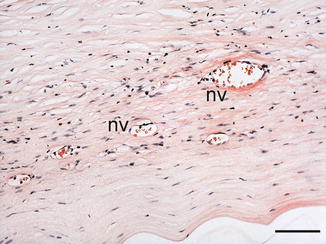
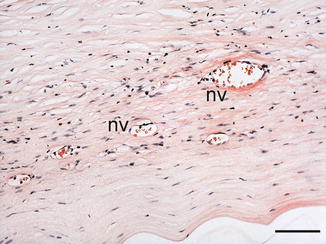
Fig. 3.6
Corneal neovascularisation. Scar formation with deep stromal neovascular vessels (nv). Haematoxylin-eosin, bar = 100 μm
Ingrowth of blood and lymphatic vessels into the cornea not only reduces corneal transparency and thereby visual acuity, but also significantly increases the rate of graft rejection if corneal transplantation becomes necessary [57].
3.4.1.3 Corneal Lymphangiogenesis
The normal cornea is not only avascular but also devoid lymphatic vessels, thus allowing for its unique immune privileged status. It can, however, acquire lymphatic vessels secondary to a variety of corneal diseases and surgical manipulations. Whereas corneal hemangiogenesis is obvious both clinically and histologically, detection of associated corneal lymphangiogenesis has long been hampered by virtual invisibility of lymphatic vessels and lack of specific markers. This has changed with the recent discovery of lymphatic endothelial markers: vascular endothelial growth factor receptor 3, lymphatic endothelium-specific hyaluronan receptor-1 (LYVE-1), Prox 1, and podoplanin. Corneal inflammation and wound healing are now known to be typically associated with lymphangiogenesis by vascular endothelial growth factor (VEGF-C/-D/VEGFR-3)-mediated mechanisms [57–59].
3.4.2 Ocular Surface Disease
Ocular surface disease is a common clinical entity but will only rarely be seen by the ophthalmic pathologist.
3.4.2.1 Dry Eye Syndrome
Definition
The Dry Eye WorkShop has defined dry eye syndrome as a multifactorial disease of the tears and ocular surface that results in symptoms of discomfort, visual disturbance, and tear film instability with potential damage to the ocular surface [60]. This syndrome is accompanied by increased osmolarity of the tear film and inflammation of the ocular surface (Fig. 3.7a). It is typically associated with older age, female gender, and certain systemic diseases such as Sjögren’s syndrome.
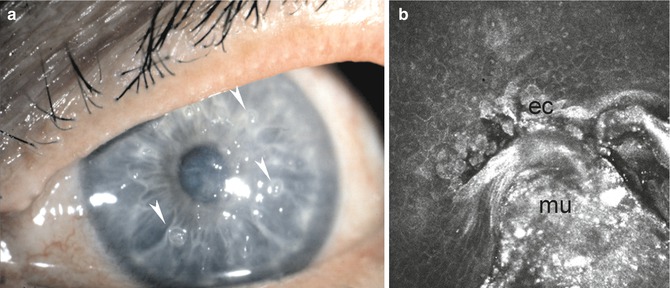
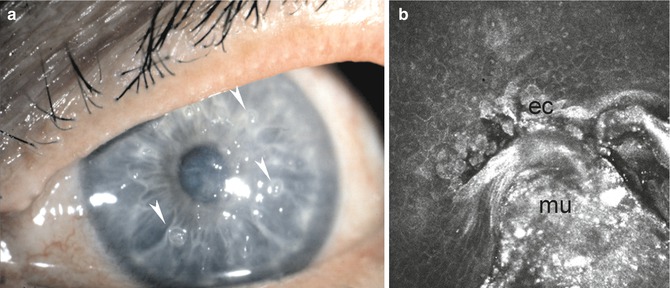
Fig. 3.7
Dry eye syndrome. Filiform keratitis demonstrating threads of desiccated corneal epithelial cells with associated mucus (arrowheads) in a severe dry eye syndrome (a). The epithelial cells (ec) and mucus (mu) as imaged by in vivo confocal microscopy (b)
Histopathology
In dry eye syndrome, the conjunctiva is affected before the cornea. It shows loss of goblet cells, conjunctival stromal edema, loss of epithelial cell surface microplicae, increased epithelial cell desquamation, and progressive squamous metaplasia [61, 62]. The cornea is more resistant to ocular surface disease. In severe cases, however, ribbon-like threads of desiccated corneal epithelial cells with associated mucus may pile up on the epithelial surface (Fig. 3.7b) [63]. In severe dry eye syndrome, sterile corneal melting, ulceration, and spontaneous perforation can occur (Fig. 3.5).
3.4.3 Immunologic Disease
3.4.3.1 Atopic/Vernal Keratoconjunctivitis
Definition
Corneal pathology caused by severe ocular allergy in patients with atopy.
Epidemiology
In a retrospective study of 45 patients with atopic keratoconjunctivitis, 34 patients had keratopathy and 21 had persistent corneal epithelial defects causing severe visual impairment (Fig. 3.8a) [64].
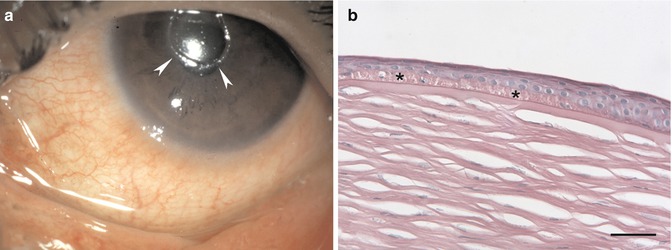
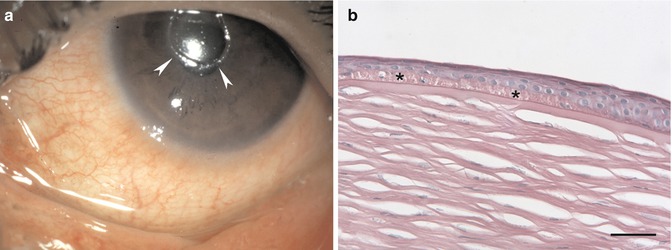
Fig. 3.8
Atopic keratoconjunctivitis. Corneal ulceration (arrowheads) in a patient with atopic keratoconjunctivitis (a). Linear deposition of subepithelial eosinophil granular substance in a corneal button (asterisks). The material was identified as major basic protein and eosinophil granule protein by immunofluorescence (b). Haematoxylin-eosin, bar = 50 μm
Histopathology
Toxic granule proteins of eosinophils such as major basic protein (MBP) and eosinophil cationic protein (ECP) inhibit epithelial cell migration and protein synthesis. These factors are typically involved in corneal ulceration in atopy. A linear subepithelial deposition of eosinophil granular substance can be detected in corneal buttons removed at transplantation in patients with atopic keratoconjunctivitis, associated with infiltration of eosinophils into the corneal stroma (Fig. 3.8b). Immunofluorescence staining is helpful in identifying this material as MBP and ECP [65].
Prognosis
Penetrating keratoplasty may be required in these cases, but a high rate of complications and graft rejections may occur [66].
3.4.3.2 Peripheral Ulcerative Keratitis
Staphylococcus-Associated Blepharokeratoconjunctivitis
Definition
Marginal keratitis, leading to corneal ulceration, is frequently observed in association with staphylococcus-associated blepharokeratoconjunctivitis. It is thought to be a hypersensitivity reaction to staphylococcal antigen.
Histopathology
Microscopic examination shows necrosis of the involved cornea and infiltration by neutrophils. Healing occurs by fibroblastic proliferation and may be associated with neovascularisation from the limbus [56].
Peripheral Ulcerative Keratitis Associated with Collagen Vascular Disease
Definition
Corneal inflammation and ulceration associated with acquired connective tissue and vasculitic disorders, including rheumatoid arthritis, psoriatic arthritis, systemic lupus erythematosus, polyarteritis nodosa, Wegener’s granulomatosis, and relapsing polychondritis. Ocular inflammation may portend catastrophic extraocular vasculitis.
Etiology
Pathophysiologic factors involved include immune complex deposition in the limbal area with activation of the complement cascade, release of inflammatory mediators, and attraction of neutrophils with resultant tissue destruction and corneal stromal melting [67].
Histopathology
Necrotising occlusive vasculitis is observed within the peripheral cornea and perilimbal lesions in patients with Wegener’s granulomatosis and polyarteritis nodosa [68, 69]. On microscopic examination, necrosis of the corneal epithelium and peripheral stroma is present with ulceration and acute inflammatory cell infiltrate. Granulomatous inflammation may be observed. The adjacent sclera is generally involved. A thinned vascularised stromal scar typically remains after healing.
Mooren’s Ulcer
Definition
Mooren’s ulcer is an idiopathic peripheral ulcerative keratitis, occurring without any identifiable systemic disorder.
Etiology
The exact pathophysiology remains uncertain, but the evidence suggests that it is an autoimmune process combining cell-mediated immunity against corneal antigens with humoral components.
Clinical Features
Mooren’s ulcer begins as a painful, yellow-grey infiltrate adjacent to the limbus and spreads circumferentially and centrally. Characteristically, it has an overhanging edge centrally. The sclera is not involved. After healing, the damaged cornea peripheral to the active, advancing front remains thinned, scarred, and vascularised leaving the patient with poor vision (Fig. 3.9a).
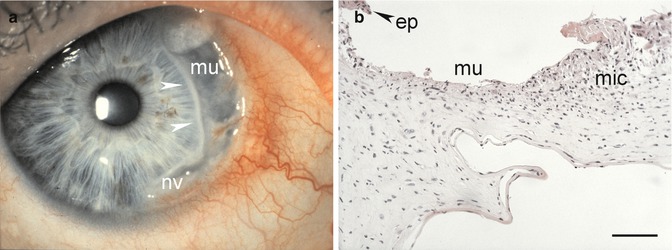
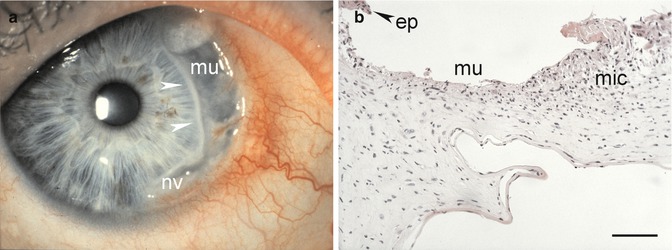
Fig. 3.9
Mooren’s ulcer. A typical Mooren’s ulcer (mu) with an overhanging central edge (arrowheads) and neovascular vessels (nv) from the limbal conjunctiva (a). Loss of epithelium (ep) within the ulcer (mu) and mixed inflammatory cells (mic) within the stroma (b). Haematoxylin-eosin, bar = 100 μm
Histopathology
On histology, lymphocytes, plasma cells, neutrophils, mast cells, and eosinophils are present in the area of ulceration. High levels of proteases and collagenases have been detected with destruction of the collagen matrix. Reactive keratocytes and disorganisation of collagen lamellae are present in midstroma (Fig. 3.9b).
3.4.3.3 Interstitial Keratitis
Syphilitic Stromal Keratitis
Definition
Syphilitic interstitial stromal keratitis results from congenital luetic infection. It is a rare disorder in developed countries. The course of the disease suggests an immune reaction toward Treponema pallidum.
Histopathology
Only a few histological studies of active disease are available. The entire cornea is edematous, inflamed, and necrotic with lymphocyte infiltration and early neovascularisation in the middle and deep stroma [56]. Necrosis of Descemet’s membrane and corneal endothelium may occur. Healing leaves a vascularised scar often with non-perfused “ghost vessels”. In chronic interstitial keratitis, the regenerating endothelium can produce focal or diffuse multilaminar thickening of Descemet’s membrane. These retrocorneal scrolls contain type I, III, IV, VI, and VII collagens as well as proteoglycans and are lined with attenuated corneal endothelium.
Cogan’s Syndrome
Definition
Cogan’s syndrome is a non-syphilitic interstitial keratitis typically associated with vestibuloauditory symptoms in young adults. An immune reaction against a common antigen of the cornea and inner ear is suggested.
Clinical Features
The mostly bilateral disease starts with diffuse or sectoral subepithelial infiltrates and progresses to classic interstitial keratitis. Episcleritis, scleritis, and uveitis can be associated with it. The end stage is a vascularised, scarred cornea with irregular astigmatism. Life-threatening aortic insufficiency and serious systemic necrotising vasculitis can complicate the course.
3.4.4 Infection
An intact corneal epithelium and a healthy tear film serve as an effective barrier to most bacterial, viral, mycotic, and parasitic infections. Interruption of this barrier, e.g. by contact lens wear, injury, or rupture of epithelial bullae, allows entrance and spread of microorganisms. Sequelae from microbial keratitis are a leading cause of corneal blindness word wide.
3.4.4.1 Bacterial Keratitis
Etiology
Bacterial keratitis can result from infection by virtually any virulent pyogenic organism (Fig. 3.10a), but the most frequent microbial species encountered in developed countries are Staphylococcus, Pseudomonas, other Gram-negative species, Streptococcus, and Corynebacterium [72, 73]. Primary tuberculous keratitis by Mycobacterium tuberculosis is now extremely rare. Non-tuberculous Mycobacteria, including Mycobacterium fortuitum, M. chelonae, M. gordonae, and M. avium-intracellulare, are all capable of causing an indolent, intractable keratitis. They are observed with increasing incidence after foreign-body injury or following office surgical procedures such as laser in situ keratomileusis (LASIK) [74]. Mycobacterium leprae is the causative agent of leprous keratitis.
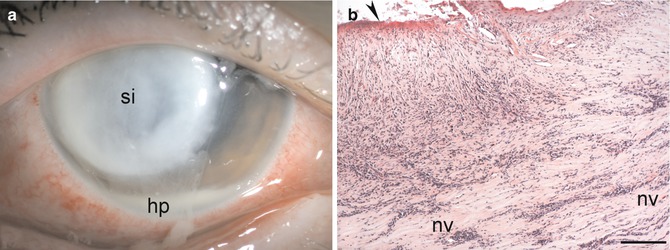
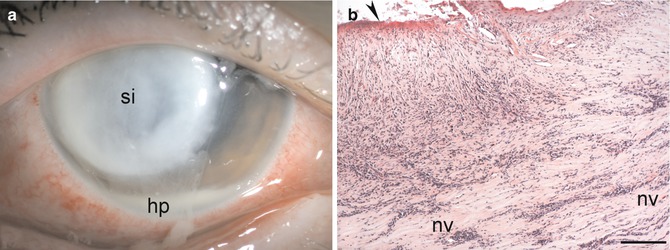
Fig. 3.10
Bacterial keratitis. Severe bacterial keratitis caused by Pseudomonas aeruginosa with a large whitish corneal stromal infiltrate (si) and hypopyon (hp) at the bottom of the anterior chamber (a). Histopathology of a corneal button after bacterial keratitis demonstrates loss of epithelium (arrowhead), massive invasion by chronic inflammatory cells, and neovascular vessels (nv) within the corneal stroma (b). Haematoxylin-eosin, bar = 100 μm
Histopathology
On histology, bacterial keratitis can be divided into distinct stages of progressive infiltration, active ulceration, regression, and healing (Fig. 3.10b). After adherence and entry of the organism, bacterial and neutrophil enzymes facilitate progressive bacterial invasion into the cornea. Tissue necrosis with subsequent sloughing of the epithelium and stroma takes place. Progressive keratolysis, descemetocele formation, and spontaneous perforation may follow. Gram stain will confirm microbial invasion. Giemsa stain is most frequently used to determine the type of infection present. Special stains include carbolfuchsin or Ziehl-Neelsen acid-fast stain for identification of suspected Mycobacterium, Actinomyces, or Nocardia. Mycobacteria may invade along peripheral corneal nerves and cause a non-necrotising, granulomatous inflammation with histiocytes and giant cells containing large numbers of acid-fast-staining lepra bacilli [75, 76].
3.4.4.2 Viral Keratitis
Adenoviral Keratoconjunctivitis IncludingEpidemic Keratoconjunctivitis
Definition
Adenovirus serotypes 8 and 19 are primarily responsible for epidemic keratoconjunctivitis (EKC).
Clinical Features
A flu-like illness may be associated. Following an acute follicular conjunctivitis, epithelial keratitis will develop in 80 % of patients, associated with marked discomfort, photophobia, epiphora, and blepharospasm. The keratitis evolves through four stages: stage 1, a diffuse, fine superficial epithelial punctate keratitis caused by live virus; stage 2, coalescence of the lesions to focal punctate, slightly raised whitish epithelial ones that last for approximately 10 days; stage 3, combined epithelial and subepithelial lesions by week 2 when all replicating virus has been removed by the host immune response; and stage 4, subepithelial nummular opacities [77, 78]. The subepithelial nebulae may persist for several months, causing glare and diminished vision before gradually resolving (Fig. 3.11a).
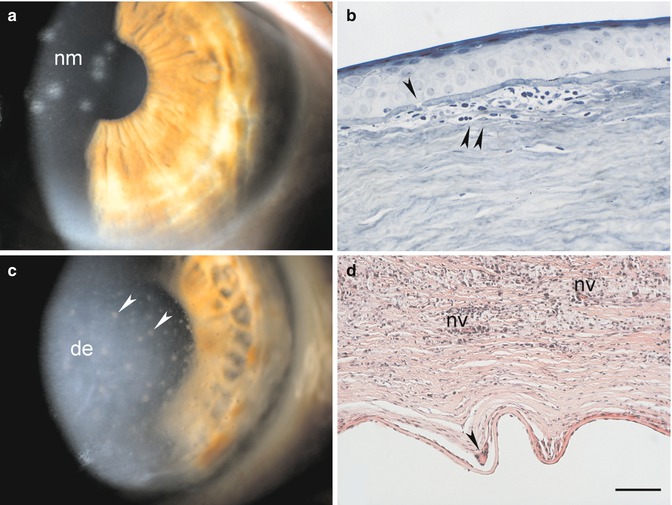
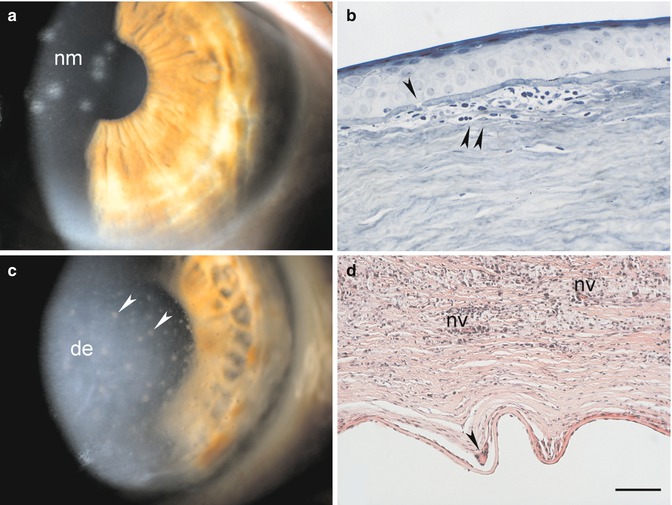
Fig. 3.11
Viral keratitis. Subepithelial stromal infiltrates (nummuli; nm) after epidemic keratoconjunctivitis (a). Specimen of a patient with nummuli 2 years after epidemic keratoconjunctivitis. Breaks in Bowman’s layer (arrowhead) with subepithelial accumulations of lymphocytes, histiocytes, and fibroblasts (double arrowhead) are present (b). An eye with Herpes simplex keratitis shows viral endotheliitis with endothelial precipitates (arrowheads) and disciform edema (de) of the corneal stroma (c). Stromal infiltration with granulomatous reaction to Descemet’s membrane (arrowhead), neovascular vessels (nv), and chronic inflammatory cells (d). Alcian blue, bar = 50 μm (b), and haematoxylin-eosin, bar = 100 μm (d)
Histopathology
Histopathological studies of adenoviral keratoconjunctivitis are rare. Adenovirus-like particles have been demonstrated within corneal epithelial cells of a patient with EKC caused by adenovirus serotype 8 [77]. Replica imprints of corneal epithelium in acute EKC show diffuse mild epithelial edema with scattered swollen and deformed cells that may be fused to small syncytial formations with pseudopodia-like processes. Intranuclear vacuolar inclusions and dense bodies with replicating virus are observed [79]. A corneal specimen taken from a patient who underwent lamellar keratoplasty for permanent visual loss due to EKC 2 years earlier revealed lymphocytes, histiocytes, and fibroblasts in the deep epithelial layers and anterior stroma. Breaks in Bowman’s layer were present (Fig. 3.11b) [80]. Virus could not be recovered by culture or visualised by electron microscopy, lending support to the theory that nummular infiltrates result from host immune and inflammatory responses to residual viral antigen.
Herpes Simplex Viral Keratitis
Definition
Herpes simplex virus (HSV), the most ubiquitous communicable infectious virus in humans, causes a vast variety of chronically recurring ocular disease.
Epidemiology
Herpes simplex virus keratitis is the leading infectious cause of corneal blindness in developed countries [81].
Clinical Features
Primary ocular involvement may present as an acute follicular keratoconjunctivitis with unspecific keratitis and associated vesicular periocular skin involvement. Recurrent corneal herpes may manifest in the epithelium as infectious epithelial keratitis (dendritic keratitis, geographic ulcers) or as a trophic (metaherpetic) keratopathy. Stromal disease may be divided into categories according to the currently accepted pathogenetic mechanisms: viral interstitial keratitis, immune rings, and limbal vasculitis (antigen-antibody-complement-mediated immune disease) and disciform edema/endotheliitis (delayed hypersensitivity immune disease; Fig. 3.11c).
Histopathology
Histologically, the dendrites correspond to epithelial cell loss. In the vicinity of the dendrite, rounded epithelial cells and variably sized syncytia containing nuclei with bizarre shapes are present. Pseudopodia-like processes containing viral DNA and some RNA extend from the syncytia into the surrounding epithelial cells, which on coming into contact with these processes become rounded and liquefied, and give rise to another syncytium. The infected epithelial cells show intranuclear and cytoplasmic inclusion bodies and polykaryocyte formation [82]. Epithelial giant cells may be present adjacent to the sites of epithelial cell loss.
In stromal disease, edema, migration of inflammatory cells from the limbus, and subsequent necrosis occur. Whereas neutrophils and macrophages are the predominant cell types in early stages, T-lymphocytes dominate later on. Animal experiments show that HSV-1-induced corneal tissue destruction is mainly mediated by mononuclear cells and neutrophils and that these cells are probably attracted into the cornea by cytokines secreted by activated CD4+, Vβ8+ T-cells [83]. Blood vessels invade the stroma only in case of necrosis. Healing is slow and involves deposition of irregular collagen bundles. Residual inflammation can be observed histopathologically long after the acute infection. Inflammation was present in the majority of corneal buttons removed at penetrating keratoplasty after HSV disease, despite being clinically inactive for more than 6 months prior to surgery. A large amount of HSV genome was detected in 86 % of quiescent corneas with a history of herpetic keratitis. However, HSV DNA in low quantities was also present in 11 % of control corneas and was also detected in corneal buttons of clinically unsuspected cases [84, 85].
Varicella Zoster Virus Keratitis
Definition
Varicella zoster virus (VZV) may involve any structure of the anterior segment of the eye.
Epidemiology
Whereas chickenpox is the primary disease, herpes zoster ophthalmicus (HZO) is a recurrent infection caused by the VZV. Of every 1,000 people surviving to the age of 85 years, 500 will have one attack of dermatomal herpes zoster, and 10 will have had two attacks. Age and impaired immunity greatly enhance the risk for development of herpes zoster [88, 89]. HZO is second only to thoracic zoster in frequency [90].
Clinical Features
In chickenpox, the cornea may develop infectious superficial punctate keratitis or branching, dendritic ulcers without terminal knobs. These lesions are positive for VZV DNA [91]. Disciform keratitis similar to that caused by herpes simplex virus may develop.
Two-thirds of HZO patients have corneal involvement. Punctate keratitis or pseudodendrites, anterior stromal infiltrates, sclerokeratitis, keratouveitis-endotheliitis, peripheral ulcerative keratitis, delayed mucous plaques, disciform keratitis, neurotrophic keratitis, and exposure keratitis can occur [92]. VZV can typically be isolated from dendritic epithelial lesions and ulcerations [91, 93]. However, VZV DNA shedding is highly variable, age-dependent, and probably related to the host’s immune status [94]. Immune keratitis similar in appearance to HSV stromal disciform edema/endotheliitis may occur. If there is an associated interstitial keratitis, there is an increased chance of deep neovascularisation with lipid deposition and fibrovascular scarring. Assays for VZV DNA have been positive in some cases [87, 95–97]. Sixty percent of HZO patients develop moderate to complete corneal anaesthesia secondary to destructive VZV ganglionitis and to aqueous tear deficiency [92, 98]. Neurotrophic keratopathy may progress to ulceration and perforation.
Histopathology
Histopathological reports of acute and chronic HZO reveal normal corneas or corneal inflammation with macrophages at the level of the endothelium associated with non-granulomatous inflammation, consisting mainly of lymphocytes and plasma cells, in the uveal tissue [96, 99, 100]. Chronic HZO keratitis may present with a giant cell reaction at the level of Descemet’s membrane [87]. VZV DNA may be detected in human corneas at least 8 years after the acute event [96, 100]. Viral DNA was mainly found in mononuclear cells with eosinophilic intracytoplasmic inclusions within vascular stromal scars, in keratocytes, and in epithelial cells [96].
3.4.4.3 Fungal Keratitis
Definition
Fungal keratitis is one of the most challenging types of microbial keratitis to diagnose and treat.
Epidemiology
The incidence of fungal keratitis in temperate climates is low compared to bacterial infection, but tends to be more commonly seen in rural areas and warm climates.
Etiology
Candida, Aspergillus, and Fusarium species are the most frequently isolated fungi in fungal keratitis. Fungi gain access into the corneal stroma through an epithelial defect caused by injury, contact lens wear, an otherwise compromised surface, or following surgery.
Clinical Features
Clinically, the following features are suggestive of fungal infection: stromal infiltrate with feathery edges, grey and elevated infiltrates, satellite lesions, presumed immune rings, and endothelial plaques (Fig. 3.12a). Corneal scrapings are typically performed to culture fungi from the cornea, but due to the location of the pathogen deep in the stroma, a corneal biopsy submitted to microbiological and histopathological analysis will often yield better results. Fungi typically cause inflammation and necrosis with penetration deep to the corneal stroma and may perforate through an intact Descemet’s membrane. Organisms that have penetrated into the anterior chamber, iris, or lens, or have infiltrated the sclera, are extremely difficult to control. Keratoplasty is sometimes necessary in acute disease when medical treatment fails, and infection may progress to deep ulceration and spontaneous perforation [101].
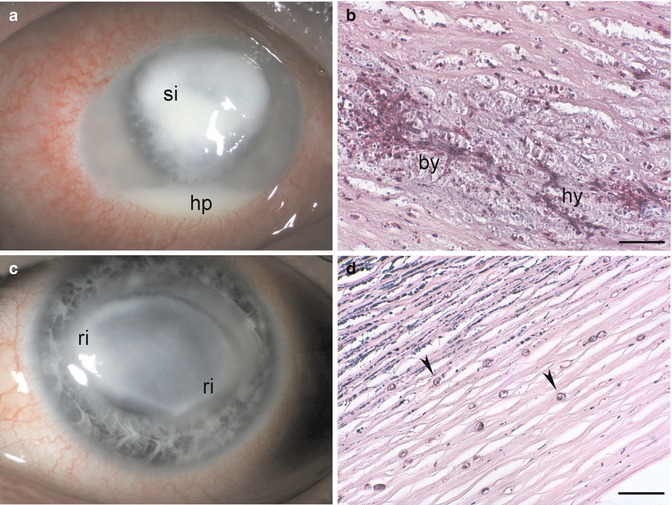
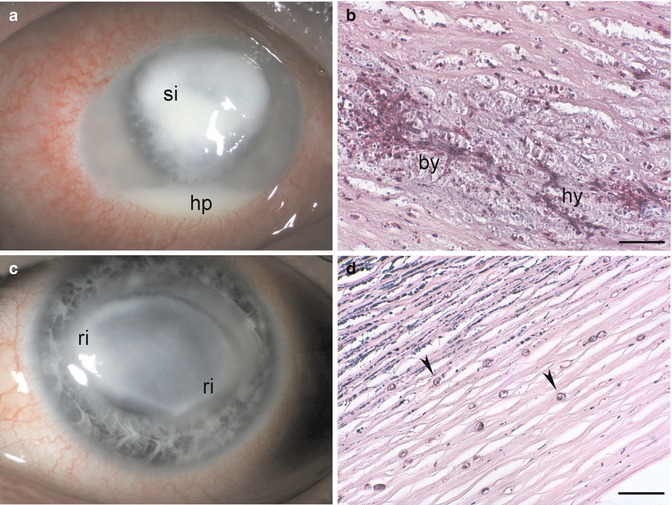
Fig. 3.12
Fungal and parasitic keratitis. Keratitis from Aspergillus species. Deep stromal infiltrate (si) with feathery edges and a hypopyon (hp) at the bottom of the anterior chamber (a). Fungal keratitis caused by Candida species. Necrotising stromal inflammation with infiltration by chronic inflammatory cells, branching hyphae (hy), pseudohyphae, and budding yeasts (by) are visible (b). Acanthamoeba keratitis with a typical ring infiltrate (ri) in a contact lens wearer (c). Chronic inflammation and multiple Acanthamoeba cysts (arrowheads) within the stroma (d). Periodic acid-Schiff, bars = 50 μm
Histopathology
Intact organisms are rarely observed within active necrosis, but they can be found in the surrounding stroma, even in culture-negative specimens. Gram stain identifies yeast, and Giemsa stain is useful in detecting fungal elements. However, if fluorescein microscopy is available, acridine orange and calcofluor white are the stains of choice. Fungal hyphae oriented perpendicular to corneal lamellae and penetration of an intact Descemet’s membrane are suggestive of progressive pathogenicity (Fig. 3.12b) [101, 102].
3.4.4.4 Parasitic Keratitis
Acanthamoeba
Definition
Acanthamoeba are small, free-living ubiquitous protozoa that exist in two life forms, an active trophozoite and a dormant cyst. Encystment is the major factor accounting for the severity of Acanthamoeba infections. The first published reports of Acanthamoeba keratitis appeared in the 1970s; it remains unclear whether earlier cases had gone undiagnosed [103]. In a retrospective study of host corneal tissue after therapeutic keratoplasty from 1955 to 1970, no case of Acanthamoeba was identified, suggesting that this may be a new infectious entity [104].
Epidemiology
Clinical Features
Clinically, severe pain is a typical feature. Acanthamoeba keratitis can present as a superficial epithelial disease – often confused with HSV keratitis – anterior stromal infiltrates, disciform keratitis, keratoneuritis, and ring infiltrate. Anterior chamber reaction is rare (Fig. 3.12c).
Histopathology
Acanthamoeba cysts can remain in corneal tissue for an extended period of time following keratitis (in one study up to 31 months even following anti-amoebic treatment) and they may cause persistent corneal and scleral inflammation in the absence of active amoebic infection [106]. Gram, Giemsa, and haematoxylin-eosin stains do not stain Acanthamoeba, making detection of this organism difficult. Histopathological studies have demonstrated both trophozoites and cysts (Fig. 3.12d) using methenamine-silver, periodic acid-Schiff, Masson’s trichrome, and iron-haematoxylin-eosin stains [107, 108]. In addition, trophozoite and cyst forms in paraffin-embedded corneal tissue sections can be rapidly and differentially stained with calcofluor white. Under the fluorescence microscope, the trophozoites are bright red-orange and cyst cell walls fluoresce bright apple-green with red-orange cytoplasm. Retrospective identification is possible by destaining haematoxylin-eosin-stained sections. Digesting corneal tissue with trypsin or collagenase and hyaluronidase solutions helps to more readily identify trophozoites [107–109].
Histopathological findings in corneal specimens with intractable Acanthamoeba keratitis include epithelial denudation and variable degrees of necrosis, inflammation, and cysts or trophozoites of Acanthamoeba within the stroma. No neovascular vessels were found in the stroma despite long-standing corneal inflammation [110]. A granulomatous reaction with many multinucleated giant cells, some with engulfed cysts of Acanthamoeba, can be present in the posterior corneal stroma and anterior chamber along Descemet’s membrane [111].
Corneal Microsporidiosis
Definition
Microsporidia are ubiquitous, obligate intracellular spore-forming protozoan parasites.
Etiology
Clinical Features
Most patients present with a punctate epithelial keratoconjunctivitis with small foci of anterior stromal infiltrations [114]. Hyphaema and necrotising keratitis have been reported.
Histopathology
Diagnosis requires demonstration of oval Microsporidia spores in specimens obtained by superficial corneal scraping or corneal and conjunctival biopsy. Spores stain Gram-positive and may show a periodic acid-Schiff positive body at one end of an oval spore. Staining is variable with routine methods such as Giemsa, Gomori silver, or Ziehl-Neelsen acid-fast staining. Calcofluor white and modified trichrome stains are preferred. Electron microscopy readily reveals the characteristic coiled tubules within the spore coat of Microsporidia [113, 115].
3.5 Injuries and Surgery
3.5.1 Chemical and Thermal Injury
3.5.1.1 Chemical Injury
Definition
Chemical injury to the ocular surface can be acidic, alkaline, or toxic.
Histogenesis
In alkali injury, hydroxyl ions rapidly advance through ocular tissues causing saponification of cellular membranes with massive cell death and extensive hydrolysis of the corneal extracellular matrix. Acidic compounds precipitate proteins within the ocular surface epithelium, thus acting as a partial barrier to further penetration. However, strong acids can overcome these precipitated proteins and progress through tissue much as alkali. Toxic agents include a wide variety of chemicals that are destructive to biological tissue, but are not particularly acidic or alkaline.
Epidemiology
Industrial accidents are far more common than domestic accidents despite mandatory preventative measures, especially in developing countries. The majority of chemical trauma affects males and is mild [116].
Clinical Features
Depending on the strength of the chemical, epithelial defects, corneal stromal opacity with melting and secondary infection, and perilimbal ischemia, necrosis or both with subsequent limbal stem cell insufficiency can occur (Fig. 3.13a). Penetration of the chemical into the anterior chamber may result in secondary glaucoma through prostaglandin release or scarring of the outflow channels, hypotony through necrosis of the ciliary body, mydriasis, cataract, and even phthisis bulbi.
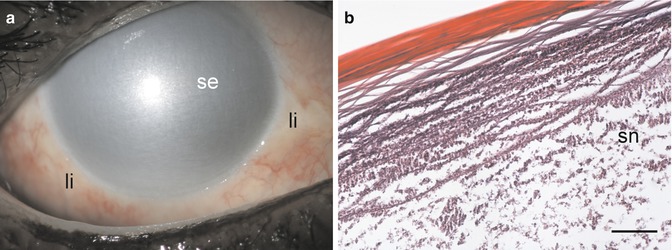
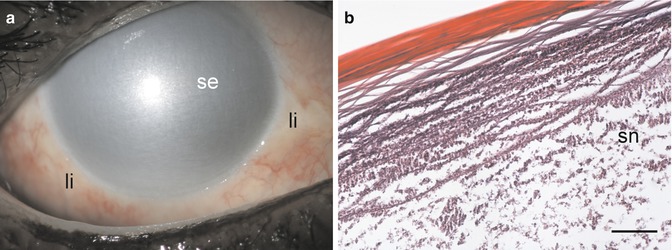
Fig. 3.13
Chemical injury. Severe alkali burn with limbal ischemia (li), complete loss of corneal epithelium, and severe stromal edema (se) of the cornea (a). Histopathology 12 weeks after severe alkali burn. Loss of epithelium, stromal inflammation, and stromal necrosis (sn) that is nearly complete (b). Haematoxylin-eosin, bar = 100 μm
Typically, a severe fibrinous inflammatory reaction develops in the anterior segment of the eye that is not necessarily time limited. Externally, the inflammation can lead to major conjunctival scarring with symblepharon formation.
Histopathology
Concentrated alkali strips the cornea of vital cells and glycosaminoglycans, leaving behind acellular, denatured collagen framework (Fig. 3.13b). Inflammatory mediators are released that are chemotactic to neutrophils [117], which release leukotrienes and cause further tissue damage through a respiratory burst. Interleukin (IL-1 alpha and IL-6) levels are markedly elevated in the regenerating corneal epithelium during the early stages of an alkali burn and may play an important role in associated corneal damage and repair [118]. Collagenase production by the epithelium, leucocytes, and fibroblasts results in corneal ulceration [119]. Fibroblasts that invade the cornea after severe alkali burn to promote healing are immature. Ascorbate deficiency following chemical injury further restricts the stromal repair process.
Prognosis
Factors influencing final outcome are (1) corneal and conjunctival surface injury, repair, and differentiation, (2) corneal stromal matrix injury, repair and ulceration, and (3) corneal inflammation [120]. Therapy depends on prompt irrigation and removal of the chemical, promoting re-epithelialisation, preventing corneal ulceration, and controlling inflammation. Even without limbal ischemia, secretion of new basement membrane and epithelial readhesion may be delayed [121]. Early surgeries may include removal of necrotic tissue, amniotic membrane transplantation, and tenoplasty. In complete limbal stem cell insufficiency, limbal autograft or allograft transplantation or limbokeratoplasty is performed later [120].
3.5.1.2 Thermal Injury
Definition
Thermal injury usually is less severe than chemical assaults to the cornea, possibly because the contact with the heat is generally of lesser duration.
Clinical Features
Thermal injury will coagulate corneal epithelium, which becomes opaque and may slough off. The stroma beneath usually remains clear in the acute phase.
A special instance is thermal damage to the corneoscleral wound during phacoemulsification (“phakoburn”) that may result in difficulty with wound closure and lead to wound leakage, damage to the adjacent corneal stroma and endothelium, fistula formation, and high degrees of postoperative astigmatism [122].
Histopathology
The primary effect of thermal energy on the corneal stromal layer is on the collagen matrix. A reversible contraction, seen clinically as corneal striae, is followed by irreversible collagen damage, including lysis of the interhelix covalent bonds and triple helix destruction of the collagen fibrils [122].
3.5.1.3 Gas Injuries
Exposure to tear gas or other gaseous irritants may cause epithelial defects, which heal without sequelae. High concentrations of gaseous substances may cause corneal necrosis.
Etiology
Sulphur mustard gas injuries, mostly suffered during chemical warfare, have been studied extensively. Sulphur mustard is a vesicant agent with severe irritating effects on many tissues.
Clinical Features
Ocular involvement is seen in 75–90 % of individuals exposed to sulphur mustard. Most cases resolve uneventfully; however, a minority of exposed patients will have either a persistent smouldering inflammation (chronic form) or late-onset corneal lesions appearing many years after a variable “silent” period (delayed form) [123]. Corneal signs after mustard gas injury include corneal scars or opacities, limbal stem cell insufficiency, epithelial defects and irregularities, corneal neovascularisation, and secondary degenerative changes, including lipid and amyloid deposition [124].
Histopathology
Excised corneal buttons after keratoplasty for sulphur mustard injury (Fig. 3.14) disclose absence or irregular epithelium, thickened epithelial basement membrane, destroyed Bowman’s layer, fibrovascular pannus, stromal necrosis with chronic inflammation, stromal scarring with keratocyte loss, amyloid deposition, and neovascularisation [124–126].
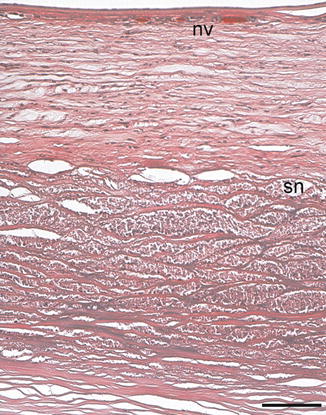
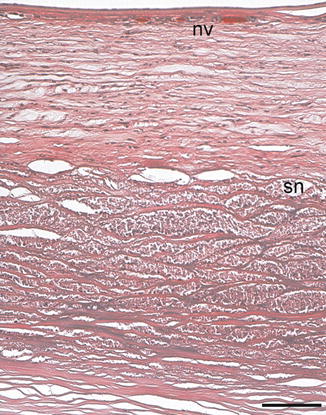
Fig. 3.14
Mustard gas injury. Absence of corneal epithelium, thickened epithelial basement membrane, destroyed Bowman’s layer, fibrovascular pannus, superficial neovascular vessels (nv), and massive stromal necrosis (sn) 13 years after exposure to sulphur mustard (Courtesy of Dr. Miriam Richter, Charité-Campus Benjamin Franklin). Haematoxylin-eosin, bar = 100 μm
3.5.2 Physical Injury
Physical injury to the cornea can have potentially devastating effects on the integrity of the globe and on vision. Blunt trauma including corneal abrasion and corneal foreign body must be discriminated from sharp injuries, i.e. penetrating or perforating corneal injury.
3.5.2.1 Blunt Trauma
Corneal Abrasion Including Recurrent Erosion Syndrome
Definition
Traumatic partial or complete loss of the corneal epithelium is one of the most common ocular injuries.
Etiology
Tangential impact from foreign bodies, including fingernail, paper, plants, or brushes, is the most common cause of corneal abrasion. Recurrent erosion syndrome usually follows a scraping caused by organic material, such as paper or fingernail.
Histogenesis
Full-thickness corneal epithelial defects rapidly heal from the periphery toward the centre via a combination of migration of polygonal cells and proliferation of newly formed basal cells. Keratocyte apoptosis beneath an epithelial debridement wound is thought to be an initiating event in wound healing [127].
Clinical Features
Although patients with traumatic corneal erosions are less likely to have recurrent disease than patients with basement membrane dystrophy, up to 46 % of patients may be symptomatic 4 years following injury [128]. Recurrent erosion syndrome is characterised by repeated episodes of sudden onset of pain usually at night or upon awakening, accompanied by redness, photophobia, and epiphora. These symptoms are related to corneal de-epithelialisation in an area in which the previously injured epithelium is weakly adherent [129].
Histopathology
When scraped loosened sheets of corneal epithelium from corneal epithelial wounds in patients with posttraumatic recurrent erosion syndrome are examined, a defect in collagen fibrils that anchor the epithelial basement membrane to Bowman’s layer is detected. Hemidesmosomes do not seem to be impaired [130]. Recurrences were associated with upregulation of matrix metalloproteinase-2 and -9 in the tear fluid [131].
Prognosis
In the majority of cases, management of the acute episode by patching, cycloplegics and topical antibiotic ointment with prophylactic application of gels during daytime and ointment at night prevents further erosion. In a minority of patients these measures prove insufficient and they may need alternative treatment modalities including doxycycline and corticosteroids, therapeutic contact lens wear, anterior stromal puncture, superficial keratectomy, and, most effectively, excimer laser therapy (phototherapeutic keratectomy) [129, 132].
Severe Blunt Trauma
Severe corneal contusion insufficient to rupture the globe may result in a transient ring-shaped corneal edema [133] and rupture of Descemet’s membrane.
Etiology
Obstetric forceps and vacuum extraction injuries are classic examples of severe blunt trauma with Descemet’s rupture. Complete corneal rupture is uncommon following blunt trauma, unless predisposing factors such as keratoconus, pellucid marginal degeneration, Terrien’s marginal degeneration, or prior corneal surgery are present [134, 135]. The rupture may occur at the point of application of the pressure but, more frequently, is of the countercoup type.
Histopathology
Histology of ring-shaped corneal edema after blunt trauma may demonstrate focal, deep stromal lamellar disruption and endothelial cell damage surrounding the injury site [136]. Especially in young individuals who have a thin Descemet’s membrane, its rupture may lead to acute corneal swelling and cellular infiltration. However, healthy endothelium is able to heal such ruptures by migrating over the area of retracted Descemet’s membrane, usually over 3 months. Recurrences of the edema may occur even years later in the area of injured endothelium [137].
After obstetric forceps injury, histopathology shows (1) large tears of Descemet’s membrane with a fragment of Descemet’s membrane extending into the anterior chamber at one end of the tear and scroll formation at the other end, (2) scrolls of Descemet’s membrane at each margin of the original break, (3) small breaks in Descemet’s membrane and healing by fibrosis, and (4) a small break in Descemet’s membrane with minimal fibrosis (Fig. 3.15a). Scanning electron microscopy reveals folds in Descemet’s membrane and attenuation or absence of endothelium. Spindle- and stellate-shaped cells and pigment granules are present in the area of the tear in most cases [138]. In addition, epithelial-like metaplasia of endothelial cells in the area of rupture may be noted [139].
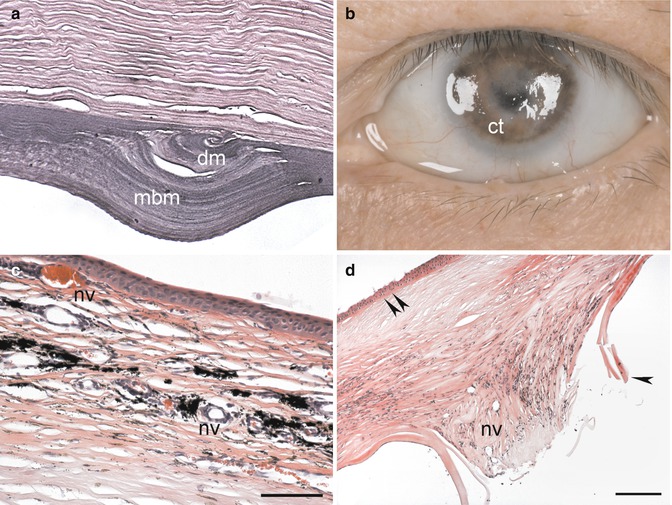
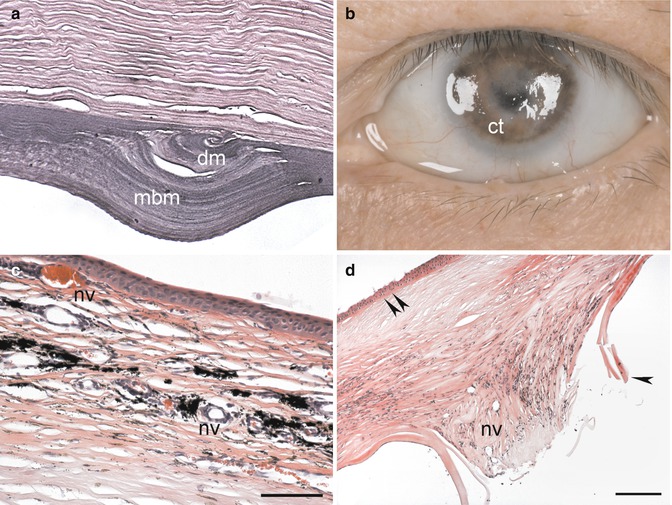
Fig. 3.15
Physical corneal injury. Blunt injury from obstetrical forceps delivery. Corneal specimen of 51-year-old patient with coiled Descemet’s membrane (dm) within a thick deposit of multilaminar basement membrane (mbm) with only few attenuated endothelial cells (a; Courtesy of Dr. Irene Pecorella, University of Rome). Corneal tattooing (ct) of a leucoma from perforating ocular injury (b). Corneal tattooing in a specimen with scar and neovascular vessels (nv) within the corneal stroma as evidenced by clumps of brown-black granules within the anterior corneal stroma within the cytoplasm of keratocytes and in the extracellular matrix (c). Severe perforating corneal injury with loss of Bowman’s layer (double arrowhead), irregular stroma, a break in Descemet’s membrane (arrowhead) with complete endothelial cell loss, and neovascular vessels (nv) in a posterior scar (d). Periodic acid-Schiff, bar = 50 μm (a), haematoxylin-eosin, bar = 100 μm (c), and bar = 200 μm (d)
3.5.2.2 Sharp Injury
Lacerations, punctures, surgical incisions, and foreign bodies can produce penetrating and perforating injuries of the cornea.
Penetrating Wounds
Penetrating wounds that do not perforate the cornea are often caused by foreign bodies. Superficial wounds may heal by proliferation of epithelium dipping down into the defect. Healing of deeper wounds will additionally involve keratocytes recruited from adjacent stroma.
Corneal Tattooing
Definition
Corneal tattooing to reduce glare following traumatic loss of iris and tattooing of corneal leucoma for cosmetic purposes are typical examples of surgically induced penetrating corneal wounds (Fig. 3.15b) [140]. A plethora of techniques and instruments to apply the dye to the anterior corneal stroma exist. India ink, metallic colours, organic dyes, and uveal pigment from animal eyes are used.
Histopathology
Keratocytes can actively ingest and retain tattooing particles of non-metallic dyes within their cell membrane for very long periods of time. Histologically, clumps of brown to black granules are present mainly in the midstroma (Fig. 3.15c). By electron microscopy, numerous round and oval electron-dense particles are seen in the cytoplasm of keratocytes, arranged as clusters or patches. No granules are detected in the extracellular matrix [141].
Prognosis
Complications such as toxic reaction, iridocyclitis, persistent corneal epithelial defects, and corneal ulceration as well as granulomatous keratitis have been reported following corneal tattooing [142].
Perforating Corneal Wounds
Perforating corneal wounds that penetrate into the eye typically lead to corneal scar formation and endothelial cell loss, which correlates closely with wound length [143].
Lacerations
Lacerations are typically induced by sharp cutting objects. Whereas shelved (oblique) incisions tend to close spontaneously, vertical (perpendicular) lacerations open spontaneously and require suture repair. Typically, lacerations are associated with loss of the anterior chamber and incarceration or prolapse of the iris. Ciliary body and lens can also prolapse (Fig. 3.15d)
Puncture Wounds
Puncture wounds result from thorns or tiny sharp instruments. The wound itself is small and heals promptly. However, damage to the intraocular structures such as cataract after lens injury as well as endophthalmitis can complicate the course.
Surgical Wounds
Surgical wounds have a lot in common with corneal lacerations. They show clean, sharply incised edges. With the exception of keratoplasty, they are typically located at the corneoscleral limbus. Modern small-incision intraocular surgery and advanced suturing techniques have reduced the risk of surgical wound dehiscence.
Wound Healing After Corneal Perforating Injury
Histogenesis
Following perforating corneal injury, a fibrin plug forms at the posterior wound. Transforming growth factor beta-2 (TGFβ2) is released by the corneal epithelium into the stroma as soon as its basement membrane is destroyed [144]. Bowman’s layer has no capacity to regenerate. Corneal stromal edema develops during the first hour after injury. Thereafter, fibroblast repair tissue originating from corneal stromal keratocytes and histiocytes fills and seals the wound.
Histopathology
Even after many months of healing, collagen remodelling in corneal scar tissue is not complete. Collagen interweaving, lack of a lamellar structure, and enlarged, disordered fibrils are visible by electron microscopy [145, 146]. Descemet’s membrane curls after the initial injury, and endothelial cells from wound margins extend across the defect and lay down new basement membrane (Fig. 3.16a).
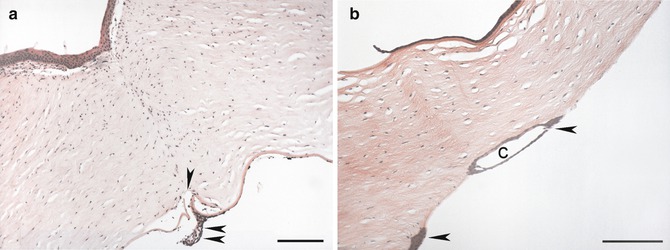
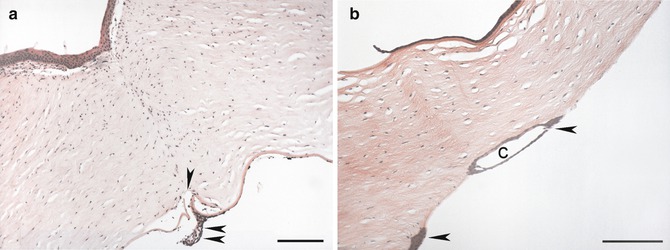
Fig. 3.16
Surgical corneal wounds. Penetrating corneal wound after perforating keratoplasty with deranged stromal lamellar structure, curling and duplication of Descemet’s membrane (arrowhead), endothelial cell loss, and adhesion of iris stroma (adherent leucoma; double arrowhead) to the posterior edge of the wound (a). Epithelial ingrowth after lamellar keratoplasty. Multilayered corneal epithelium (arrowheads) with cyst (c) formation is present on the posterior surface of the graft corresponding to the lamellar interface (b). Haematoxylin-eosin, bar = 100 μm (a) and bar = 200 μm (b)
Epithelial downgrowth
Definition
Epithelium may show ingrowth into a corneal wound in the beginning of the healing response, but it is normally displaced to the surface level with time. However, incarcerated epithelial islands or cysts may remain within the stroma. If the wound leaks, the epithelium may even grow through a full-thickness corneal wound into the anterior chamber to line the endothelial surface, extending to the anterior chamber angle and iris surface (epithelialisation of the anterior chamber). Occasionally, the ingrowth may form a cyst.
Clinical Features
Clinically, epithelial ingrowth can cause glaucoma, fistula formation, retrocorneal membranes, uveitis, iris cysts, bullous keratopathy, and corneal graft failure. It can present decades after initial injury [147]. Although rare with modern surgical techniques, the incidence of epithelial ingrowth has increased again after introduction of lamellar corneal surgical techniques.
Histopathology
Analysis of 207 consecutive cases of epithelial ingrowth showed that it was cystic in 40 cases and diffuse in 167. Interestingly, it was not suspected prior to histopathological examination in 36 % of cases. It was mainly caused by penetrating injury, cataract surgery, and penetrating keratoplasty. Histologically a multilayered surface epithelium was present on intraocular surfaces such as the cornea, iris, chamber angle, ciliary body, and lens capsule (Fig. 3.16b) [148].
3.5.3 Postsurgical Pathology
3.5.3.1 Corneal Collagen Cross-Linking
Definition
Histopathology
Analysis of human corneas following cross-linking demonstrates a significant increase in collagen fibre diameter in the anterior stroma. Keratocyte proliferation was increased 6 months following cross-linking as evidenced by increased Ki-67 immunopositivity [151]. A considerable keratocyte loss in the anterior and midstroma of the central and peripheral cornea (Fig. 3.16) was present even 30 months postoperatively [152]. Keratocyte differentiation into myofibroblasts does not seem to play a crucial role in the postoperative flattening of the cornea [151, 152] (Fig. 3.17a).
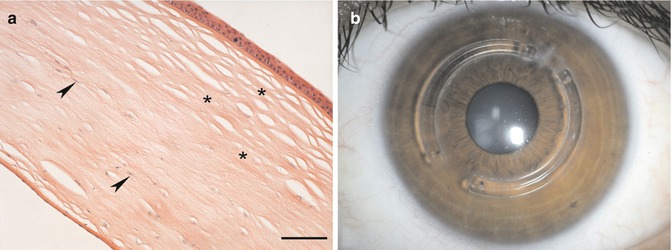
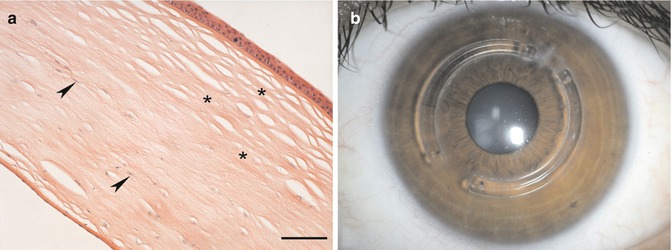
Fig. 3.17
Postsurgical corneal pathology. Histopathology of corneal collagen cross-linking for progressive keratoconus. Loss of keratocyes (arrowheads) from the anterior and middle layers (asterisks) of the corneal stroma (a). Intrastromal corneal ring segments implanted for progressive keratoconus (b). Haematoxylin-eosin, bar = 100 μm
3.5.3.2 Intrastromal Ring Implants
Definition
Intrastromal corneal ring segments are polymethyl methacrylate (PMMA) corneal implants for the correction of low to moderate myopia. They are also used for the treatment of corneal ectasia in keratoconus or after laser in situ keratomileusis (LASIK) to prevent or delay the need for a corneal transplant (Fig. 3.17b) [153].
Histopathology
In rabbit models, new collagen formation with lamellar organisation and increased keratocyte density are observed adjacent to the implant [154]. Weeks and months after implantation in humans, lamellar channel deposits consisting of intracellular lipids occur around the implants without alteration of optical performance [153]. Histopathology of a cornea following intrastromal ring segment implantation for corneal ectasia after LASIK showed peripheral focal thickened areas around the implant channel. Adjacent collagen lamellae were displaced. The stroma around the segment stained with periodic acid-Schiff stain. On electron microscopy, the stroma contained deposits of electron-dense granular material with interspersed empty spaces. Overlying the implants, a small area of epithelial hypoplasia was present [155].
Prognosis
Spontaneous extrusion of intrastromal ring segments has been reported. Rare complications necessitating removal of implants include keratitis, stromal necrosis, and corneal melting [156].
3.5.3.3 Radial Keratotomy (RK)
Definition
Incisional procedures to alter refraction by weakening corneal structure and by producing surface gaps to increase anterior corneal surface volume. Near full-thickness radial incisions known as radial keratotomy (RK) are used for correction of myopia (Fig. 3.18a). The greater accuracy and precision of photorefractive keratotomy (PRK) and laser in situ keratomileusis (LASIK) have diminished the role of RK in refractive surgery.
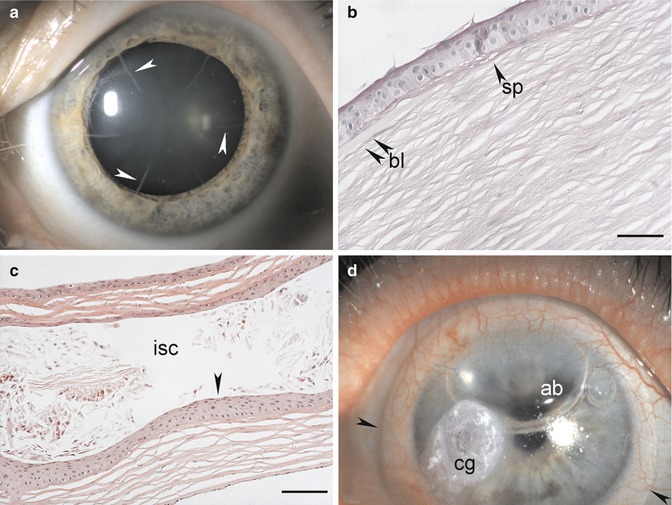
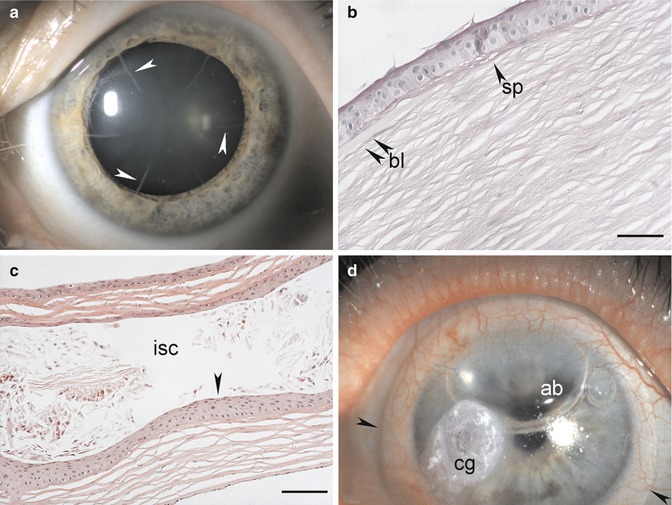
Fig. 3.18
Postsurgical corneal pathology. Radial keratotomy cuts (arrowheads) for progressive keratoconus (a). Histopathology after phototherapeutic keratectomy. Loss of Bowman’s layer (bl) within the ablated area that shows a nonvascularised subepithelial pannus (sp) instead (b). Histopathology following complicated laser in situ keratomileusis (LASIK). Epithelial ingrowth (arrowhead) into the flap interface with intrastromal cyst (isc) formation (c). Tissue adhesive (cg; cyanoacrylate glue) with a contact lens (between arrowheads) and an intraocular air bubble (ab) to seal a descemetocele (d). Periodic acid-Schiff, bar = 50 μm (b), and haematoxylin-eosin, bar = 100 μm (c)
Histopathology
Animal studies have shown that healing of RK incisions by wound fibrosis is accomplished by transformation of adjacent keratocytes to myofibroblasts, eventually leading to stromal wound contraction [157]. In humans, many incisions retain epithelial plugs. Underneath these plugs, the epithelial-stromal interface is characterised by three adjacent zones: a duplicated basement membrane complex, a zone that resembles Bowman’s layer, and a zone in which the collagenous fibres are oriented parallel to the plugs. Scar tissue orientation is transverse at the base of the plug and sagittal in deeper wound regions [158, 159].
3.5.3.4 Epikeratoplasty
Definition
Epikeratoplasty was originally performed for aphakia, keratoconus, and myopia. The procedure includes suturing a commercially cryolathed corneal-derived lenticule (epikeratophakos) with a specific dioptric power onto de-epithelialised central cornea. Epikeratoplasty is rarely performed today.
Histopathology
Human corneas after epikeratoplasty show thickened epithelium, bends and fractures in Bowman’s layer, interface epithelial ingrowth and cyst formation, absence of keratocytes and stromal abnormalities in the lenticule, and abnormal host keratocytes in the recipient lamellar bed [162–165]. The interfibrillar collagen distance of the lenticule was increased compared with that of the host cornea. The central thickness of the lenticule increased the total corneal thickness by 56 % [163].
3.5.3.5 Photoablative Refractive Surgery with Excimer Laser
Phototherapeutic and Photorefractive Keratectomy
Definition
The excimer laser, which uses ultraviolet radiation (193 nm), is applicable to both phototherapeutic (PTK) and photorefractive (PRK) keratectomy.
Histopathology
In both procedures, the corneal epithelial cells with their basement membrane, Bowman’s layer and the anterior stroma are disrupted. Re-epithelialisation typically occurs within 24–48 h, followed by subepithelial synthesis of new collagen, reorganisation of the epithelium, production of type VII collagen (a major component of anchoring fibrils), proliferation of reactive keratocytes, and increase in clinically visible subepithelial haze that decreases within 6 months [166, 167]. Between 6 and 15 months, the epithelium is thicker than normal, Bowman’s layer remains absent, and superficial stromal scarring with an increase in the number of keratocytes has developed in the area of ablation (Fig. 3.18b). Ultrastructural studies show that the epithelial basement membrane has focal discontinuities. The stroma underlying and surrounding the scar remains normal [168].
Laser In Situ Keratomileusis
Definition
In laser in situ keratomileusis (LASIK), a mechanical microtome or a femtosecond laser is used to create a hinged corneal flap that is 120–180 μm in thickness and has a diameter of 8–10 mm. The flap is lifted, and excimer laser ablation of the corneal stromal bed is performed. Finally, the flap is repositioned without sutures.
Clinical Features
LASIK provides advantages compared to photorefractive keratectomy (PRK) including no significant central corneal haze, faster visual recovery, less postoperative discomfort, and less myopic regression [169]. Unlike after incisional refractive surgery, eyes that have undergone LASIK do not have an increased risk for globe rupture compared to normal eyes [161]. Corneal ectasia is a devastating complication after LASIK and, less frequently, after PRK.
Histopathology
In animal studies, apoptosis of keratocytes occurs 1 day after LASIK in a zone approximately 50 μm anterior and posterior to the lamellar flap incision [170]. Proliferation, migration, and differentiation of keratocytes into myofibroblasts and inflammatory cell infiltration are observed during the following 3 months [171, 172].
Histopathology of human corneas obtained at autopsy 2 months to 6.5 years after uncomplicated LASIK showed focal epithelial hypertrophic modifications, focal undulations in Bowman’s layer, and a variably thick lamellar stromal interface scar in all specimens with variable degrees of epithelial ingrowth at the peripheral wound. The flap wound margin, which was adjacent to the epithelium, healed by producing an approximately 8-μm-thick hypercellular fibrotic stromal scar, whereas the central and paracentral wound regions healed with a thinner hypocellular primitive stromal scar. The stroma contained eosinophilic deposits, periodic acid-Schiff-positive, electron-dense granular material interspersed with randomly ordered collagen fibrils, increased spacing between collagen fibrils, and widely spaced banded collagen. Immunofluorescence microscopy identified increased type III collagen and myofibroblasts in the hypercellular fibrotic scar regions and decreased or absent amounts of all corneal stromal components other than type I collagen in the hypocellular primitive scar regions [173, 174].
Prognosis
Intrastromal epithelial ingrowth with or without cyst formation can occur after LASIK (Fig. 3.18c). Histopathological and ultrastructural findings in cases with corneal ectasia following refractive surgery include epithelial hypoplasia, breaks in Bowman’s layer, stromal thinning in the ectatic region, and a thin residual stromal bed. Studies suggest that interfibre fracture with interlamellar and interfibrillar biomechanical slippage occurs as is also seen in keratoconus [175].
3.5.3.6 Tissue Adhesive
Definition
Tissue adhesives are used to strengthen or seal ulcerating and perforated corneas.
Clinical Features
Histopathology
Effects of surgical adhesives in the rabbit cornea included neovascularisation and a noticeable inflammatory response surrounding cyanoacrylate adhesive [179].
3.5.3.7 Conjunctival Flap
Definition
Creation of a conjunctival flap for the treatment of chronic, non-healing corneal ulceration especially in eyes with poor visual potential was described by Gunderson in the late 1950s and has become a standard procedure [54]. A pedunculated flap of bulbar conjunctiva is mobilised and secured with sutures over the cornea.
Histopathology
The integrity of the globe is successfully maintained in most eyes, but retraction or dehiscence of the flap with re-exposure of the cornea, infection, progression of the inflammation beneath the flap, loss of the flap from epithelial ingrowth, and epithelial cyst formation have been described [180].
3.5.3.8 Amniotic Membrane Transplantation
Definition
Amniotic membrane transplantation is used increasingly to treat persisting epithelial defects, corneal ulcers, and alkali burns. Amniotic membrane is the innermost avascular layer of the placenta and consists of a monolayer of cuboidal epithelium, a basement membrane, and a stromal layer [181].
Clinical Features
Used as a graft, amniotic membrane is intended to provide a new basement membrane for corneal epithelial cells (Fig. 3.19a). Used as a biological shield, growth of new corneal epithelium is promoted by growth factors released beneath the amniotic membrane.
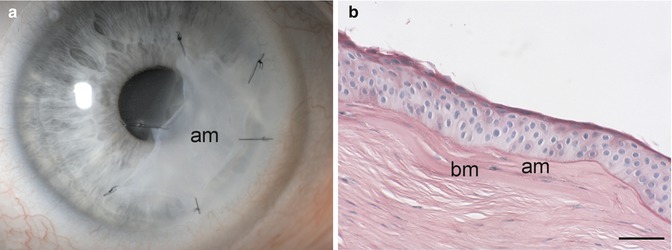
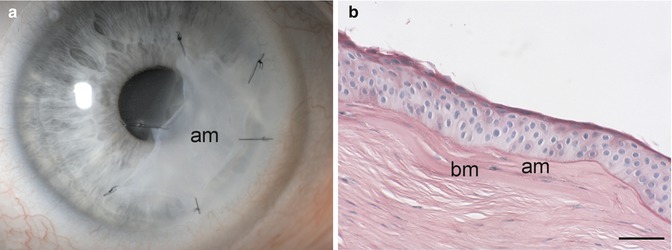
Fig. 3.19
Amniotic membrane transplantation. Amniotic membrane (am) graft for persistent corneal trophic ulcer (a). The amniotic membrane (am) graft is integrated into the cornea subepithelially. Note loss of Bowman’s layer (bl) centrally and the subepithelial pannus that replaces it (b). Periodic acid-Schiff, bar = 50 μm
Histopathology
Histological evidence confirms that an amniotic membrane can be integrated into the corneal surface and can persist for months in one of four patterns: (1) intraepithelial, (2) subepithelial (Fig. 3.19b), (3) intrastromal with various grades of retraction, and (4) superficial (disintegrated) [182]. Firm adhesion between the amniotic membrane and corneal epithelial cells is established by newly formed basement membrane, interdigitating microvillous cell processes, and formation of desmosomes and gap junctions [182–184]. Transformed corneal stroma-derived cells migrate from the anterior stroma through breaks in Bowman’s layer into the connective tissue of the amniotic membrane, integrating the membrane with corneal tissue and allowing healing of the corneal stroma [185].
3.5.3.9 Limbal Stem Cell Transplantation
Definition
Reconstruction of the ocular surface in patients with limbal stem cell insufficiency (LSCI) and subsequent conjunctivalisation of the corneal surface remains challenging. The objective of limbal stem cell transplantation is to restore the corneal surface with normal corneal epithelium.
Clinical Features
In unilateral total LSCI, a limbal autograft from the healthy eye is transplanted in order to prevent an immune rejection. In bilateral, total LSI a cadaver or a living limbal allograft from a relative is used for transplantation followed by systemic immunosuppression [186].
Histopathology
Rabbit corneas showed a decrease in corneal vascularisation after surgical removal of their limbal area and consecutive limbal transplantation and, even more importantly, a corneal phenotype of the transplant-derived regenerated epithelium [187]. Donor-derived epithelial cells were detected long term in the paracentral cornea of patients after limbal allograft transplantation [188].
3.5.3.10 Keratoplasty
Penetrating Keratoplasty
Definition
Penetrating keratoplasty (PK) refers to surgical removal of the central diseased cornea with replacement by full-thickness corneal donor tissue, usually cadaveric. It is performed for optical, tectonic, or therapeutic reasons.
Epidemiology
In clinical situations with a low risk of rejection, PK is one of the most successful transplantations, with a 10-year success rate, defined as corneal clarity, of up to 92 % without routine human leucocyte antigen (HLA) matching or systemic immunosuppression [189]. In high-risk situations such as when the cornea is vascularised, after previous grafting, or when using a large graft, success rates drop to 40–50 % [53, 190].
Histopathology
In experimental corneal graft rejection, any or all of three layers of the cornea can be involved. In epithelial rejection, congestion of limbal vessels is followed by the appearance of an elevated epithelial rejection line that migrates centrally. This type of rejection is benign. Stromal rejection can manifest as subepithelial infiltrates, which respond readily to topical steroids. Diffuse stromal rejection in combination with an endothelial rejection is observed in rabbit models, but is rare in humans [191].
Endothelial rejection with an endothelial rejection line composed of lymphoid cells, known as the Khodadoust line, will develop in approximately 20 % of KP patients [192]. Untreated, this rejection proceeds across the donor endothelium leaving damaged endothelium behind. Keratic precipitates composed of lymphoid cells and an anterior chamber reaction are typically present.
Lamellar Keratoplasty
Anterior Lamellar Keratoplasty
Definition
Anterior lamellar keratoplasty (ALK) is defined as the removal and replacement of deformed or diseased anterior corneal tissue while sparing the Descemet’s membrane and endothelium. Midstromal dissection is associated with interface scarring. Therefore, deep anterior lamellar keratoplasty (DALK) with or without baring of Descemet’s membrane is now preferred [193].
Clinical Features
Anterior lamellar keratoplasty is indicated for the treatment of corneas that have a healthy endothelium but have pathological changes anterior to Descemet’s membrane such as anterior stromal scars or keratoconus. Various procedures have been designed to facilitate separation of the posterior stroma. Currently, the most popular is dissection by insufflated intrastromal air (“big bubble” technique).
Histopathology
Intrastromal air insufflation may complicate the histopathological workup of excised corneal tissue, which often floats in formalin (Fig. 3.20a). In one study, 58 % of corneal buttons were affected by air emphysema, 5 % by epithelial edema related to the hydrodelamination procedure that mimicked bullous keratopathy, and 1 % were lost specimens. Histopathological evidence of conus formation could not be detected in 7.4 % of DALK specimens as compared to 1.6 % of PK specimens of keratoconus [194].
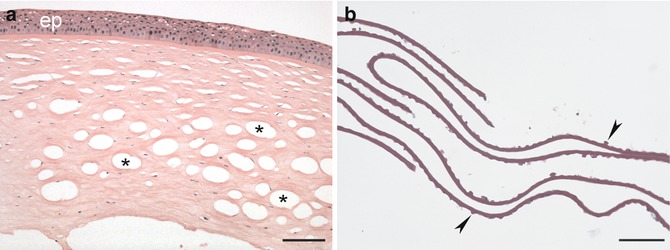
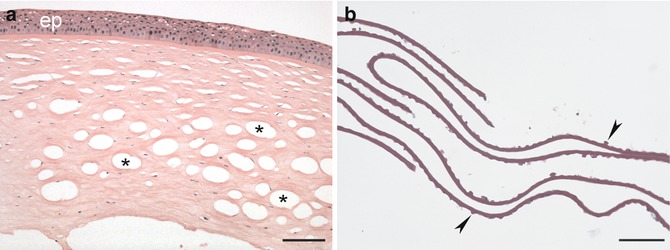
Fig. 3.20
Lamellar keratoplasty. Deep anterior lamellar dissection with intrastromal air injection. Note epithelial edema (ep) and massive intrastromal air emphysema (asterisks) related to the surgical procedure (a). Descemet’s membrane with excrescences (arrowheads) and loss of endothelial cells compatible with Fuchs’ endothelial dystrophy from posterior lamellar keratoplasty (b). Haematoxylin-eosin, bar = 100 μm (a), and periodic acid-Schiff, bar = 50 μm (b)
Posterior Lamellar Keratoplasty
Definition
Posterior lamellar keratoplasty is the replacement of the corneal endothelium without corneal surface incisions or sutures in the management of corneal endothelial disorders. The most popular procedure is known as Descemet’s stripping automated endothelial keratoplasty (DSAEK). The graft contains endothelium, Descemet’s membrane, and a thin layer of donor corneal stroma. In Descemet’s membrane endothelial keratoplasty (DMEK), only Descemet’s membrane and endothelium are transplanted.
Clinical Features
Posterior lamellar keratoplasty is indicated for replacement of diseased or damaged endothelium such as in Fuchs’ endothelial corneal dystrophy or pseudophakic bullous keratopathy. After unfolding and proper orientation of the posterior transplant, an anterior chamber air bubble is insufflated to support attachment of the graft.
Histopathology
Examination of failed grafts after DSAEK reveal attenuation of endothelial cells, fibrocellular tissue in the graft-host interface, retained Descemet’s membrane, epithelial ingrowth, or a combination thereof. Decentred DSAEK grafts may have full-thickness corneal layers at one edge [195]. In addition, metaplasia of attenuated corneal endothelial cells with formation of an abnormal posterior collagenous layer may contribute to an impaired visual function despite complete graft adherence after DMEK [196]. Pathologists have to get used to seeing specimens with only Descemet’s membrane and a variable number of endothelial cells. The diagnosis of endothelial disease must be established without analysing epithelial and stromal pathological features (Fig. 3.20b).
Prognosis
Early visual recovery and excellent postoperative functional results have been reported. The major complication is incomplete attachment of the graft in the early postoperative period, leading to repositioning with air bubbles. Inadvertent grafting of a partially full-thickness graft in DSAEK may lead to epithelial ingrowth between the graft and host cornea or into the anterior chamber. Causes of primary donor failure in DMEK include upside-down positioning of the graft and endothelial cell loss due to excessive surgical manipulation [197].
3.5.3.11 Keratoprosthesis
Definition
In patients with numerous failed keratoplasties or when keratoplasty otherwise has been deemed to be hopeless, keratoprosthesis surgery is performed (Fig. 3.21).
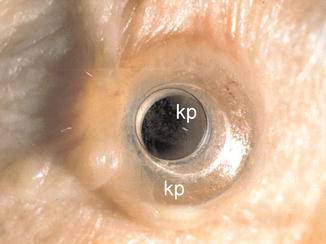
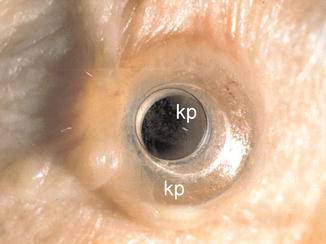
Fig. 3.21
Osteo-odonto keratoprosthesis. Keratoprosthesis (kp) in a patient with end-stage mucous pemphigoid. The base of the prosthesis is intrastromal
Clinical Features
Current approaches include an osteo-odonto-keratoprosthesis made of an osteodental lamina as a skirt for a polymethyl methacrylate (PMMA) cylinder, a hydrogel sheet with porous edges, and double-plated PMMA devices with holes for nutrition and hydration of a carrier corneal graft. Complications after keratoprosthesis surgery include surface complications, chronic inflammation, retroprosthesis membrane formation, glaucoma, endophthalmitis, and keratoprosthesis extrusion [198–200].
Histopathology
A transparent tissue layer consisting of non-keratinised squamous epithelium of corneal origin can cover the anterior surface of the keratoprosthesis representing bio-integration of the device [201].
Histopathology of failed keratoprostheses revealed invasion of the porous hydrogel keratoprosthesis by reactive fibroblasts and multinucleated foreign-body giant cells. In the area of dehiscence, thinning, and lysis of collagen fibres, infiltration by lymphocytes and plasma cells with a sheet of fibroinflammatory tissue, extending into the anterior chamber, was evident [200].
Retroprosthetic membranes occur in 25–65 % of patients with the double-plated PMMA keratoprosthesis. They represent stromal downgrowth from the host corneal stroma. These membranes grow through gaps in Descemet’s membrane to reach behind the back plate and may adhere to the anterior iris surface [202]. In patients with inflammatory preoperative diagnoses, massive inflammatory cell infiltration, tissue necrosis with stromal melting adjacent to the transcorneal stem of the prosthesis and epithelial fistula formation may be observed [203].
In a case of failed osteo-odonto-keratoprosthesis, the osteodental laminae showed various degrees of transformation and resorption with associated inflammation and downgrowth of keratinising squamous epithelium [204].
Prognosis
Long-term success is good in noninflammatory situations; however, prognosis is guarded for patients with autoimmune disease such as pemphigoid, Stevens-Johnson syndrome, severe chemical burn, and uveitis [203]. When the alveolar-dental ligament is preserved in osteo-odonto-keratoprosthesis, long-term survival of the prosthesis has been reported [205, 206].
3.6 Degenerations and Dystrophies
3.6.1 Degenerations
3.6.1.1 Keratoconus and Keratoglobus
Definition
Keratoconus and keratoglobus refer to an idiopathic bilateral corneal ectasia which is conical or globular in shape, respectively.
Epidemiology
Etiology
The pathophysiology of keratoconus remains open. Both genetic and environmental factors, including rubbing of the eyes and autosomal dominant inheritance with incomplete penetrance, have been proposed and both may play a role [207, 209, 210]. Genetic mapping has identified candidate loci on chromosomes 1, 2, 3, 5, 8, 9, 13, 14, 15, 16, 20, and 21, suggesting genetic heterogeneity. Most cases are sporadic. Keratoconus is frequent in some syndromes including Down’s syndrome, Marfan’s syndrome, and Leber’s congenital amaurosis. It is associated also with atopy and vernal keratoconjunctivitis.
Clinical Features
Keratoconus presents with regular myopic astigmatism, followed by irregular astigmatism and poor visual acuity once this cannot be adequately compensated with spectacles [211]. Progressive axial steepening and ectasia are associated with stromal thinning (Fig. 3.22a, b). Focal anterior stromal scarring at the apex of the cone, an iron line around the base of the cone (Fleischer’s ring), and vertical stress lines in the stroma (Vogt’s striae) are common. Descemet’s membrane may eventually break leading to acute corneal swelling (hydrops).
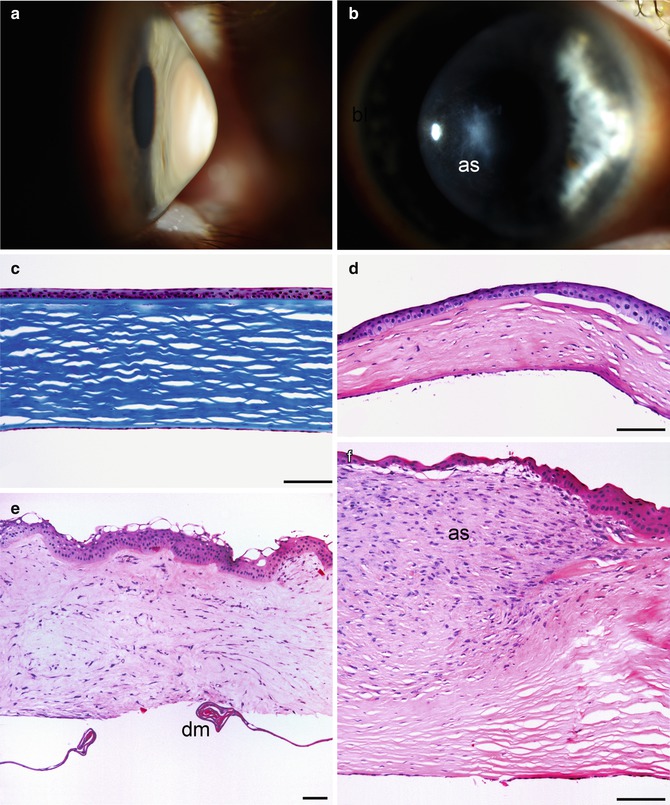
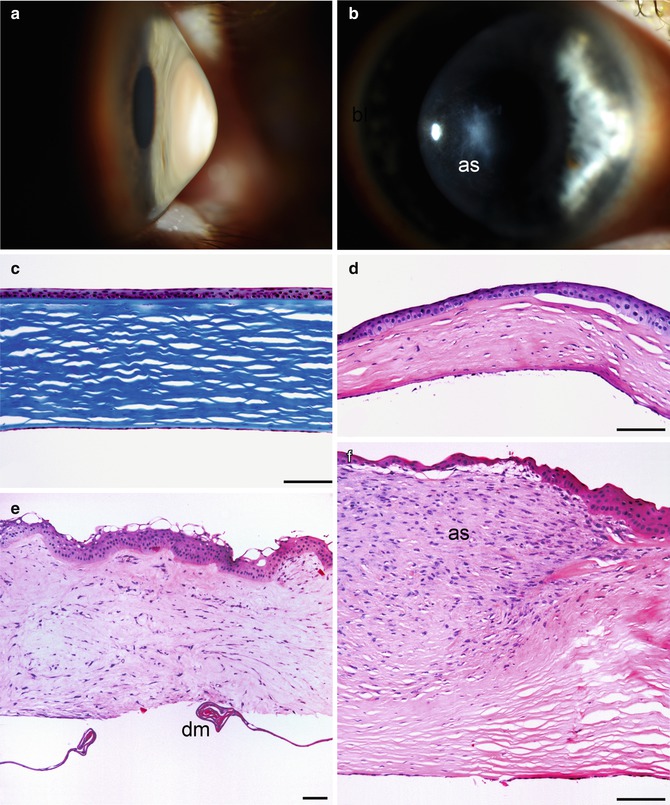
Fig. 3.22
Keratoconus. Conical ectasia of the cornea just below the optic axis (a). Greyish apical scar (as) from chronic stretching that has led to breaks in Bowman’s layer and a subsequent reparative reaction (b). Moderate keratoconus: corneal epithelium and stroma are thinner than average (300 μm) but otherwise normal, and Bowman’s layer is intact (c). Advanced keratoconus: Bowman’s layer is destroyed at the apex of the cone, stroma is extremely thin (120 μm), and stromal lamellae are irregular from scarring (d). Complicated keratoconus: epithelial edema with stromal swelling (hydrops) and disorganisation after rupture of Descemet’s membrane (dm); note curling of the ends of the ruptured membrane (e). Complicated keratoconus: exuberant hyperplastic apical scarring (as) after repeated rupturing of Bowman’s layer at the apex of the cone (f). Masson’s trichrome (c), haematoxylin-eosin (d–f), bars = 100 μm
Histopathology
The epithelium and stroma are abnormally thin at the apex of the cone (Fig. 3.2c, d) [207, 212, 213]. Descemet’s membrane and endothelium are generally normal, but in more advanced cases the endothelial density may be reduced at the base of the cone, and the membrane demonstrates a break in acute hydrops (Fig. 3.22e). Later, the break is sealed by migrating endothelial cells and newly deposited basement membrane. Bowman’s layer frequently has breaks that may be associated with anterior stromal scarring at the apex of the cone (Fig. 3.22f). In keratoglobus, corneal thinning is either uniform or circumferential, and Bowman’s membrane tends to be absent [214].
Prognosis
Keratoconus is progressive but varies widely in severity. Mild cases stabilise by the age of 40 years without need for surgery. In more aggressive cases, corneal collagen cross-linking or intracorneal rings slow progression (see Sect. 3.5.3.1–3.5.3.2). Rapidly progressive cases benefit from anterior lamellar or penetrating keratoplasty, which has an excellent prognosis in keratoconus. Keratoglobus is more difficult to manage surgically.
3.6.1.2 Pellucid Marginal Degeneration
Definition
Pellucid marginal degeneration refers to a bilateral corneal ectasia located at the inferior periphery of the cornea, which is considered different from keratoconus and keratoglobus.
Synonyms
Keratotorus.
Epidemiology
A rare degeneration usually diagnosed at the age of 20–40 years.
Etiology
This degeneration is idiopathic.
Clinical Features
Pellucid marginal degeneration is asymptomatic except for progressive irregular astigmatism [215]. The thinning typically affects the inferior cornea from the 4 o’clock to the 8 o’clock meridian and is separated from the limbus by 1–2 mm of normal cornea. Corneal hydrops is very rare.
Histopathology
Light microscopic findings mimic those of keratoconus but are located in the corneal periphery and the thinning is more abrupt toward the central cornea [216]. Descemet’s membrane is typically intact even when the ectasia is very advanced.
Prognosis
Pellucid marginal degeneration varies widely in severity. Keratoplasty is difficult because of the location of the thinning.
3.6.1.3 Bullous Keratopathy
Definition
Bullous keratopathy means corneal swelling that results from insufficiency of the corneal endothelial pump.
Epidemiology
This degeneration is common and affects, among others, 0.1 % of patients undergoing cataract surgery. It is mainly affects the elderly because of age-related loss of the endothelial cells and the need for intraocular surgery for cataract or glaucoma.
