Color Doppler Imaging in Ophthalmology
Mitesh K. Kapadia
Suzanne Freitag
Robert Sergott
Tom Hedges
Color Doppler imaging (CDI) combines two-dimensional (2D) ultrasonography with Doppler spectral analysis to evaluate vascular structures and blood flow velocities. CDI was first described in 1979 and used in the imaging of various nonophthalmic organ systems.1 Erickson2 first described its use in the orbital region in 1989 and CDI has been used to study a number of ophthalmic disorders since that time. The first part of this chapter will discuss background information related to the technique of ophthalmic CDI and the second part of the chapter will discuss the use of CDI in specific ophthalmic disorders.
Background
Johann Christian Doppler was born in 1805 and died at the age on 49, having gained little acceptance during his lifetime of the theory which now carries his name (Fig. 1).3 His work postulated that the frequency of sound increases as an observer and a source move toward each other and decreases as they move apart.
In 1845, Buys Ballot verified Doppler’s theory by borrowing a locomotive and having a trumpet player stand on the moving train, while an observer who had perfect pitch stood at the station. Compared to when the locomotive was stationary, the pitch of the trumpet was a half a note higher when the locomotive was moving toward the listener and half a note lower when moving away.3
The quantitative form of Doppler’s equation is as follows:
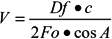
where V=velocity, Df = Doppler frequency shift, c=speed of sound, Fo = frequency of the sound source, and A=angle of incidence of the observer relative to the source.
Ophthalmic CDI uses this equation to measure blood flow velocity. Fo is the frequency emitted by the ultrasound transducer. Df is found by measuring the ultrasound frequency returning to the transducer and subtracting this value from the frequency of emission (Fo). The maximum Doppler shift occurs when A = 0 degrees. When measuring blood flow, the angle A can be a significant source of error if it is greater than 45 degrees. When A is close to 90 degrees, it becomes difficult to differentiate forward from backward flow and these values may be averaged by the transducer, producing spurious results.
Technique
To perform CDI of the orbit, the patient is placed in a supine position with the eyes closed and gaze directed at the ceiling. The ultrasound transducer is applied to the closed eyelid using a coupling agent such as methylcellulose. Care is taken to avoid excessive pressure on the globe, which may result in significant errors during measurements. A modification attaching the ultrasound probe to a slit lamp for measurements in seated patients has been described.4
Scans are made through the eye and orbit in multiple planes to identify the desired structures and vessels. After the desired vessel is located and the angle of incidence recorded, a few seconds of Doppler waveform are recorded. The system analyzes the Doppler waveform and calculates the peak systolic and peak diastolic blood flow velocities based on the Doppler equation described earlier. Normal values for orbital vessels are shown in Table 1.
TABLE 1. Normal Orbital Color Doppler Parameters | |||||||||||||||||||||||||||||||||||
---|---|---|---|---|---|---|---|---|---|---|---|---|---|---|---|---|---|---|---|---|---|---|---|---|---|---|---|---|---|---|---|---|---|---|---|
|
The pulsatility index (PI), a measure of the impedance or total resistance to flow is calculated from the difference in systolic and diastolic velocities. A number of different pulsatility indices have been developed. One commonly used with CDI is Gosling’s PI, which is calculated with the equation:
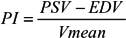
where PSV=peak systolic velocity, EDV=end diastolic velocity, and Vmean=average velocity. Another is resistive index (RI) or Pourcelot’s ratio, which is calculated according to the formula:
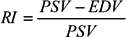
These indices are reported as ratios or percentages with a value of 0 or 0% denoting no resistance and 1 or 100% denoting maximum resistance.
The display of the imaging machine is designed to display a 2D grayscale image of static structures with Doppler information superimposed in color. The sclera, extraocular muscles, and optic nerve are easily visualized, providing an anatomical reference for identifying orbital vessels. Of course, intraocular structures visible with standard, non-Doppler ultrasound, such as the cornea, lens, and iris, can be visualized as well.
By convention, the color red is used to designate flow toward the transducer and blue to designate flow away from the transducer. When orbital vessels are examined through closed eyelids, the ultrasound beam is nearly parallel to the vessels; therefore, arterial flow is red and venous flow is blue. Arteries and veins are also differentiated by the pulsatile nature of flow in arteries in contrast to the more continuous flow seen in veins. Even vessels that lie in close proximity, such as the central retinal artery (CRA) and CRV, are easily distinguished using this technique (Figs. 2 and 3).
![]() FIGURE 2. Simultaneous Color Doppler image of normal CRA (red), CRV (blue) and ophthalmic artery (red). |
One of the limitations of CDI is that it measures blood velocity, and not blood flow. The relationship between blood flow and velocity is related to the radius of the vessel according the following equation:

where Q = blood flow, V = velocity, and r = radius of the vessel. In orbital CDI, the radius of orbital vessels is too small to determine accurately; therefore, blood flow can not be measured directly. Instead, blood flow velocities are measured and serve as an indirect marker of blood flow volume.
Changes in blood flow velocity accurately reflect changes in blood flow volume as long as the radius of the measured vessel remains constant from between measurements. Imaging a vessel along an area of vascular stenosis may reveal an erroneously high velocity, because at a constant flow rate, velocity must increase according to the formula shown above.
Orbital Vascular Anatomy
Detailed knowledge of orbital vascular anatomy is required to locate desired vessels (Fig. 4). The internal carotid artery (ICA) gives rise to the ophthalmic artery (OA), which travels beneath the intracranial portion of the optic nerve and enters the orbit within a layer of dura through the optic canal. The OA then curves around the lateral aspect of the optic nerve to run in a supermedial position in relation to the optic nerve. Along its orbital path, the OA gives off several branches, the first of which is the CRA. This branch enters the dural sheath of the optic nerve 10 to 15 mm behind the globe and passes through the optic nerve into the retina. The OA also gives off two long posterior ciliary arteries that supply the iris and ciliary body, as well as six to eight short posterior ciliary arteries that branch into 10 to 20 divisions and enter the posterior globe to supply the choroids and optic nerve. Other branches of the OA include the lacrimal artery, anterior and posterior ethmoidal arteries, supraorbital arteries, and branches to the extraocular muscles.
The venous drainage of the eye and orbit is mainly via the superior ophthalmic vein, which is formed from several vessels including the central retinal vein (CRV), vortex veins, and inferior ophthalmic vein. The superior ophthalmic vein forms near the tendon of the superior oblique muscle and runs under the tendon the superior rectus muscle and above the optic nerve within the muscle cone in a lateral and posterior direction. Then it tracks along the lateral orbital wall and finally passes through the superior orbital fissure to drain into the cavernous sinus.
Orbital vessels commonly imaged using CDI include the OA, CRA, CRV, superior ophthalmic vein, and short posterior ciliary arteries. Generally the ultrasonographer starts by identifying the optic nerve. The OA, the largest artery in the orbit, can be found superomedial to the optic nerve in the anterior orbit in approximately 85% of individuals.11,12 The velocity waveform of the OA shows a high and steep peak systolic value with a rapid decrease to a low end diastolic velocity (Fig. 5). Often a dicrotic notch, which corresponds to aortic valve closure, is seen dividing the diastole waveform. Normal velocities are compiled from various sources and are listed in Table 1.
![]() FIGURE 5. Normal Color Doppler image and waveform on an OA. Normal peak velocity 38 +/-11 cm/second. |
The CRA can be found most easily within the optic nerve for approximately 12 mm behind the globe, where it runs a fairly straight course. It is usually located nasal to the adjacent CRA. The transducer can be positioned so that the C RA and CRV are visualized simultaneously. The velocity waveform of the CRA is similar, but less steep, than that of the OA. Blood flow through the CRA can be affected by intraocular pressure; therefore, care must be taken not exert excessive pressure on the globe with the transducer.
The CRV is located temporal to the CRA within the optic nerve. It has a much smoother waveform than the CRA, but does exhibit some pulsatility.
The posterior ciliary arteries can be found 10 to 20 mm behind the globe surrounding the optic nerve and are variable in number. The long posterior ciliary arteries may be seen entering the globe adjacent to the ciliary body with the aid of a gelatin standoff device placed between the globe and the transducer. The waveforms are similar to that of the CRA, but may be less pulsatile. The reproducibility of velocity values for the posterior ciliary arteries is poorer than for the larger vessels because it sometimes difficult to determine how many vessels are being imaged simultaneously. In addition, there may be significant vascular tortuosity, especially in the posterior orbit where vessels may be imaged as they bend back on themselves, introducing errors in measurements. Intravenous injection of an echogenic contrast material may improve visualization of smaller caliber vessels.13
The superior ophthalmic vein lies above the optic nerve in the mid-orbit. The flow pattern and direction of flow are variable within this vessel. Usually, there is a low-flow continuous waveform that may show some fluctuation with the respiratory cycle. This vessel can be difficult to locate because of its low-flow nature, but is reported to be found in 90% of normal individuals.2,14 Asking a patient to perform a Valsalva maneuver may help enlarge the vessel for easier localization but may reverse flow in the vein, making it appear arterial.
Safety
CDI of the eye and orbit is thought to be very safe. Ultrasound has been shown to cause choroidal lesions and cataracts in animal eyes when very high energies were used,15,16 but this level of energy is not typically encountered in clinical studies on human patients. During CDI examination, in situ peak intensity may reach 77 mW/cm2,17 which does exceed United States Food and Drug Administration recommendations, but is below maximum levels recommended by the American Institute for Ultrasound in Medicine and the British Medical Ultrasound Society.17 The portion of CDI when high intensities are used is brief, and to our knowledge, no adverse effects have been reported.
CDI in Ophthalmic and Systemic Disease
Systemic Variables
Systemic factors such as age and blood pressure clearly have important effects on measured velocities. Systolic blood pressure is correlated with peak systolic velocities in both the ophthalmic and central retinal arteries.18 In a large study of 140 healthy volunteers, older patients were found to have decreased flow velocities in the ophthalmic, central retinal and posterior ciliary arteries with a corresponding increase in resistive indices of those vessels.19 The extent of age-related changes may be different in men and women.19,20
Several studies have examined the role of menopause and hormone replacement therapy on orbital hemodynamics. Hormone replacement therapy decreases parameters of vascular resistance in the CRA21,22 and OA,23,24 but does not seem to affect the posterior ciliary arteries.24 In one study of postmenopausal women who do not take hormone replacement, the relative amounts of the sex hormones estradiol and testosterone showed significant correlations with orbital hemodynamic parameters. The RI of the CRA tended to decrease with increasing levels of estradiol and increase with increasing levels of testosterone.25
Carotid Occlusive Disease and Ocular Ischemic Syndrome
CDI is commonly used in the diagnosis of carotid artery disease, and the relationship between carotid artery stenosis and orbital blood flow has been studied extensively. A Color Doppler image of carotid artery stenosis is shown in Figure 6.
![]() FIGURE 6. Color Doppler image of a stenotic ICA. Note red and blue colors within lumen, indicating turbulent loss of normal laminar flow. |
Compared to normal controls, eyes with ocular ischemic syndrome have lower velocities in the central retinal and posterior ciliary arteries as well as higher pulsatility indices in these vessels.32 Carotid endarterectomy improves orbital hemodynamic parameters to near normal levels in about half of patients.33 Patients with the greatest reduction in orbital flow velocities appear to be at increased risk for developing iris neovascularization,34 suggesting that CDI may be useful in identifying patients who need more frequent follow-up.
Reversal of flow in the OA is a frequent finding in patients with ocular ischemic syndrome. Some authors claim that this finding is highly specific to ocular ischemic syndrome,32 whereas other authors state that this finding may also be found in patients with carotid stenosis without ocular ischemic syndrome.33,35
Reynolds et al36 studied 304 carotid arteries in 152 consecutive patients presenting for carotid ultrasound. They found that OA flow was reversed in 56 of 101 patients with greater than 80% stenosis of the carotid artery, but that the direction of OA flow was never reversed in patients with less than 80% stenosis. Thus, in their study, reversal of flow in the OA was highly specific for severe carotid artery stenosis. Patients were not tested for ocular ischemic syndrome in this study.
Carotid-cavernous fistulas
In carotid-cavernous fistulas there is a communication between the cavernous sinus and either the ICA or dural branches of the internal or external carotid artery. This can lead to the reversal of blood flow in the superior ophthalmic vein (Fig. 7), as well other orbital and conjunctival veins, because of elevated pressure in the cavernous sinus. Flaherty et al37 reported the use of CDI in three patients with carotid-cavernous fistulas, demonstrating enlargement of the superior ophthalmic vein and a pulsatile, arterialized pattern of blood flow in this vessel. The 2D ultrasound portion of the examination may highlight other aspects of carotid-cavernous fistulas, such as enlargement of the extraocular muscles and a dilated superior ophthalmic vein. The utility of CDI in both the diagnosis and the monitoring of treatment of carotid-cavernous fistulas has been confirmed by several other reports.38,39,40
Orbital lesions
CDI has proven to be a useful tool in the evaluation of patients with orbital lesions of both vascular and nonvascular origin. Lieb et al41 reported a case of a clinically suspected orbital varix that was examined with CDI. No vascular abnormalities were noted on initial exam. When the patient performed a Valsalva maneuver, an area of vasculature was noted to expand with blood flow moving toward the transducer. As the patient exhaled, the direction of blood flow reversed and the lesion became smaller. Because of the dynamic, instantaneous nature of ultrasound examination, it may be easier to demonstrate these lesions using CDI compared to other imaging techniques, such as computed tomography.
Verity et al42 used CDI to study 24 children with capillary hemangiomas. Lesions typically had flow velocities that were two- to three times the velocity of normal capillaries. Abnormal velocities were found in the region of the tumors even after complete clinical resolution. Other orbital lesions studied by CDI include superior ophthalmic vein thrombosis, cavernous hemangiomas, and arterovenous malformations.43,44
Hatton et al45 and Orawiec et al46 reviewed their experience using CDI to help differentiate between benign and malignant orbital lesions. They found that tumors with significant internal blood flow tended to be malignant, whereas benign lesions tended to have minimal to no blood flow.
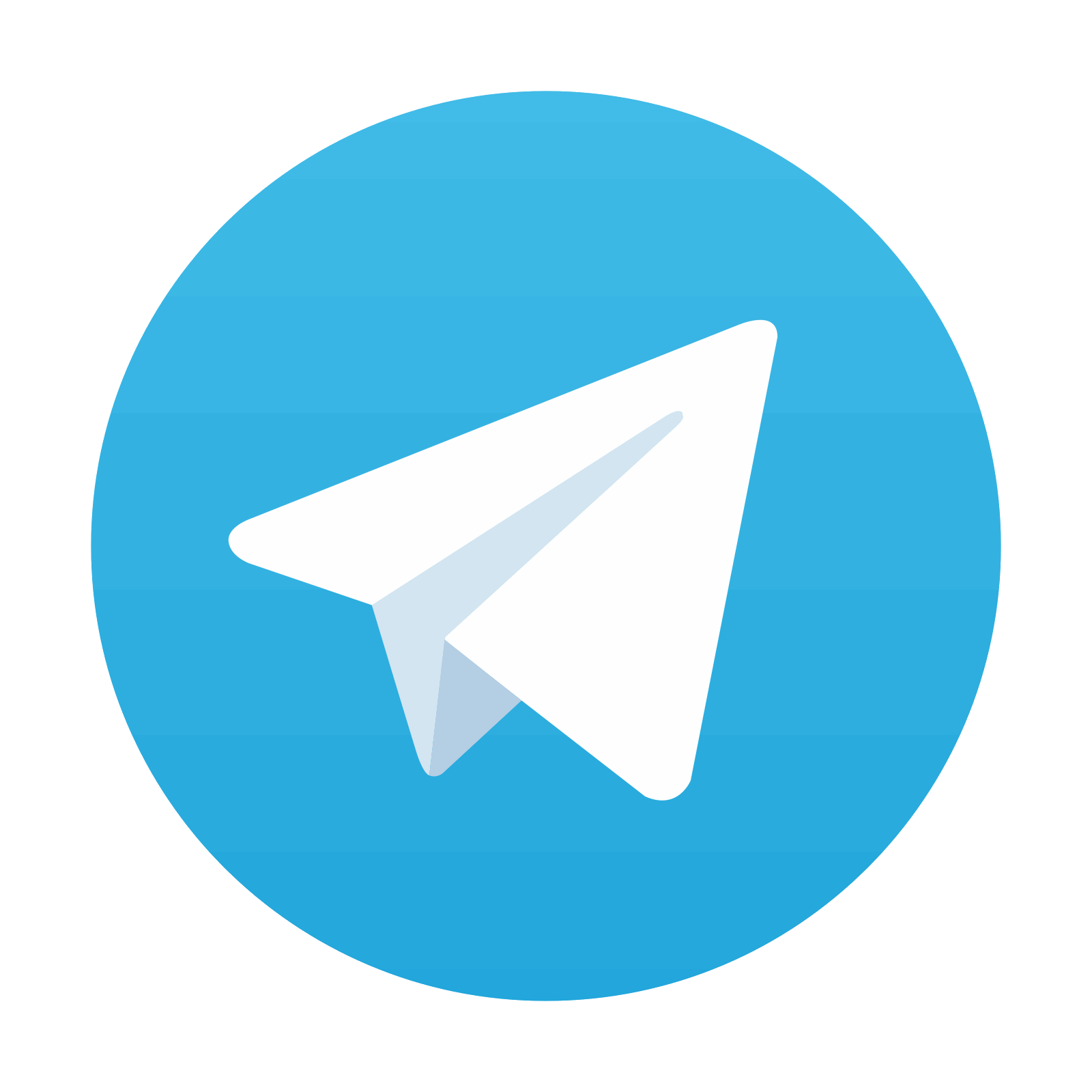
Stay updated, free articles. Join our Telegram channel

Full access? Get Clinical Tree
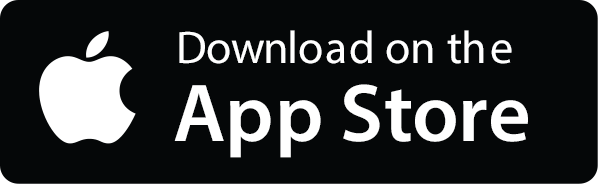
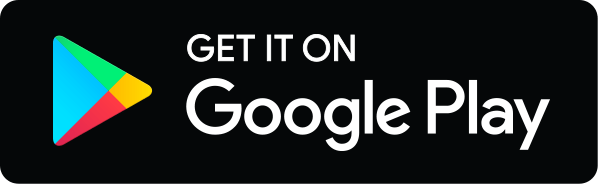