15 Cochlear Implants and Auditory Brain Stem Implants Cochlear implants serving as neuroprostheses have had a considerable influence in the field of otology. Replacing the function of the sense of hearing with a prosthesis gives patients a chance to reconnect with their environment acoustically. By the fall of 1999, some 32 000 patients throughout the world had so far benefited from these inner ear prostheses, and 80% of these patients are capable of open-set speech understanding. Effectiveness here is characterized by the ability to use the phone. For some five years now, two-thirds of patients provided with a cochlear implant have been children aged around five months or older. More than 50% of the children who have received an implant since the mid-1980s are now capable of attending a mainstream school. The implants that are used in cases of hearing disorders in the inner ear are generally constructed on the same principles; only a few individual features differ. The functioning of the various products, too, is more or less similar. The main differences lie in the number of electrodes, the shape of the electrode array, the material used for the implant, the location of the magnet, and speech coding strategies. However, these differences have no implications either for the indication or for the surgical procedure. One fundamental difference, though, is the procedure required in patients who are unable to receive a cochlear implant due to malfunctioning of the auditory nerve. A prosthesis known as an “auditory brain stem implant” is placed directly on the second neuron of the auditory pathway, the cochlear nucleus complex (CNC), as a replacement for the auditory nerve. More than 80 patients have been provided with multichannel implants in Europe so far, as well as about 120 patients in the USA, in Australia, and in Asia. The results are not comparable to those with the cochlear implant, but nevertheless, 75–85% of the patients who have received this type of implant since 1992 no longer wish to do without the prosthesis. This Chapter is based on the author’s personal experience in about 1000 cases of cochlear implantation and auditory brain stem implantation since 1984 (Laszig and Marangos 1998). The systems used are all built in a similar way (Fig. 15.1). All of the available products work by stimulating the still functioning auditory pathway inside the cochlea. A multitude of studies were conducted on questions of biocompatibility and the effect of chronic electrical stimulation in earlier years, and there have also been more recent studies (Shepherd et al. 1983, Shepherd and Clark 1991, Xu et al. 1997). However, it is not yet entirely clear whether extremely high stimulation rates can be tolerated without damage, and whether they are useful at all. The external components of a cochlear implant system consist of the microphone, the speech processor, and the transmitting coil. The microphone is worn behind the ear like a behind-the-ear hearing aid, and it is connected to the speech processor via a cable. Manufacturers are now offering (BTE) units in which the processor and microphone are combined. This improves convenience, and the cable connection between the processor and microphone, which can sometimes cause problems, is no longer needed. However, the efficiency of these BTE devices is rather limited. They are not convenient for children, but are nevertheless popular among adolescents and adults for cosmetic reasons. It is surprising how little acceptance from patients the BTE processors have met with so far, although it may be due to the costs, which have to be covered by patients themselves. At our hospital, patients are allowed to choose the speech processor for themselves, and we advise parents to choose the larger device initially. The incoming signal is electronically analyzed in the speech processor. It is not clear yet which of the existing speech coding strategies is more effective, or—more importantly—whether the different speech coding strategies have any advantages for different kinds of hearing impairment. The most recent strategies (with acronyms such as ACE, CIS, SPEAK, SAS, etc.) undoubtedly allow improved speech discrimination; however, none of them is superior to any of the others in a statistically significant fashion. To summarize, the electrical stimulation of the auditory pathway provides sufficient information, which can be processed in a complex way within the auditory cortex and can allow speech discrimination with the help of cognitive functions. It appears that a prerequisite for this is placement of the electrodes (up to 22) within the cochlea. As long ago as the early 1960s, Zöllner and Keidel (1963) pointed out, in a little-remarked publication that, in their opinion, about 20 electrodes would be optimal—a recommendation that was based entirely on pure deduction. The speech signal can be coded in time and type with the help of multichannel stimulation inside the cochlea. The higher the number of electrodes, the more flexible is the use of the various modes of stimulation, as the stimulation rate should be lower with a higher duration of stimulation, or the number of the active electrodes should be reduced. Simultaneous or overlapping stimulation of different electrodes provides further opportunities for flexible stimulation. Fig. 15.1 The 24 M cochlear implant system, with the implant (above) and the external part of the system (below), including the speech processor, microphone, and transmitting coil. Some of the characteristics of speech that appear to be important for the transmission of information are known. Speech processors analyze the incoming signals according to their intensity, frequency, spectral pattern, etc. The coding strategies define the way in which these analyzed signals will be transmitted via the electrodes inside the cochlea to the spiral ganglion. At this location “it is established which electrode will be stimulated and when, at what intensity and at what phase of current, in synchronicity with the previous stimulation cycle and the following one. Subjective perceptions such as loudness and pitch level are used for individual fitting of the speech processor. Although there is insufficient space here to explain the different speech coding strategies further, it should be emphasized that a larger number of electrodes and a microchip allowing fast signal processing are useful. There have been dramatic changes in the indication for cochlear implantation, and consequently in patient selection criteria since the 1980s. Strict observation of the initial criteria—such as complete acquired deafness in both ears in an adult patient without any other handicap—was abandoned only two years later. This is due to the continuous growth of knowledge in this field, and advances have been so rapid that we have all been surprised by the extremely positive results. Today, implantation is no longer contraindicated in a multiply handicapped child with residual hearing. Obviously, there are still substantial topics requiring further debate, and current discussions of implantation in both ears show that developments are continuing. With regard to cochlear implantation, apart from the broader medical questions, issues involving costs will also need to be discussed. Since the products are manufactured only in limited numbers, they are expensive. However, the benefits for patients and for society are considerable, as has been shown by several studies in various countries using different models for financing the provision of implants. There is agreement today on the indication for a cochlear implant in cases of residual hearing in both ears in patients who cannot be provided with conventional or other implantable hearing aids. Speech understanding, in these cases, may not be more than 30% of monosyllables at a 70 dB sound pressure level in free field audiometry (Fraysse et al. 1998, Klenzner et al. 1999). However, it can be expected that these limitations will be extended, so that a limit of 50% speech understanding in the same circumstances will become the limit. These indications are only valid for adults and adolescents who are able to make reliable statements. However, further research in this field is still needed, on topics such as objective and additional subjective audiometric testing and diagnosis using imaging procedures. Objective examinations such as electrocochleography, brain stem evoked response audiometry (BERA) and cortically evoked response audiometry (CERA) are useful for further clarification of the cause of hearing problems, including possible psychogenic causes. In adults, we also use subjective promontory testing. This examination (which we also carry out in special cases of explicit residual hearing) is often omitted, but the experience of hearing sensations during this test is astonishing for patients who have not been deaf for very long. The sensations are not related to any type of speech understanding, but they help to motivate the patient and reduce anxiety, with appropriate guidance from the physician. We insist on proceeding quickly in the treatment of children; it should be considered, however, that it is not possible to ascertain the entire hearing capacity with children (i.e., not just the sound threshold alone) as clearly and with as little doubt as in adults. Neither subjective promontory testing nor subjective statements are available in children, so we depend entirely on objective tests. We have found that providing children with hearing aids as soon as possible has proved successful when it was possible to reproduce Jewett V in BERA and action potentials in electrocochleography. In this situation, it can be expected that residual hearing will be found that can be used with conventional hearing aids. Thus, there are sufficient criteria for intensive training with hearing aids. Measuring early and middle latencies using an objective promontory test has not been useful due to stimulus artifacts, and the method and depth of anesthesia influence the registered potentials during electrical stimulation (Marangos et al. 1995). Although statistically valid physiological data regarding the best moment for implantation are not yet available, it should be done as early as possible—but this means early diagnosis as well. We do not approve of rigidly applying specific criteria for patient selection, such as the Freiburg Classification (Löhle 1991), for example, or the so-called bronze, silver, and gold standards. These are all useful guidelines, but in each patient all of the other pieces of the puzzle have to be considered individually, too. The parents’ decision has to be taken into account as an important factor. However, we do insist on an early decision that should lead to implantation even before the second year of age. Carrying out implantation in children under the age of two often still remains wishful thinking; however, the numbers of children who receive implants before the age of four is steadily growing. The situation with children who became deaf due to bacterial meningitis is dramatically different, and this will be discussed in greater detail later in connection with imaging diagnosis. The cause of deafness is not clear in about 50% of our selected patients, as is evident from a survey of 96 children seen between May 1993 and January 1997 (Table 15.1). These children have been provided with cochlear implants at our hospital. Meningitis was the second most frequent cause, even ahead of genetic or syndrome-related causes. The large number of patients with deafness due to rubella embryopathy is surprising. A large vestibular aqueduct can often be seen in syndromes, as well as in cases of genetic deafness. The indication for implantation can therefore be established with certainty in adults with deafness, and with sufficient certainty in children, using subjective and objective tests and conventional audio-logical and audiometric methods. The use of high-resolution computed tomography (CT) and magnetic resonance imaging (MRI) is indispensable today for preoperative diagnosis (Laszig et al. 1988, Frau et al. 1994, Marangos and Aschendorff 1997). The imaging methods are valuable in investigating the causes of the patient’s deafness, as well as for planning the operation. Bony or scar tissue obliteration of the inner ear after bacterial meningitis or after transcochlear fractures can be identified, as well as malformations of the cochlea and inner auditory canal. The results of these examinations therefore have an impact on establishing the indication for implant surgery, as well as on the choice of the ear in which the implant will be placed. The imaging findings can also affect preoperative counseling, since the presence of a cochlear malformation or obliteration means both that the prognosis for rehabilitation will be cautious at best and also that there is a higher surgical risk.
Cochlear Implants
Construction and Functioning
Indication and Patient Selection
Imaging Diagnosis
Cause | n |
Unknown | 47 |
Meningitis | 15 |
Genetic | 10 |
Syndrome-related | 8 |
Cytomegalovirus | 5 |
Asphyxia | 4 |
Rubella embryopathy | 4 |
Ototoxic medication | 3 |
Total | 96 |
CT imaging using appropriate algorithms is preferable for delineating osseous structures; obliteration of the cochlea can also be detected. Particularly in cases of malformation, or after bacterial meningitis with signs of obliteration of the cochlea on the CT, MRI should also be carried out. It is also easier to assess the contents of the inner auditory canal and the structure of the brain stem with MRI, which can provide information about the volume of the cochlear nucleus—important in cases of complex malformation of the organ of hearing.
Bacterial meningitis resulting in deafness no longer represents a challenge for the surgeon. As is well known, the cochlea may become obliterated by scar tissue within 8–12 weeks after bacterial meningitis (Fig. 15.2), and this has medicolegal implications. The challenge lies in offering the parents appropriate counseling to clarify that the situation is an “emergency” one. The good to excellent results obtained in cases of regular implantation of up to 22 electrodes within the scala tympani cannot be expected in patients in whom the cochlea has been completely obliterated—even with new methods of surgery and new developments of prostheses with a shorter electrode array or even two or more electrode arrays, or with central stimulation at the cochlear nucleus complex by an auditory brain stem implant. From the beginning, our aim has been to diagnose obliterations as early as possible using the following monitoring program, with which we have not observed any further cases of complete obliteration during the past six years:
- Monitoring during meningitis: behavioral audiometry, pure tone audiometry, otoacoustic emission, stapedius reflex audiometry, brain stem evoked response audiometry, high-resolution CT.
- Monitoring after
Stay updated, free articles. Join our Telegram channel

Full access? Get Clinical Tree
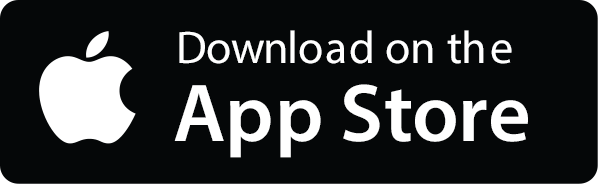
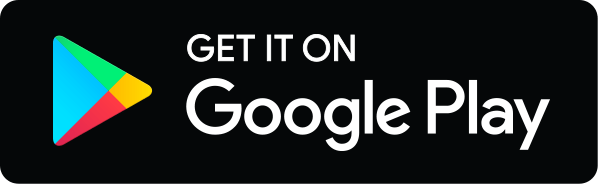