Chemical Injuries of the Eye
Robert A. Ralph
Chemical injuries are among the most urgent of ocular emergencies, often resulting in a dramatic decrease in visual acuity or loss of an eye. The prognosis for a burned eye depends not only on the severity of the injury, but also on the rapidity with which therapy is initiated.
Epidemiology
Ocular chemical injuries can occur under diverse circumstances and in such varied locations as the home, workplace, and school. These injuries are common in industrial chemical laboratories, machine factories, and agriculture, and among laborers and construction workers. They are also frequently reported from fabric mills, automotive repair facilities, and cleaning and sanitizing crews. Chemical burns of the eyes most often occur among the 20- to 40-year-old age group, with young men at greatest risk. Injuries caused by caustic chemicals are among the most severe; the collective prognosis for satisfactory recovery from these injuries is extremely poor.1
In a retrospective study on the incidence and prevalence of ocular chemical burns, 171 consecutive patients were studied during a 1-year interval. Industrial accidents caused 61% of these burns, whereas 37% occurred in the home. The remainder was of unknown origin. The authors reasoned that household chemical accidents are among the most difficult to prevent because of the paucity of safety rules.2 Most of the eye injuries at home result from the use of lime and drain cleaners.1
Automotive battery acid burns can be especially devastating when combined with the shrapnel resulting from explosions3 (Fig. 28.1). These accidents typically occur in the colder months, almost always after dark, and usually involve young men. During recharging of a lead acid storage battery, which contains up to 25% sulfuric acid, hydrogen and oxygen produced by electrolysis form a highly explosive gaseous mixture.4 The most common causes of storage battery explosions are lit matches (used to see battery cells) and the incorrect use of jumper cables. The National Safety Council has frequently advised the public regarding safe use of jumper cables.5
![]() Figure 28.1 Auto battery explosion resulting in ocular chemical injury, corneal laceration, iris tear, and hyphema. |
The deployment of automobile air bags on vehicle impact saves lives and has reduced the risk of serious injury. Activation of the airbag ignites a cartridge of sodium azide, which explosively releases a mixture of nitrogen gas, sodium hydroxide, carbon dioxide, and perhaps even metallic sodium. Talc or cornstarch, used to lubricate the air bag, is also expelled on deployment. The air bag inflates in a fraction of a second, but begins to deflate almost immediately by releasing the gas and powder through holes in the bag. This alkaline material is capable of causing caustic damage to the eyes and must be treated as any other chemical injury.6,7,8,9
Alkali Versus Acid Injuries
In general, alkali injuries are more damaging to the eye than those caused by acids. Alkalies are water-soluble substances that release hydroxyl ions and have a basic pH in solution. On the ocular surface, they saponify cell membranes and intercellular bridges, allowing rapid penetration into the corneal stroma, the anterior chamber, and the vitreous compartments. Cell damage from alkaline agents depends on both the concentration of the alkali and the duration of exposure. The higher the pH, the greater the damage to the eye, with the most significant injuries occurring at a pH of 11 or higher.10 As the pH rises, destruction of the epithelial barrier to penetration becomes progressively more extreme. In the corneal stroma, alkali cations cause damage and necrosis by binding to the mucopolysaccharides and to collagen.11
The most common alkalies involved in ocular injury are calcium hydroxide (lime), potassium hydroxide (potash), sodium hydroxide (lye), and ammonium hydroxide (ammonia). Of these, calcium hydroxide penetrates corneal tissue most slowly because the calcium soaps it forms are relatively insoluble, impeding further penetration of the agent. Potassium hydroxide penetrates corneal tissue a bit faster. Sodium hydroxide penetrates even faster, but ammonia’s transit across the cornea is fastest. If the epithelial barrier is removed, rates of penetration increase until they are nearly equal for all alkalies except ammonium hydroxide, which remains the fastest.10
Ammonium hydroxide passes through the cornea most rapidly because it destroys the epithelial barrier by saponification of the lipoidal cell walls and because it diffuses fastest through the stroma. Ammonia is fat soluble but most other alkalies are not. In addition, other alkalies lack the high mobility of ammonia necessary to permeate layers of cells.10
Acids dissociate in water to form hydrogen ions. The strongest acids have the highest concentration of hydrogen ions in solution. Free hydrogen ions cause cellular necrosis. Weak mineral acids generally cause less severe ocular damage than alkalies, although tissue destruction from strong acids (e.g., sulfuric, nitric, and hydrochloric acids) may be as severe as that from ammonia. The intact epithelium offers moderate protection against penetration of dilute or weak acids, allowing little damage unless the pH is 2.5 or less. However, severe tissue destruction can occur at a pH >2.5 after the epithelium has been removed.10
Acids quickly denature proteins in the corneal stroma, forming precipitates that retard additional penetration. Overall, the rates of penetration for acids at equivalent concentrations and pH vary widely, with sulfurous acid penetrating more rapidly than hydrochloric, phosphoric, or sulfuric acids.10 Corneal tissue has an inherent buffering capacity that tends to equilibrate local pH to physiological levels, but severe chemical injuries exhaust the cellular and extracellular resources, allowing extremes of pH that are incompatible with tissue survival.11
Hydrofluoric acid is a strong inorganic acid (used in industry for cleaning and etching) that is particularly toxic because it has a complex mode of tissue injury. Along with necrosis from a high concentration of hydrogen ions, this agent causes cellular death because the fluoride anion binds calcium more quickly than the body can mobilize calcium from bone to equilibrate the loss.13 In addition, the fluoride blocks the Na-K ATPase enzyme of cell membranes, resulting in a fatal loss of potassium from the cells.14 The corneal damage sustained from exposure to hydrofluoric acid worsens with increasing concentration; it can be as mild as transient edema or as severe as immediate opacification or early perforation.10
Pathophysiology of Chemical Injuries
Role of Corneal and Conjunctival Epithelium
The corneal and conjunctival epithelia merge at the limbus, where the subtle transition from the nonkeratinized, stratified squamous epithelium of the cornea to the nonkeratinized, stratified columnar epithelium of the conjunctiva occurs. Together, these two epithelial surfaces cover the exposed portions of the eye, and function as a barrier to chemical insults and to invasion of micro-organisms.
Corneal Epithelium
The corneal epithelium serves the unique function of providing a smooth optical surface and the transparency necessary to transmit images with minimal distortion. The chemically injured corneal epithelium may desquamate, or it may become irregular and lose its clarity. When the integrity of the epithelium is compromised, exposure of the underlying stroma may result in an alteration of hydration that further compromises corneal transparency.
The epithelial cell membranes are composed of lipid, which renders these cells hydrophobic. Adjacent cells are linked by secure junctional complexes, which impede the penetration of caustic substances through the epithelium. Strong alkalies pass rapidly through this relative barrier, however, because they saponify and liquefy the lipoidal cell membranes and junctional complexes.
Epithelial cells can be vigorous contributors to various phases of the corneal immune response.15,16 Intermingled with corneal epithelial cells are sparse Langerhans cells,17,18 which appear during local or remote corneal inflammation19 and which also participate in the corneal immune reaction.20 Epithelial cells secrete cytokines, including those that can inhibit the production of type I collagenase (capable of digesting corneal stroma) by underlying keratocytes.21 Curiously, epithelial cytokines can also stimulate the same keratocytes to produce type I collagenase.22 In stromal ulcerative disorders, for example, after severe chemical injuries, regulatory participation of regenerating epithelium can help tip the balance toward either further stromal degradation or re-establishment of corneal integrity. Epithelial cells themselves can produce a collagenase, although it is type V collagenase (gelatinase), which digests a substrate of denatured collagen (Fig. 28.2).23 Epithelial cells can also release prostaglandins in response to inflammation.15
Source of Regenerating Corneal Epithelium
The undisturbed corneal epithelium consists of approximately five layers of cells overlying the basal epithelial cells, which are affixed to their underlying basement membrane (Bowman’s layer) through a group of adhesional components, primarily the hemidesmosomal anchoring plaques. The presence of an intact extracellular matrix, with fibronectin, laminin, glycosaminoglycans, and collagen is essential for secure binding of the basal epithelial cells.24
Epithelial cells arising from multipotential stem cells at the corneoscleral limbus migrate continuously in a centripetal fashion toward the corneal center. They replace the epithelial cells that have moved toward the surface during their normal maturation and have desquamated from the cornea.25 In the uninjured cornea, complete replacement of epithelial cells occurs every 5 to 7 days.26 The rate at which migrating cells move over the corneal surface increases after a traumatic loss of corneal cells,27 for example, after chemical injuries resulting in epithelial desquamation. In the ideal situation, sheets of advancing corneal epithelium impinge on the epithelial defect in convex waves that eventually meet in healing ridges resembling the arms of a “Y.” These ridges later disappear as the healing cells readjust their positions to restore the normal contour of the injured cornea.
Within the first few hours after corneal epithelial injury, the surviving margin of intact epithelium sends fingerlike extensions forward into the injured zone. Fibronectin and other proteins from the tear film are deposited on the bare stroma or intact Bowman’s layer. By the sixth hour, basal epithelial cells from the margin of the wound have lost their hemidesmosomal attachments and have become mobile. They migrate centripetally into the denuded zone, dragging with them one or two overlying layers of epithelial cells. At first, individual cells become thin, increasing their surface area to facilitate migration over the defect. Later, their numbers increase as mitosis occurs a few millimeters behind the advancing edge. Only after the defect is completely closed do the healing epithelial cells establish secure attachments to the underlying basement membrane and extracellular matrix. Next, they synthesize the proteins and intercellular bridges that render an intact epithelial surface resistant to penetration by infectious agents and noxious chemicals.26,27,28
Conjunctival Epithelium
The conjunctiva is a vascularized mucous membrane that lines the entire exposed surface of the eye posterior to the cornea. It is reflected through the conjunctival fornices onto the posterior aspect of the lids. Its epithelium provides a moist, smooth surface over which the lids can pass. It participates with the corneal epithelium in establishing a relative barrier to the passage of micro-organisms and noxious chemical agents, and it is active in local immune reactions.29 Its goblet cells produce mucin, which adsorbs to the glycoproteins coating the microvilli of corneal and conjunctival epithelial cells. This precorneal and preconjunctival mucin layer merges gradually with the overlying aqueous tear film to ensure complete wetting of the ocular surface.
The conjunctival epithelium provides a source of cells to repopulate the corneal surface when the entire corneal epithelium has been denuded and the limbal stem cells have been destroyed, as in severe chemical injuries.30,31 After complete re-epithelialization of the cornea with conjunctival epithelium, a phenotypic change in the structure of these cells takes place by the process of transdifferentiation.32 Gradually, the new epithelial surface begins to resemble that of corneal epithelium. The pseudostratified, columnar conjunctival epithelium becomes pseudostratified squamous, and there is an attrition of goblet cells. After several weeks, the biochemical functions of the healing cells begin to more closely resemble those of corneal epithelium. After transdifferentiation in cases of severe chemical injury, however, vascularization of the healing tissue inevitably occurs, with a return of goblet cells and conjunctivalization of the corneal surface.33
Role of Corneal Stroma
Ninety percent of the cornea is stroma, consisting of approximately 200 layers of mostly type I collagen.34 Resting on the most superficial portion of the stroma is Bowman’s layer, a meshwork of filaments derived from collagen types I, III, V, VI, and possibly IV.35 The stroma itself is relatively acellular, with only 2% occupied by keratocytes, which are the corneal equivalent of fibroblasts. These keratocytes produce collagen, which accounts for >70% of the stroma by weight. The keratocytes secrete a procollagen triple helix, from which collagen molecules form, into the extracellular space. These coalesce into fibrils, which in turn assemble into collagen fibers. Collagen fibers are uniform in diameter, with a constant spacing, factors that favor the transparency of corneal stroma.36
The average diameter of collagen fibers and the average separation between adjacent fibers each measures less than half the wavelength of visible light (the shortest visible wavelength of violet light is 380 nanometers and the longest visible wavelength of red light is about 740 nanometers). This physical property allows a ray of light to pass through the stroma without significant scattering. When there is stromal edema, however, the mean separation between some adjacent fibers increases to greater than half the wavelength of visible light, causing interference in the transmission of light and loss of transparency.37
Keratocytes also synthesize glycosaminoglycans, which help regulate water metabolism in the stroma and maintain the transparency of the stroma. Most of the water in the intercellular stroma is bound to glycosaminoglycan molecules, which maintain uniformity of collagen fibril spacing and the cornea’s transparency.36
Keratocytes also fabricate matrix metalloproteinases (MMPs),38 otherwise known collectively as matrixins or collagenases. These proteases regulate the synthesis and degradation of extracellular matrices, and participate vigorously in corneal wound healing. The MMPs consist of more than 20 types,39 of which MMP-1 and MMP-8 are true collagenases, MMP-2 and MMP-9 are gelatinases (which use a substrate of degraded collagen), and the remaining varieties (MMPs 3, 7, 10, 11, and 12) are stromelysins.38 MMPs are critical components of the process by which injured extracellular matrix is digested and later reformed. MMPs are regulated in vivo by tissue inhibitors of metalloproteinases (TIMPs) and other inhibitors.38 Imbalance between MMPs and TIMPs can lead to excessive fibrosis (when the inhibitor exceeds the enzyme) or to excessive tissue melting (when the enzyme exceeds the inhibitor).38 The major proteinase inhibitors of the extracellular matrix are alpha 2-macroglobulin,38 which also constitutes most of the anticollagenase activity in plasma,40 and the TIMPs.
Among the other participants in corneal wound healing are the various growth factors, including epidermal growth factor (EGF) and transforming growth factors (TGFs) alpha and beta. These are mitogens and chemotactic agents.41 In cornea, there is a constant interaction between epithelium and stroma in the repair of stromal injury.42 For example, TGF beta-2 from epithelial cells in culture inhibits collagenase synthesis by cultured corneal stromal cells; other epithelial cytokines stimulate stromal keratocytes to produce collagenase.42
After a chemical burn or similar injury, keratocytes increase in number by mitosis, and new ones migrate into the region of damage. Migration of keratocytes occurs only under an intact epithelium.35 EGF and fibroblast growth factors both help regulate the influx of new keratocytes.43 The energized keratocytes produce new collagen and proteoglycans.35 Although the new collagen is type I, the diameter of the resulting fibers is larger and the spacing is irregular,44 decreasing transmission of light through the resulting scar. Meanwhile, the new proteoglycans bind water more avidly, resulting in excess hydration of the scar, which further ensures irregular spacing (with lack of transparency) of the new collagen.35 In addition, stromal keratocytes develop intracytoplasmic contractile elements that cause contraction of the scar and irregular astigmatism.35
In the chemically injured cornea, these complex mechanisms by which the stroma responds typically lead to minimal scarring and loss of transparency when an overlying epithelial defect heals rapidly and the damage to the stroma is superficial. In injuries of great severity, with long chemical contact time and maximal variances from physiological pH, the overwhelming stromal response is that of degradation or melting. These are situations in which the intrinsic control mechanisms fail to maintain homeostasis.
Role of Polymorphonuclear Neutrophil
Collagenase activity has been documented in polymorphonuclear neutrophils (PMNs).45 Before they are released from the bone marrow, PMNs synthesize proteases, including gelatinase B and collagenase 2.46,47 Matsuda and Smelser observed numerous PMNs at sites of stromal ulceration after ocular chemical injuries.48 Pfister et al. observed that after alkali injury of the cornea, the tripeptide degradation products of stromal collagen are among the earliest biochemical factors stimulating PMN influx into the cornea.49,50 The gathering PMNs themselves release leukotrienes, which are chemotactic agents for the additional influx of neutrophils.51 The alkali-injured collagen also liberates a cytokine that triggers a respiratory burst among the accumulating PMNs. These cells release not only proteolytic enzymes, but also superoxide radicals, both of which aid in further collagen degradation and resultant corneal ulceration.51
After burns with sodium hydroxide, Kenyon et al. demonstrated that in rabbit eyes, when both epithelium and PMNs were excluded from the exposed stroma by gluing a contact lens in place 2 days after the burn, only 10% of corneas ulcerated.52 In the control eyes without corneal protection, ulceration occurred in 2 weeks. If the lenses were removed at day 14, 80% of corneas ulcerated within the next week. When rings (exposing the corneas centrally) were glued onto the denuded stroma of 15 burned eyes, epithelium was excluded, but PMNs migrated to the exposed central zone through the tear film. Eleven of the corneas developed ulcerations, with PMNs adhering to the digested tissue. In non–alkali-burned controls, with or without glued-on contact lenses or rings, no ulcerations occurred despite the presence of many PMNs.52
Evidence suggests that 10% sodium citrate applied hourly to the alkali-burned eye may offer some relative protection against stromal ulceration53 by inhibition of selective PMN activities. The PMNs must adhere to the endothelium of vessels before their movement through the vascular wall and chemotaxis can cause a tissue infiltrate. Citrate inhibition of PMNs may be through a mechanism that keeps them circulating and prevents them from adhering to the endothelium.54
Prevention of Corneal Ulceration
Inhibitors of Collagenase
Endogenous inhibitors of collagenase and related proteinases participate vigorously in embryogenesis and tissue maturation, and in the remodeling of connective tissues that occurs during wound healing, bone resorption, and various types of periodontal disease.40,55 TIMPs located in proximity to connective tissue cells are important regulators of the status of the extracellular matrix. Humoral inhibitors, primarily alpha 2-macroglobulin, control the activity of metalloproteinases in body fluids.40 Binding of alpha 2-macroglobulin to MMPs is extremely tenacious, rendering this circulating agent one of the strongest known inhibitors of MMPs.40 Berman et al. used alpha 1-antitrypsin, another anticollagenolytic factor derived from human serum, in an effort to inhibit corneal ulceration.56
The search for safe, effective exogenous inhibitors of collagenase progressed during most of the 1960s. Eisen et al. used cysteine to prevent collagenolytic activity in human skin.57 Both the disodium and calcium derivatives of ethylenediaminetetraacetic acid (EDTA) inhibited lysis in concentrations as low as 10-4 M. The calcium EDTA appeared safer because it did not inhibit cell growth in tissue culture in concentrations as high as 10-2 M.58,59
Hook et al. believed that EDTA chelated the calcium necessary for collagenase activity. Because calcium is replaced by tissue fluids, EDTA would have to be used almost continuously to maintain anticollagenolytic activity. Cysteine theoretically could be used less frequently because it has a double action: Not only does it chelate calcium, but it also irreversibly reduces a critical disulfide bond in the collagenase molecule.60
Slansky et al. reported that along with 0.2 M l-cysteine and EDTA, 1.2 M N-acetyl-l-cysteine was also effective in vivo against collagenase and was more stable than cysteine. Initial trials on ulcerating rabbit corneas were performed with N-acetylcysteine (Mucomyst), as a 10% or 20% solution. Later, the studies were reconfirmed with a pure acetylcysteine solution when it was determined that Mucomyst contains 10-3 M EDTA (itself a known collagenase inhibitor) as a stabilizer. When treatment with this agent was stopped on day 21, four of six eyes ulcerated within 48 hours, but none of the eyes still receiving the drug ulcerated.61
Tetracycline
Tetracyclines are a family of broad-spectrum antibiotics that exhibit anti-inflammatory and anticollagenolytic activity independent of their antimicrobial properties.62,63,64 Windsor et al. reported that after subjects received oral tetracyclines for 4 days, white blood cell phagocytic activity diminished, and their ascorbic acid levels decreased by at least 50%.62 Forsgren et al. demonstrated both in vitro and in vivo that in the presence of tetracycline or doxycycline, human PMNs displayed a marked decrease in their phagocytosis.63 In 1985, Perry and Golub described the healing of a noninfected corneal ulcer after the patient began taking 250 mg oral tetracycline four times a day.65
The inhibition of MMPs in periodontal disease and reactive arthritis, inflammatory conditions resembling ocular collagenolytic disorders, has been demonstrated using tetracycline, chemically modified tetracycline (without antibiotic properties), doxycycline, and minocycline.66,67,68
Numerous studies have documented the benefits of topical and systemic tetracycline in the management of ocular chemical injuries. In alkali-burned rabbit eyes treated with topical 1% or 5% tetracycline hourly for 12 hours a day, 54% of the control eyes ulcerated, but only 8% of the treated eyes ulcerated.69 Intramuscular tetracycline also inhibited corneal ulcerations in alkali-burned rabbit eyes. Eyes that ulcerated showed inflammatory infiltrates with PMNs, but there were no inflammatory cells in nonulcerating eyes.70 When oral tetracycline was added to the therapy of 18 patients with nonhealing corneal epithelial defects, the erosions of 14 patients healed.71
Although tetracycline, doxycycline, and minocycline can inhibit mammalian collagenases, antibiotics such as penicillin and cefazolin are ineffective. Collagenases and the other MMPs can function only in the presence of divalent calcium and zinc cations. When these ions are chelated, the activity of collagenase decreases. Tetracycline binds to collagenase by a calcium bridge, inactivating the enzyme unless additional calcium is added.71 Tetracycline also appears to decrease collagenase activity by altering the gene expression responsible for synthesis of the MMPs.72 Tetracycline has both direct and indirect effects on PMNs. It decreases their ascorbic acid levels,62 and it reduces PMN infiltrates in injured corneal stroma by decreasing collagen lysis, the products of which are chemotactic for PMNs.70
Finally, tetracycline has a beneficial effect on levels of alpha-1-antitrypsin, one of the two (the other is alpha-2-macroglobulin) most significant humoral inhibitors of MMPs. Alpha-1-antitrypsin, otherwise known as serpin, inhibits serine proteases such as neutrophil elastase and thus protects matrixins from proteolysis. However, serpin itself is readily digested by neutrophil collagenase. When doxycycline is added to an in vitro mixture of neutrophil collagenase and serpin, this degradation of alpha-1-antitrypsin is significantly reduced, and the digestion of matrixins is curtailed.73,74,75
Synthetic Inhibitors of Collagenase
Among the many synthetic inhibitors of collagenase is the hydroxymate-containing dipeptide, Galardin.76 This agent appears to prevent the corneal ulceration of alkali injury by diminishing the release from PMNs of MMPs that digest capillary walls to allow extravasation of the PMNs.77 Any decrease in the number of locally extravasated PMNs decreases the potential pool of MMPs at the site of injury. Galardin further reduces inflammation by preventing release of tumor necrosis factor, a cytokine that activates PMNs, from producer cells such as macrophages and activated T cells.25 Galardin may also block MMPs released from inflammatory cells, corneal fibroblasts, and epithelial cells.25
Other synthetic inhibitors of MMPs include mercaptan (thiol)-containing compounds resembling the drug captopril, which inhibits the metalloproteinase angiotensin-converting enzyme in the treatment of heart failure and hypertension.78 One of the MMP inhibitors derived in this manner, HSCH2CH[CH2CH(CH3)2]CO-Phe-Ala-NH2 (SIMP), is effective in inhibiting corneal ulceration in the alkali-burned rabbit eye when the therapy is initiated shortly after onset of ulceration.79,80 Although the goal is to discover new effective inhibitors of collagenase, development of these agents has been hampered by their toxicity.81
Role of Steroids
Koob et al. reported in 1974 that dexamethasone inhibited collagenase production in tissue cultures of human skin,82 but it also predisposed to perforation the alkali-burned rabbit cornea, possibly by decreasing collagen synthesis.83 François and Feher demonstrated that topical corticosteroids retarded the fibroblastic repopulation of acellular stroma,84 and that corticosteroids given during the second and third weeks after an alkali burn enhanced the severity of ulceration.85 This enhancement of ulceration appeared to result from an inhibition of repair processes rather than an increase in collagen lysis. Thus, the suppression of collagen repair in a severely burned cornea could result in perforation even when collagenase production was simultaneously reduced.
Jeffrey et al.86 and Halme et al.87 observed that progesterone blocked collagenase formation in cultures of postpartum rat and rabbit uterus. In 1977, Newsome and Gross reported that in alkali-burned rabbit corneas, topical, intramuscular, or subconjunctival application of medroxyprogesterone was effective in preventing corneal ulceration.88 Because medroxyprogesterone is not known to be an inhibitor of collagenase itself, the data from these rabbit trials were consistent with a blockage of collagenase production. In 1981, however, Lass et al. were not successful in reducing ulceration using topical or subconjunctival medroxyprogesterone in alkali-burned rabbit eyes.89
Roles of Ascorbate and Citrate
Both ascorbate and citrate have been studied extensively as possible therapeutic modalities in the prevention and treatment of corneal ulceration after ocular chemical injury.
Experimental alkali burns of rabbit eyes release ascorbic acid from injured tissues,90 suggesting that a possible therapeutic approach might include supplementation with ascorbic acid. Ascorbic acid is required for hydroxylation of the proline and lysine used by fibroblasts in the synthesis of a collagen peptide chain. When hydroxylation is imperfect, as in ascorbic acid deficiency, the result is the formation of unstable collagen molecules vulnerable to proteolytic enzymes.91 Because ascorbic acid is closely involved in the biosynthesis and maintenance of collagen,92 it might prove useful in the treatment or prevention of corneal ulceration in corneal chemical injuries. Ascorbic acid is not an inhibitor of collagenase, however, because collagenolytic activity in skin wound healing is not affected by altered ascorbic acid levels.93
Ascorbic acid levels in aqueous may be an indicator of alkali damage to the ciliary body94 because ascorbic acid is actively transported by the ciliary body into the aqueous. After severe ocular chemical burns in rabbits, aqueous ascorbic acid concentrations drop markedly, remaining low in eyes that ulcerate. When the aqueous ascorbic acid level is artificially maintained at a level >15 mg/dL, corneal ulceration can be prevented or significantly reduced.95 The large demand for the small amount of ascorbic acid in the aqueous after alkali burning helps explain corneal ulcers and perforation on the basis of insufficient collagen production while the denatured collagen is being resorbed.95,96
Citrate is effective in decreasing corneal ulceration after alkali burns of rabbit eyes.53 It inhibits the collagenolysis promoted by PMNs by chelating the extracellular calcium required for PMN activities and by preventing the PMNs from adhering to the endothelium of vessels near the injured site. PMNs must adhere to endothelium before their movement through a vascular wall can cause a tissue infiltrate.54
Classification of Chemical Burns
Hughes originally classified ocular chemical burns according to their clinical findings during the acute phase97,98:
Mild
Erosion of corneal epithelium
Faint haziness of cornea
No ischemic necrosis of conjunctiva or sclera
Moderately severe
Corneal opacity blurring iris details
Minimal ischemic necrosis of conjunctiva and sclera
Very severe
Blurring of pupillary outline
Blanching of conjunctival and scleral vessels
Ballen,99 and later Roper-Hall,100 revised the Hughes classification, expanding it to include four stages. They emphasized the importance of limbal hyperemia and blanching in determining a prognosis.
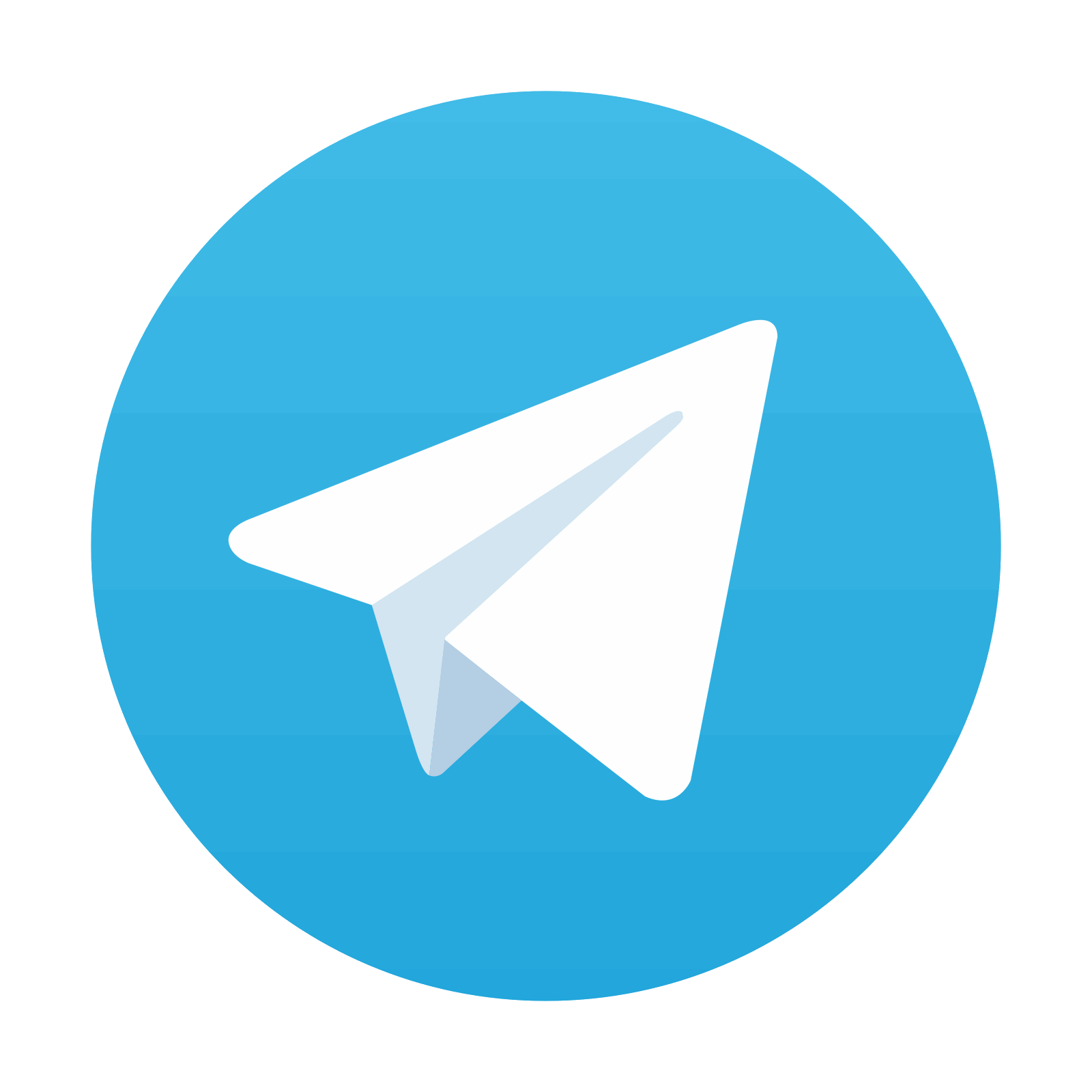
Stay updated, free articles. Join our Telegram channel

Full access? Get Clinical Tree
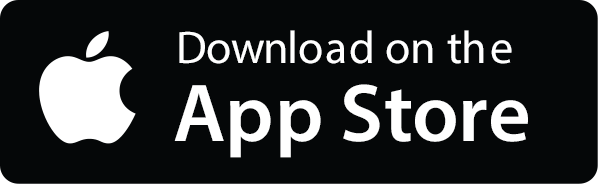
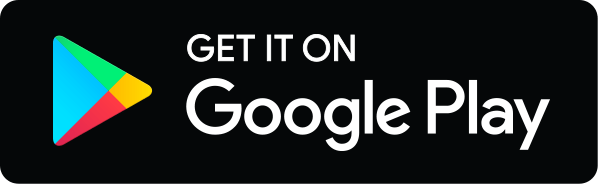