Fig. 11.1
Example of a football helmet with increased protection around base of jaw, making airway maneuvers such as chin lift more difficult
The athlete who returns to the sidelines complaining of neck pain requires careful assessment. Generally, any athlete with restricted or painful cervical motion, bony tenderness, or any motor sensory deficit that involves more than a single upper extremity and does not quickly resolve should be removed from competition, placed on spinal precautions, and referred for further evaluation. The athlete who complains of symptoms consistent with a stinger, unilateral upper extremity burning pain and/or weakness typically in a C5–C6 distribution, should be removed from competition until all symptoms have resolved and there is normal and painless cervical range of motion and strength.
Imaging
Imaging of the young athlete with suspected cervical spine injury typically begins with plain radiographs including AP, lateral, and open-mouth odontoid views. Interpreting cervical spine radiographs in children can be challenging due to the developing anatomy where pseudo-subluxation of C2 on C3 and occasionally C3 on C4 can occur (see Fig. 11.2). In one retrospective review of 138 pediatric trauma patients, a 22 % incidence of pseudo-subluxation of C2 on C3 was found [8]. One way to differentiate pseudo-subluxation from true injury is to assess the spinolaminar (Swischuk’s) line on the lateral c-spine X-ray. In cases of pseudo-subluxation, the spinolaminar line should pass within 1 mm of the anterior cortex of the posterior arch of C2 (see Fig. 11.3). When this line passes >1.5 mm from the anterior cortex of the posterior arch of C2, acute injury is likely (see Fig. 11.4). The atlantodens interval , the distance from the anterior aspect of the dens to the posterior aspect of the anterior ring of the atlas, can show more variation in children than adults. The atlantodens interval in adults is usually ≤3 mm; however, in children <8 years of age, an atlantodens interval of 3–5 mm is seen in about 20 % of patients [9]. Understanding normal physes is another challenge unique to children. Unfused C1 ring apophyses, apical odontoid epiphysis, and secondary centers of ossification of the spinous processes can all be mistaken for fractures. Generally normal physes are smooth structures with sclerotic subchondral lines, whereas fractures are more irregular and lack sclerotic lines. In children under age 8 years, anterior wedging of the vertebral bodies up to 3 mm is within normal limits. Wedging can be most pronounced at C3 due to hypermobility of the pediatric cervical spine with increased motion especially at C2–C3.
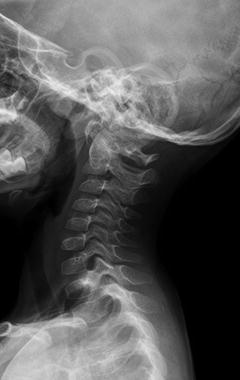
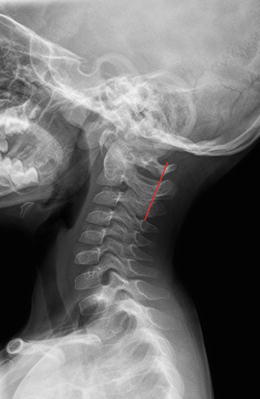
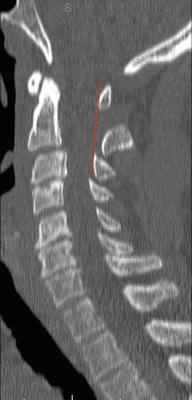
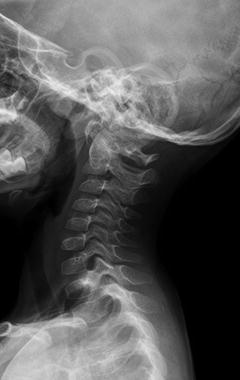
Fig. 11.2
Lateral cervical spine radiograph demonstrating mild pseudo-subluxation of C2 on C3
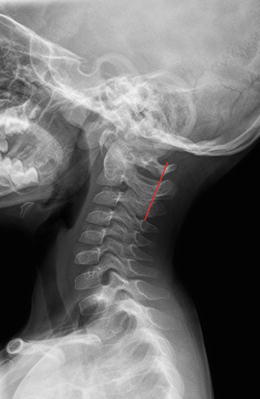
Fig. 11.3
Same X-ray as seen in Fig. 11.2. with Swischuk’s (spinolaminar) line showing normal alignment
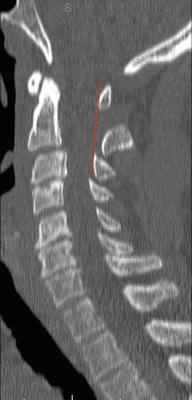
Fig. 11.4
Sagittal image of CT scan showing abnormal spinolaminar line representing injury at C2–C3
Although multiple reports have recommended cervical spine CT scan as the preferred screening tool for adult trauma patients, demonstrating quicker time to diagnosis and a shorter stay in the trauma resuscitation area compared to plain films, there remains some question with pediatric patients [10–15]. There is concern, especially in pediatric patients, regarding the radiation dose from CT scanning. A missed cervical spine injury, however, can have lifelong devastating consequences, and therefore CT scan may be used when clinical suspicion for injury is high. A recent review of 1307 pediatric trauma patients compared CT scan to plain X-rays in diagnosing cervical spine fractures [10]. The study found CT scan had a sensitivity of 100 % and a specificity of 98 %, while X-rays had a sensitivity of 62 %. The authors concluded that “CT scans should be the primary modality to image a cervical spine injury.” The study also looked at flexion/extension views , and the authors stated that “flexion/extension views did not add to the decision making for C-spine clearance after CT evaluation and are probably not needed” [10]. CT scan is likely most effective in older children and adolescents, where injury patterns are similar to adults. Younger children, however, are more prone to purely ligamentous or soft tissue injury, which may not be appreciated on CT scan. In these patients magnetic resonance imaging (MRI) may be the modality of choice to identify injury. One study of MRI in 64 pediatric cervical spine patients found MRI demonstrated injury in 24 % of patients where X-rays were normal and allowed for spine clearance in three children where CT scan was equivocal [16].
The question of radiation exposure from CT scan in pediatric patients merits consideration. One study prospectively examined radiation exposure in pediatric patients undergoing CT versus conventional radiographs [17]. The authors found a 1.25 higher effective radiation dose with CT scan. Another study comparing CT scan to X-rays found a higher radiation dose with CT for patients with a Glasgow Coma Scale >8, but for those with a GCS <8 the doses were equivalent due to the higher need for repeated radiographs [15]. Although CT scans produce more radiation than plain radiographs, there are ways to shield patients, and by following these protocols, CT exposure can be reduced by 30–50 % in pediatric patients compared to adults with no loss in the quality of images [18, 19]. For most pediatric patients with low suspicion for cervical spine injury, however, three view plain radiographs have been shown to suffice and should constitute the initial radiographic evaluation [20].
Fractures
Cervical spine fractures are relatively rare in athletic events, especially in younger children. Most pediatric cervical fractures result from motor vehicle accidents or falls. In children 8 or less, it is very uncommon to see a subaxial fracture from athletics. The atlantoaxial complex is most at risk in younger children, with the majority of serious injuries involving a ligamentous disruption rather than fracture. Axial compression with extension can potentially cause a fracture of the ring of C1, but the forces required to do so are rarely seen in youth sports. Similarly, odontoid fractures can occur, usually from a rapid deceleration with flexion mechanism, but the forces in youth sports rarely are high enough to cause such injury. When fractures of the odontoid occur, they tend to happen through the synchondrosis of C2 at the base of the odontoid. These fractures tend to displace anteriorly, and reduction can usually be accomplished through immobilization of the cervical spine in extension. In adolescents the cervical anatomy approaches that of adults, and subaxial fractures are occasionally seen with the most common being a compression fracture. More severe burst patterns can also occur. When these compression/burst injuries happen, they tend to occur between C5 and C7, a result of the increased forces on this portion of the spine as the anatomy matures.
The incidence and pattern of cervical spine fracture vary by sport. In the United States, football accounts for the highest number of catastrophic cervical spine injuries due in part to the annual participation in the sport of approximately 1.8 million athletes [21]. The incidence of cervical injury per 100,000 players is actually higher in gymnastics and hockey. The rate of cervical spine injury in football has changed over time. From 1971 to 1975, the National Football Head and Neck Injury Registry recorded 259 cases of cervical fractures (4.14/100,000) and 99 cases of quadriplegia (1.58/100,000) [22]. In 1976 rule changes banning headfirst contact were implemented by the National Collegiate Athletic Association and high school football governing bodies. From 1976 to 1987, the rate of cervical injuries decreased almost 70 % at the high school level from 7.72/100,000 to 2.31/100,000. The rate of quadriplegia decreased 82 %. Over the 25 years, from 1977 to 2001, 223 football players sustained a catastrophic cervical spine injury with either no or incomplete recovery with 183 of those occurring in high school athletes, 29 in college, seven professional, and four recreational players [23].
Understanding the mechanism resulting in cervical spine fracture is important in an effort to reduce the incidence. In football it has been recognized for some time that the headfirst tackle with the neck flexed is a vulnerable position that can lead to axial loading of a straightened spine. When the spine is extended or in neutral position, compressive forces can often be dissipated through the paravertebral musculature and ligaments . When the same force is applied to a straightened spine, the energy is primarily transferred to the vertebrae, and buckling or fracture dislocation of the spine can occur. Most of these fractures occur in the lower cervical spine. As football helmets and face masks evolved in the 1960s, the head was able to be used as a weapon in tackling, and this change likely contributed to the increased rate of cervical spine fractures in the early 1970s. In ice hockey, the rate of catastrophic cervical spine injury was relatively low prior to 1980. From 1982 to 1993, ice hockey saw an increasing rate of cervical injury, again likely related to increased head and face protection making the neck more vulnerable [21]. In ice hockey a common mechanism of cervical spine fracture is headfirst contact into the boards, often when a player has been checked from behind. Rule changes were enacted prohibiting checking from behind a player who no longer has the puck. Data from the Canadian registry suggest that following those changes, major spinal injury and quadriplegia resulting from these illegal techniques decreased [24].
Neuropraxias
Neuropraxias are more common than fractures in youth sports. A “stinger” or “burner injury” is the most common neurologic injury and involves a temporary burning sensation and/or weakness in a single upper extremity. Collision sports such as football and rugby pose the highest risk for stingers. In younger athletes a stinger most commonly results from a forced stretching of the head and neck away from the involved limb resulting in traction to the brachial plexus with the C5 and C6 roots most commonly affected. In older adolescents and adults, the stinger more commonly results from a forced compression of the head and neck toward the involved limb. The shoulder may simultaneously be forced upward, causing a momentary narrowing of the cervical foramen and resulting in a pinching or compression of the nerve root and transient radiculopathy . Kelly et al. have shown that some children have congenital narrowing of the cervical foramen, placing them at increased risk of sustaining a burner [25]. Athletes who have sustained a stinger typically report immediate burning and weakness in the involved extremity and report a “dead arm” sensation . Both sensory and motor function typically return to normal within seconds to minutes with full recovery by 10 min the rule. With repetitive injury permanent damage can occur, and rarely with a severe cervical pinch mechanism, a nerve root(s) can be severed.
Cervical cord neuropraxias are important to differentiate from stingers. Stingers are limited to a single upper extremity, whereas cervical cord neuropraxias typically present with transient quadriplegia and either loss of sensation in all four extremities or a burning or tingling sensation. Motor function usually recovers within minutes, but sensory changes can last longer. Athletes with a congenital narrowing or stenosis are thought to be at increased risk of cervical cord neuropraxias. Although the determination of cervical stenosis is an area of some controversy, general consensus holds that between C3 and C7 the anteroposterior (AP) spinal canal heights in adolescents and adults are normal above 15 mm and stenotic below 13 mm. Resnick et al. have stated that CT and myelography are more sensitive than plain X-rays in determining spinal stenosis [26]. They note that X-rays fail to appraise the width of the spinal cord and cannot detect when stenosis results from ligamentous hypertrophy or disc protrusion. Ladd and Scranton state that the AP diameter of the spinal canal is “unimportant” if there is total impedance of the contrast medium [27]. For all these reasons, spinal stenosis cannot be ruled out based only on bony measurements. “Functional” spinal stenosis , defined as the loss of the cerebrospinal fluid around the cord or in more extreme cases deformation of the spinal cord, whether documented by contrast CT, myelography, or MRI, is a more accurate measure of stenosis [28]. The term functional is taken from the radiographic term “functional reserve” as applied to the protective cushion of cerebrospinal fluid (CSF) around the spinal cord in a normal spinal canal.
Cervical spinal stenosis in the athlete may be a congenital/developmental condition or may be caused by acquired degenerative changes in the spine. For the athlete with severe stenosis and no CSF around the spinal cord on MRI, it is this author’s opinion that collision sports should be avoided. The athlete with spinal stenosis is at risk for neurologic injury during hyperextension of the cervical spine [29]. When the neck is hyperextended, the sagittal diameter of the spinal canal is further compromised by as much as 30 % by infolding of the interlaminar ligaments. Matsuura et al. studied 42 athletes who sustained spinal cord injury and compared them to 100 controls [30]. They found that “the sagittal diameter of the spinal canals of the control group was significantly larger than those of the spinal cord injured group.” Eismont et al. have stated that “the sagittal diameter of the spinal canal in some individuals may be inherently smaller than normal, and … this reduced size may be a predisposing risk factor for spinal cord injury” [29]. The idea that spinal stenosis predisposes to spinal cord injury is not new, with multiple authors as far back as the 1950s reaching the same conclusion including Wolfe et al. [31], Penning [32], Alexander et al. [33], Mayfield [34], Nugent [35], and Keenan et al. [15] who stated that “patients who have stenosis of the cervical spine should be advised to discontinue participation in contact sports.” More recent support for this stand comes from the National Center for Catastrophic Sports Injury Research , where cases of quadriplegia have been seen in athletes with cervical stenosis but without fracture or dislocation. In athletes with a normal-size canal, quadriplegia has not been seen without fracture/dislocation of the spine. And most importantly, full neurologic recovery has been observed in 21 % of athletes who were rendered initially quadriplegic after fracture/dislocation with normal-size cervical canals, while complete neurologic recovery has not been seen in any athlete after fracture/dislocation and quadriplegia when spinal stenosis was documented by MRI.
< div class='tao-gold-member'>
Only gold members can continue reading. Log In or Register a > to continue
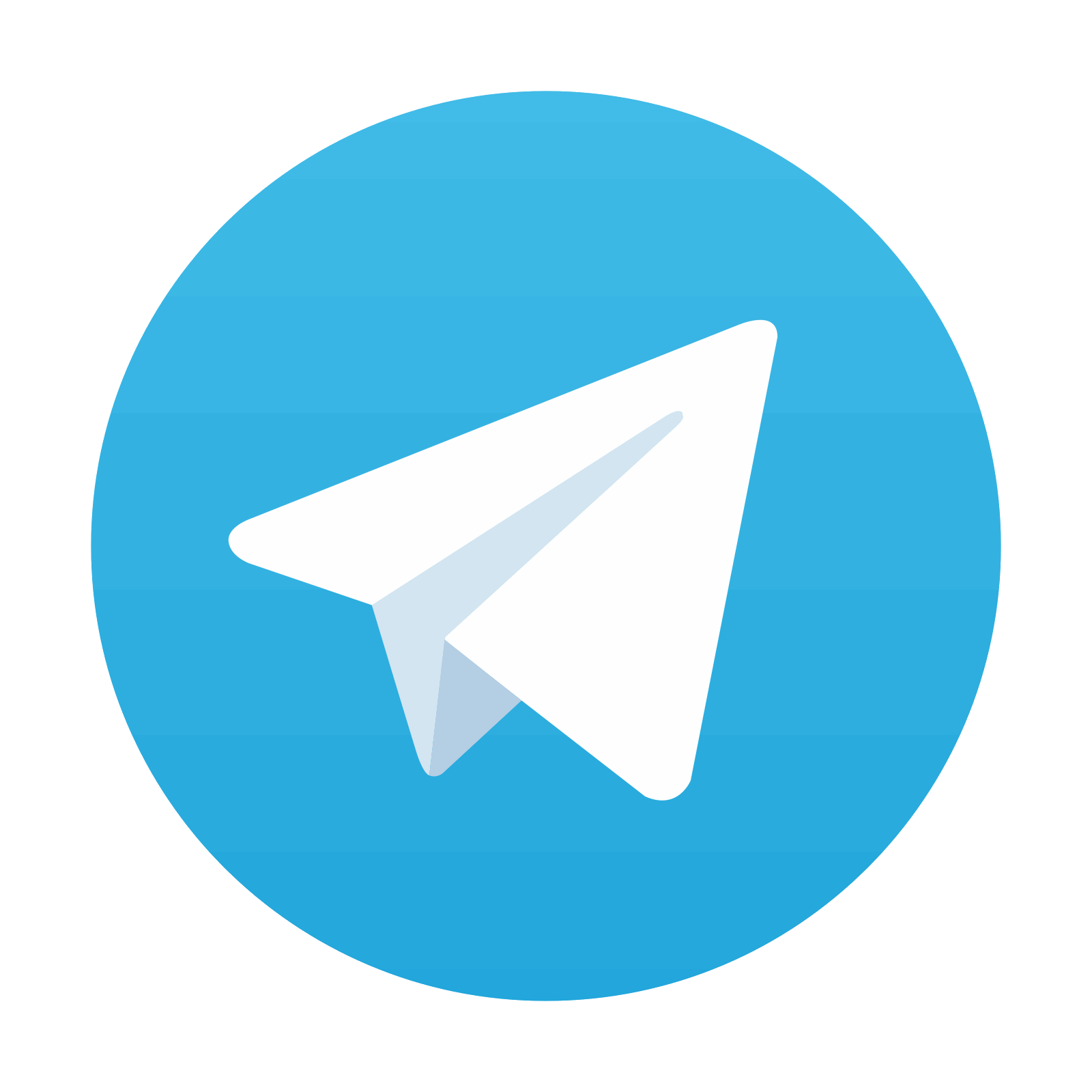
Stay updated, free articles. Join our Telegram channel

Full access? Get Clinical Tree
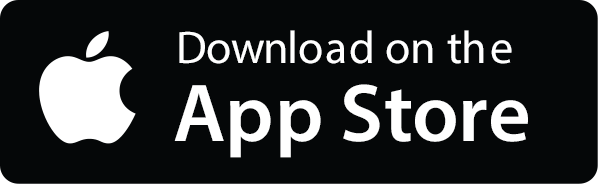
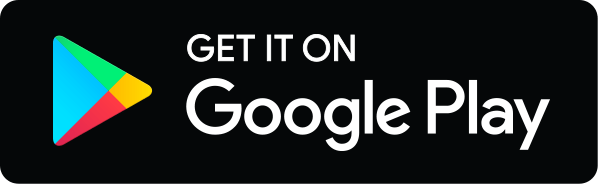