Fig. 7.1
(a, top left) A traumatic diastatic fracture involving the sagittal suture and left coronal suture in a 53-year-old male after a fall. (b, top right) A depressed left frontal skull fracture in a 9-year-old following a golf club strike to the head; note the subcutaneous and intracranial air indicating the open nature of the injury. (c, bottom left) A linear fracture of the occipital bone in a 22-year-old following a motor vehicle-related injury. (d, bottom right) An image taken from the same scan and same patient as in (c), showing that the fracture has tracked inferiorly and has comminuted involvement of the skull base
Epidemiology and Etiology
The epidemiology of traumatic brain injury (TBI ) as a whole is limited by a number of factors, including non-standardized definitions, underreporting of cases (in particular from patients who may not seek medical attention), differences in specific population focus across studies, and, until relatively recently, lack of coordinated efforts to monitor epidemiologic data on a broad scale. Despite this, there is a greater appreciation that sports are a major contributor to the incidence of structural injuries, especially in the young. A retrospective review of the National Hospital Ambulatory Medical Care Survey revealed that 14 % of emergency room visits for life-threatening injuries were sports related, with children having a higher percentage compared to adults (32 % vs. 9 %) [65].
Data on skull fractures in particular are clouded by their frequent coincidence with other types of TBI. In studies that examine the incidence of TBI, the separate incidence of overlying skull fractures is not consistently reported. It is important to keep in mind that epidemiology of these lesions remain difficult when interpreting data regarding their incidence in the population.
The incidence of skull fractures across all ages, as measured by presentation to the US emergency departments, has been estimated at 16 cases per 100,000 per year (3.5 % of all those presenting to attention for head injuries) [45]. However, measured incidences show considerable variation, with some researchers reporting an incidence of 66 per 100,000 per year in adults of the United Kingdom and others citing a 6 % incidence of comminuted skull fractures alone among patients presenting with head injury [40, 93]. Children are particularly prone to skull fracture, with some estimating that greater than 20 % of children presenting to attention in the emergency room for head injury have evidence of a skull fracture [36].
As is consistent across almost all types of head injury , skull fractures have a strong predilection for the young male, with an estimated 2:1 to 3:1 male to female ratio [8, 93]. Though few studies explicitly have evaluated skull fractures as a separate entity from other types of TBI, it is presumed that the causes of skull fracture mimic those of head injury overall, in which transportation-related incidents, occupational hazards, falls, and assaults feature prominently [8, 46, 59, 88]. It should be noted that the presence of an overlying skull fracture has been shown to dramatically increase the risk of finding an underlying intracranial pathology in both adults and children, as much as 20-fold in some studies [17, 23, 75, 99].
Most studies do not feature sports-related injuries as a major contributor to measured incidences of skull fractures [5]. The converse is also typically true, and most sports-related head injuries do not feature skull fractures or other structural brain injuries. Nonetheless, skull fractures are not unheard of in sports and are commonly seen in patients with severe injuries that require inpatient admission. As many as 40 % of inpatient admissions for sports-related head injury have evidence of a skull fracture [53, 58]. Common mechanisms for these injuries include high-energy collisions (skiing, football, hockey), impacts from hard, high-velocity projectiles (golf, shooting), or falls from heights (cycling, climbing, horseback-riding) [30, 58]. Interestingly, although head injuries in this sport are extremely rare, some limited evidence suggests that among sports-related head injuries presenting to the emergency department, those that are golf related have an association with skull fractures. This is presumably due to a unique combination of hard, high-velocity objects with small surface areas (golf balls and club heads) and no routine use of protective headgear [58, 76, 96]. Despite some work into identifying skull fractures incidences in individual sports, however, it is important to emphasize that little evidence has yet been gathered on the epidemiology of skull fractures in the overall population of young athletes.
The incidence of most types of traumatic intracranial hemorrhage is lower than that of skull fractures due to the higher necessary kinetic energy to generate these injuries. It is common for patients with traumatic intracranial hemorrhage to have overlying skull fractures, but the converse is not necessarily true. Among patients presenting with TBI, incidence estimates range from 2.7 to 4 % for EDH, approximately 11 % for SDH, and 8.2 % for intraparenchymal hemorrhage [10–12]. The incidence of tSAH is significantly higher than those of other types of intracranial hemorrhage. An estimated 30–60 % of admissions for TBI will feature some type of tSAH present on computed tomography (CT) imaging [26, 52, 62, 69]. As with skull fractures, the mechanisms for intracranial hemorrhage are similar to those for TBI as a whole: transportation-related (most often motor vehicle-related) injuries, falls, occupational hazards, and violence/assault [10–12, 62].
Though sports-related injuries are not known to be a leading cause of these lesions in previously reported literature, the incidence of intracranial hemorrhage in sports-related TBI has not been systematically examined to our knowledge. Sports-related intracranial hematoma formation is not unheard of, however, and they are implicated in the majority of fatalities and catastrophic morbidity across a variety of sports [3, 15, 30, 49, 106]. Brain injuries account for an estimated 69 % of football-related fatalities in the United States, with 75 % of these occurring in high school-level players [15]. SDHs account for the overwhelming majority (86 %) of those brain injury-related fatalities and are also implicated in the majority of boxing-related fatalities [66]. This association is not necessarily true for fatalities across all sports, however, and intracranial hemorrhage accounts for approximately half of amateur baseball-related fatalities and only a tiny portion of serious basketball-related injury [4, 24].
Biomechanics and Pathophysiology
Numerous studies have demonstrated the resilience of the human skull to a variety of biomechanical loads. Unembalmed cadaveric studies have demonstrated that the human skull can withstand blunt force loads of up to 14.1 kN (3170 lb, mean: 6.4 kN = 1439 lb) and energies of up to 68.5 J (50.5 foot-pounds, mean: 28.0 J = 20.7 foot-pounds) before fracture [103]. These values are roughly consistent across different cadaveric studies [25]. These measurements, however, are not necessarily representative of all anatomic regions of the human skull. The pterion and squamous temporal bone are known to be particularly weak, with lower peak forces needed to induce fracture [102]. Special consideration also needs to be given to the pediatric skull, which not only has thinner bone but also unfused cranial sutures in infants. In cadaveric studies of infants, falls from heights of 82 cm (~32 in.) have been shown to consistently produce skull fractures [98]. Skull thickness grows steadily in children, with 85–90 % of peak skull thickness present by the second decade of life and peak skull thickness typically achieved by the third decade of life [1, 34].
Of note, there exist differences in the generation of different types of skull fractures [64]. Linear fractures are typically the result of pure energy absorption by the skull after contact with a hard object, with little dispersion of the kinetic energy toward translation, rotation, or angulation. Depressed skull fractures often result from contact with smaller objects, which focus the energy to a smaller region of the skull. This focus can be significant enough to disrupt the bone underlying the site of impact in a circumferential manner, resulting in separation from the surrounding bone and depression of the fracture. Cases of basilar skull fracture can result from either transmission of mechanical forces from remote sites of impact, which can disrupt some of the relatively weaker structures of the skull base, or from direct pressure upon the bones of the face or skull base.
The pathophysiology of intracranial hemorrhage inevitably involves the disruption of the integrity of an intracranial vessel due to either direct laceration by a bone fragment or propagation of kinetic energy leading to shearing forces upon the vessel itself. The type and location of the vessel dictate the nature of subsequent hemorrhage.
In instances of EDH, the disrupted vessel lies between the skull and underlying dura and classically involves laceration of the middle meningeal artery due to an overlying skull fracture of the pterion (though venous bleeding from the middle meningeal vein, diploic veins, or venous sinuses have also been known to occur) [11, 68].
In cases of SDH, the disrupted vessels are located just beneath the dura but still on top of the arachnoid membrane. The classically involved vessels in cases of SDH are the bridging subdural veins and, in cases of young athletes without preexisting structural brain disease, are not easily damaged. Laceration of these vessels require either an overlying skull fracture or foreign object that has been propelled inward to lacerate the dura itself or a very high-energy impact such as from a high-speed motor vehicle collision. As such, acute traumatic SDH is associated with very significant injuries and prognosis is poor, with mortality estimates ranging from 40 to 60 % [12, 37, 55, 100]. In both SDH and EDH, the immediate concern is the risk of mass effect of the hematoma upon underlying brain tissue, which, if severe enough, will cause herniation and subsequent damage of critical neural structures.
A recent review attempted to set thresholds for acceleration values needed to produce SDH in football players. Reviewing cadaveric studies, animal studies, and finite-element simulations, a threshold of approximately 5000 rad/s [2] was set for rotational acceleration (RA) and 3000g for translational acceleration (TA) to cause venous rupture and subsequent SDH [28].
tSAH, on the other hand, is a much more common occurrence, but its precise etiology is unclear. In particular, though the natural history and etiology of aneurysmal and spontaneous SAH have been well documented, comparatively less has been written about the pathophysiology of SAH following trauma. It has been proposed that tSAH results from hemorrhage of pial vessels or possibly diffusion of blood from cerebral contusions into the subarachnoid space [91]. When present, the blood itself is rarely of sufficient quantity to cause significant mass effect on its own, but the chemical irritation of the blood is well known to produce significant headaches. Perhaps more significantly, there is a concern that tSAH predisposes the patient to delayed vasospasm and subsequent cerebral ischemia as is seen in cases of aneurysmal SAH [35, 89] (Fig. 7.2). These concerns are somewhat controversial, however, and many have proposed that tSAH is a process that is biologically distinct from aneurysmal SAH, with lower associated risks of vasospasm [31]. An additional controversy is whether tSAH predisposes patients to posttraumatic hydrocephalus, presumably through damage to cerebrospinal fluid (CSF) absorption pathways by hemorrhagic materials [90]. Other studies, however, have failed to find any correlation between the presence of tSAH and the development of posttraumatic hydrocephalus [74].
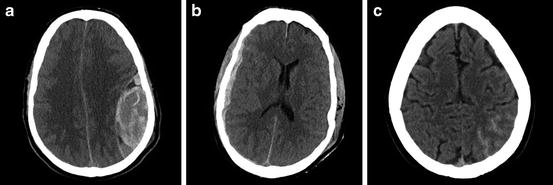
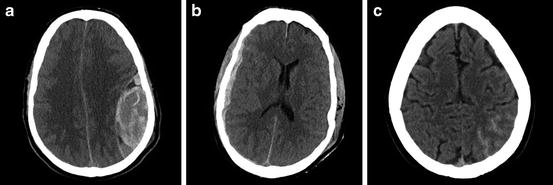
Fig. 7.2
(a, left) A large, left parietal epidural hematoma a 40-year-old male following falling down a flight of stairs. Note the characteristic ellipsoid appearance of the lesion and the associated midline shift. (b, middle) A right frontotemporal subdural hematoma in a 56-year-old male involved in a motorcycle accident. Note that the hematoma spreads across multiple suture lines. (c, right) Traumatic subarachnoid hemorrhage in the left occipital lobe in a 32-year-old male following a fall down a flight of stairs. Note the presence of hyper-attenuating substance tracking along the cortical sulci on the left compared to the open ones on the right
Initial Evaluation and Diagnosis
When assessing a patient with a suspected skull fracture or intracranial hematoma, there are several points that need to be considered in the initial assessment and workup. As with many forms of potentially serious trauma, timing to proper evaluation of the patient is key. As has been demonstrated in the literature on EDH in particular, the time between trauma and operation is consistently listed as one of the major predictors of outcome and mortality (while gender, age, mechanism of injury, EDH location, and shape are not) [7, 54, 78].
History taking should be thorough but focused and obtained from reliable witnesses if the patient is unable to accurately describe the incident themselves. Key factors to elucidate include:
Exact timing of the trauma (reports of general times of day, for instance, “earlier this evening,” can be misleading and are often insufficient)
Sequence of events surrounding the trauma, with consideration of the possibility that a change in mental status (from some unrelated pathology) leads to the trauma instead of the converse
Mechanism of injury (including good approximation of speeds and energies involved in the trauma)
Precise anatomic location of any cranial trauma (with special attention to possibility for the head to be launched from the primary impact and make a secondary impact)
Other bodily injuries, particularly significant ones that can “distract” the patient from relevant pain along the craniospinal axis, or other injuries that may impact the findings on neurologic examination
Post-trauma course, with special attention to mental status, amnesia, occurrence of any seizure or seizure-like episodes, and any potential therapies or workup that may already have been initiated
Focused review of systems, including nausea, vomiting, seizure, changes in mental status, any new-onset neurologic deficits, and reports of clear, salty fluid in the ears, nose, or throat which may be indicative of a CSF leak
Brief social history, with the understanding that the patient’s consumption of alcohol, tobacco, and illicit drugs can significantly impact their presentation and hospital course
Past medical history, including an assessment of baseline health and with particular focus on previous neurosurgical procedures or preexisting neurologic disease such as hydrocephalus, epilepsy, or recent history of head injury
In addition to the history, the physical examination can often provide key insights that may influence management. In addition to a proper trauma survey and neurologic examination (details of which are beyond the scope of this chapter), there are several important factors that are important not to miss as part of the initial examination. These include:
Vital sign abnormalities, in particular bradycardia, hypertension, and respiratory irregularities that may signal impending or ongoing increases in intracranial pressure.
Brief assessment of the patient’s mental status and level of consciousness. Determination of a patient’s Glasgow Coma Scale (GCS) score is important, and repeated studies have validated the utility of GCS in predicting outcomes and influencing management [11, 18, 38, 62].
Careful inspection of the entirety of the scalp, with clearing of any dirt or blood products if they obstruct proper inspection. Inspection of only the injury sites described in the patient history is often insufficient, and patient hair can very easily hide lacerations, hematomas, and bony defects unless explicitly examined.
Palpation for any cranial defects, step-offs, or inappropriate mobility. In certain cases of complex or depressed skull fracture, mobility of liberalized bone fragments can be easily appreciated.
Inspection for any signs of basilar skull fracture, including periorbital or postauricular ecchymosis or hematoma, and any new-onset deficits of any of the cranial nerves.
Examination of the tympanic membranes and nares for any signs of blood or CSF.
Neurologic examination should be quick but thorough, with focus given to detection of new-onset deficits. Any abnormalities must be interpreted in the context of the patient’s other injuries, current mental status, and baseline status of health.
In cases where structural brain or skull injury is suspected, computed tomography (CT) scan is the imaging modality of choice for further workup due to its speed and high sensitivity for both bony and hemorrhagic lesions. Plain skull radiograph is insufficient as a radiologic investigation of structural brain injury. Moreover, though some have proposed their utility as a screening tool for intracranial hemorrhage (based on the strong association between skull fractures and underlying structural imaging previously noted in this chapter), their usage in this manner remains controversial [17, 36, 42]. Magnetic resonance imaging (MRI), though possessing a superior ability to detect intracranial blood products, is typically too slow or unavailable for routine use in assessing head trauma.
In the setting of moderate to severe brain injury, focal neurologic deficit, or obvious skull fracture, prompt evaluation with a CT scan is necessary to assess for structural lesions. The decision to obtain imaging in cases of mild head injury, however, can be sometimes be more nuanced. Various guidelines exist for determining the need for CT scan in the setting of mild brain injury [39, 44, 72, 84, 86]. The Canadian CT Head Rule, one of the more widely applied schemas that has compared favorably against competing sets of guidelines, specifies that cases of mild head injury require a head CT only if they have [87]:
GCS score < 15 at 2 h following injury
Suspected open or depressed skull fracture
Any signs of basilar skull fracture
Two or more episodes of vomiting
Age greater than or equal to 65 years
Amnesia that covers greater than 30 min before the impact
Dangerous mechanism (pedestrian struck by motor vehicle, occupant ejected from a motor vehicle, fall from height greater than 3 ft or 5 stairs)
Though other guidelines differ in their specific cutoffs for imaging, all agree that radiologic evaluation should be conducted in a prompt fashion if there is any suspicion for underlying structural skull or brain injury [39, 44]. It is also important to keep in mind that neuroimaging is also necessary in head trauma patients if the patient’s mental status is impaired and a proper neurologic examination is unattainable (and thus a new neurologic deficit cannot be reliable excluded).
Based on findings of the CT scan, further imaging may be indicated. In particular, if CT scan demonstrates skull fracture extension over any of the dural venous sinuses or through vessel-containing foramina, CT angiography can be performed to assess for vessel integrity and patency [16, 105].
In addition to imaging, in cases of severe head injury, intracranial pressure monitoring may also be indicated. Continuous intracranial pressure monitoring, when combined with measurements of mean arterial pressure, can help assess cerebral perfusion pressure, an important, modifiable, predictor of outcomes in severe head injury [2, 19, 50]. Moreover, intracranial pressure monitoring can also be useful in the early detection of delayed-onset hemorrhage and expanding intracranial masses, which may require surgical intervention.
Intracranial pressure monitoring can be performed through installation of an invasive or noninvasive monitor by a trained neurosurgeon and is indicated in all salvageable head injury patients with GCS scores 3–8 (after resuscitation) and an abnormal CT scan [6]. Monitoring is also indicated in salvageable patients who meet two or more of the following criteria:
Age > 40 years
Unilateral or bilateral motor posturing (decerebrate or decorticate posturing)
Systolic blood pressures < 90 mmHg
In all instances of severe head trauma, prompt consultation with a neurotrauma specialist can help guide further possible workup and also facilitate transition of these patients from the emergent setting to a properly equipped and staffed intensive care unit.
Treatment
The treatment for skull fractures and associated structural brain injuries varies based on the severity of the pathology. When necessary, treatment for these lesions relies heavily on surgical intervention, as the pathology is anatomic in nature. There should be no hesitation to seek prompt neurosurgical consultation if the primary practitioner feels that operative intervention may be required or if they feel they require additional expertise or assistance.
Skull Fractures
Closed, nondepressed linear skull fractures without evidence of underlying structural parenchymal lesions do not require surgical intervention and can be managed expectantly (Table 7.1). Care should be geared toward reassurance and management of any potential coinciding nonstructural injuries.
Table 7.1
Overview of skull fractures
Nondepressed | Depressed | |
---|---|---|
Closed | Features: Can be linear or comminuted, can have extension into the facial bones or skull base | Features: Fracture fragments with inward displacement into the cranial vault, no evidence of communication with outside environment |
Mechanism: High-energy collision | Mechanism: Impact from a hard object with a smaller surface area and significant force | |
Management: Evaluate and rule out any associated underlying injures (cerebrospinal fluid leak, vascular disruption, intracranial hemorrhage, etc.). If no other injuries, expectant management | Management: Operative elevation and washout if fracture displaced greater than the distance of the adjoining skull. Follow for risk of infection and seizures | |
Open | Features: Can be linear or comminuted, must have communication with outside air through disruption of the skin and galea | Features: Fracture fragments with inward displacement, evidence of open communication with outside environment |
Mechanism: High-energy impact and associated deep skull laceration | Mechanism: Impact from a hard object with a smaller surface area and significant force and associated deep skull laceration | |
Management: Irrigation and closure | Management: If displacement greater than width of adjoining skull, operative elevation, debridement, and closure. Follow closely for risk of infection and seizures |
Open, nondepressed skull fractures without evidence of underlying hematomas can also typically be managed nonoperatively. The existence of an open communication to the outside world does raise the concern for increased infection risk, and in such cases thorough irrigation and approximation of the wound are prudent. Care should be taken, however, to ensure that there exists no disruption of the underlying dura and that the brain parenchyma has not been directly exposed to environmental pathogens.
Very little data exist on the management of simple (closed) depressed skull fractures due to their relative rarity compared to compound (open) depressed fractures [5, 17]. Current practice advocates surgical elevation if the degree of depression surpasses the full thickness of the surrounding skull. The thought is that significant displacement of the fracture will result in increased pressure and irritation of the underlying cortical surface (possibly raising in the incidence of posttraumatic epilepsy) and will also result in poor cosmetic appearance upon healing of the fracture [9]. However, as mentioned above, very little data exists to support this practice, and authors have proposed that surgery should be pursued only in cases of unacceptable cosmetic appearance or dural disruption [48, 85].
< div class='tao-gold-member'>
Only gold members can continue reading. Log In or Register a > to continue
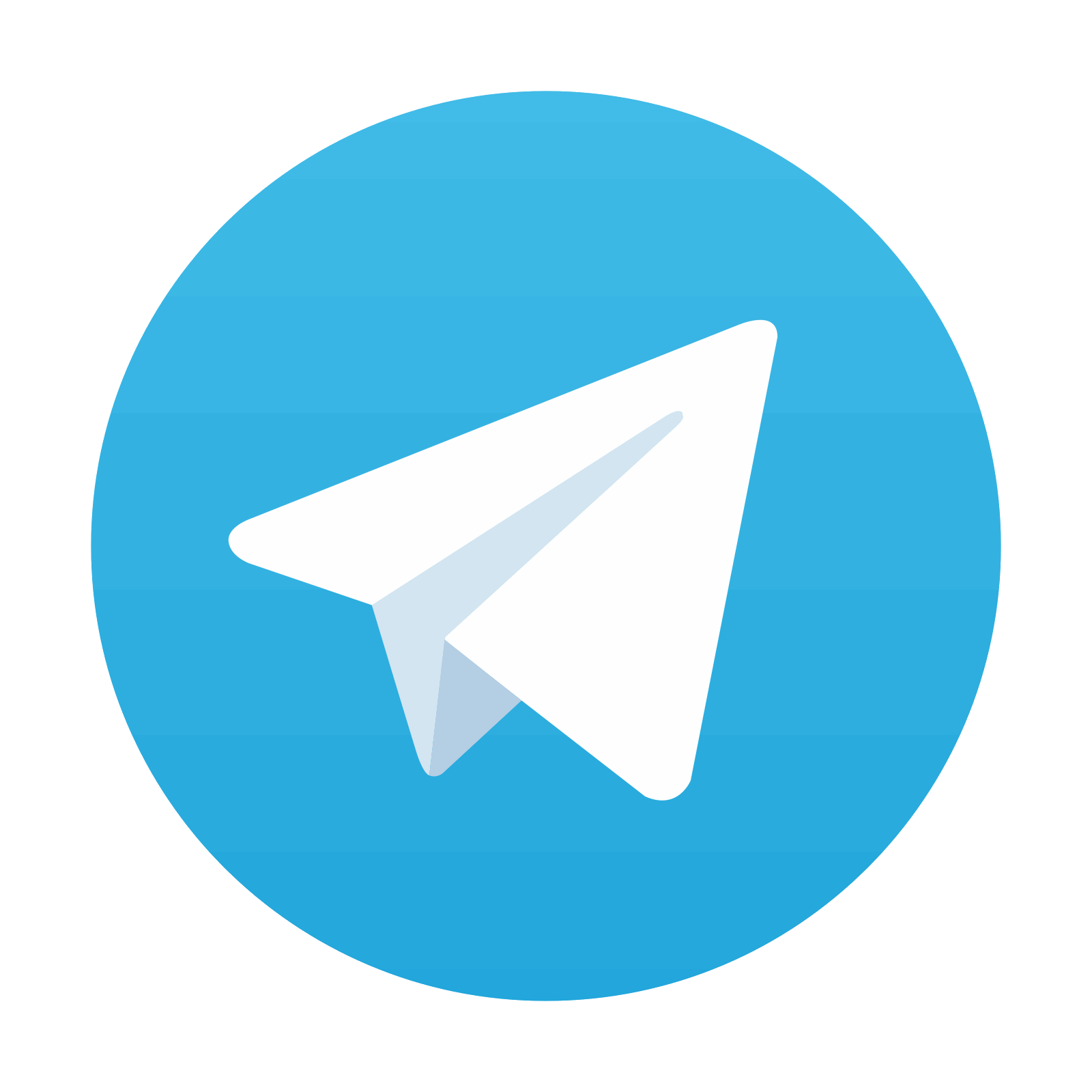
Stay updated, free articles. Join our Telegram channel

Full access? Get Clinical Tree
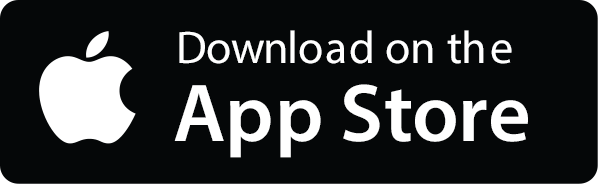
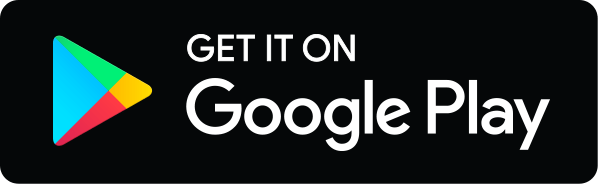