(1)
Department of Ophthalmology and Visual Sciences, University of Iowa Hospitals and Clinics, Iowa City, IA, USA
In 1855, Liebreich [1] first described the clinical picture of retinal vein occlusion and called it “retinal apoplexy.” Michel [2] established it as a clinical entity resulting from thrombosis in 1878. Duke-Elder and Dobree [3] accurately summarized the state of knowledge on retinal vein occlusion in 1967 when they stated: “Since that time (von Michel’s) the condition has been the subject of almost continuous research but nevertheless, even today, many points both in the aetiology and in the intimate mechanism of the obstruction are still unelucidated.” Later advances, however, have provided a good deal of new information on various aspects of central retinal vein occlusion (CRVO). The major problem in reviewing the information is that the majority of the reports in the literature have combined all types of retinal vein occlusions in one group, rather than describing information specifically on CRVO separately. I plan to discuss CRVO to provide the latest information on this common, important disease.
Classification of CRVO
My experimental study in 1964 in rhesus monkeys showed that CRVO is actually of the following two types [4, 5]. That finding has since been confirmed by a large number of clinical studies in patients (see below):
1.
Nonischemic CRVO
2.
Ischemic CRVO
Historically, this was an accidental discovery. In the early 1960s, I was experimentally investigating the pathogenesis of optic disc edema in raised intracranial pressure, which had been a controversial subject, the focus of numerous conflicting theories, since 1853. A popular theory at that time was that raised intracranial pressure increased the pressure in the optic nerve sheath and that pressed on the central retinal vein (Fig. 24.1) to produce optic disc edema. This was because in optic disc edema due to raised intracranial pressure, there is always engorgement of the retinal veins and optic disc and peripapillary hemorrhages. By inserting a balloon into the cranial cavity of rhesus monkeys and inflating it slowly, simulating a slowly growing intracranial tumor, I was able to produce optic disc edema with raised intracranial pressure experimentally for the first time [7, 8]. To find out whether the theory that raised intracranial pressure compresses the central retinal vein where it exits the optic nerve, I decided to close the central retinal vein at its exit from the optic nerve sheath (Fig. 24.2), by application of diathermy. In the first few experiments, it did not produce any appreciable optic disc edema similar to what I could produce with raised intracranial pressure, but it produced engorged retinal veins and a few punctate retinal hemorrhages. One day, while trying to apply diathermy to the central retinal vein, I accidentally touched the central retinal artery lying next to it with the diathermy probe (Fig. 24.2) and that closed both the vein and the artery. I felt the experiment was a failure, but the next day, the fundus showed what was described at that time as classical CRVO findings (Fig. 24.3). After that, I did the same occlusion intentionally and reproduced the same finding. That formed the basis of my discovery that CRVO was of two types. My subsequent studies showed that the pathogenesis of optic disc edema due to raised intracranial pressure resulted from a totally different mechanism [7].
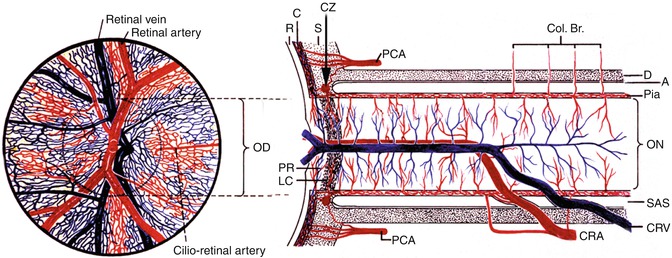
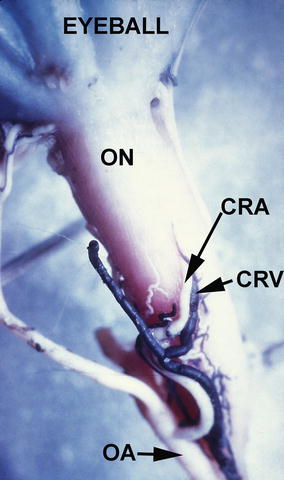
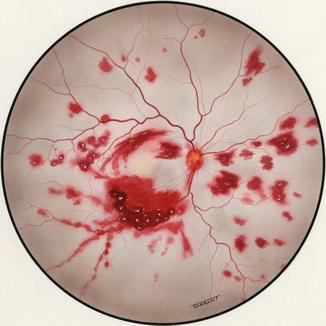
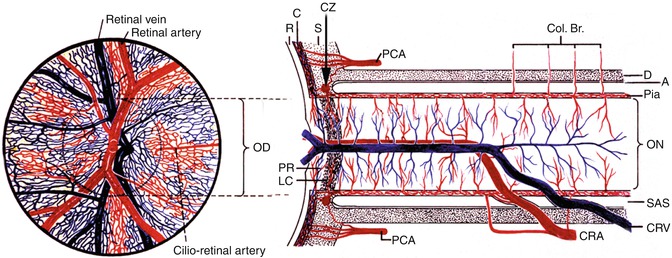
Fig. 24.1
Schematic representation of blood supply of the optic nerve (Modified from Hayreh [6]). A arachnoid, C choroid, CAR and CRA central retinal artery, Col. Br. collateral branches, CRV central retinal vein, CZ circle of Zinn and Haller, D dura, LC lamina cribrosa, OD optic disc, ON optic nerve, PCA posterior ciliary artery, PR prelaminar region, R retina, S sclera, SAS subarachnoid space
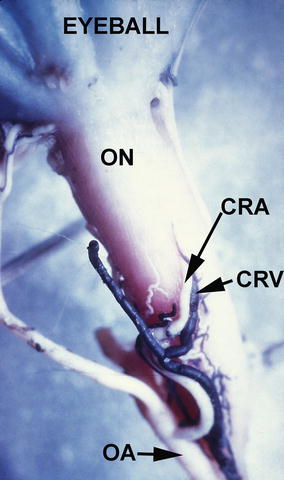
Fig. 24.2
Inferior surface of the intraorbital part of the optic nerve (ON), showing the central retinal artery (CRA) and vein (CRV) and their site of penetration into the sheath of the optic nerve in a rhesus monkey (Reproduced from Hayreh [5]). Abbreviations used: CRA central retinal artery, CRV central retinal vein, OA ophthalmic artery, ON optic nerve
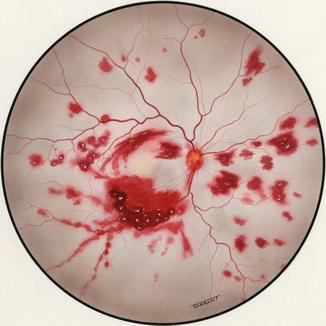
Fig. 24.3
Fundus drawing of the posterior pole of the fundus, on the third postoperative day after experimental occlusion of both the central retinal vein and artery in a rhesus monkey, shows hemorrhages below the macula, and small ones in relation to the small venules (Reproduced from Hayreh [5])
Conditions Associated with CRVO
There is a voluminous literature describing systemic conditions associated with CRAO. The presence of a particular associated systemic disease does not necessarily imply a cause-and-effect relationship with CRVO; that disease may or may not be one of the risk factors in a multifactorial scenario predisposing an eye to develop CRVO. Following is a summary of the conditions described in the literature. It is beyond the scope of this chapter to review and discuss the voluminous, often contradictory, literature on the subject of systemic diseases associated with CRVO.
Systemic Conditions
Kohner and Cappin [9] found a prevalence of arterial hypertension and diabetes mellitus in 116 patients with CRVO similar to that in the general population. Dodson and associates [10] found a significantly (p = 0.05) higher incidence of diabetes mellitus in CRVO (40 patients) compared with their control group (40 persons). Elman and associates [11], in a cross-sectional study of 197 patients with CRVO, found a significantly higher prevalence of arterial hypertension (p = 0.03) and diabetes mellitus (p = 0.005) when compared with that in the National Health Interview Survey, with no difference in prevalence of cerebrovascular or cardiovascular diseases. The largest series reported was that of the multicenter Eye Disease Case-Control Study Group [12], 258 patients – 84 with ischemic CRVO, 148 with nonischemic CRVO, and 26 not classified (but the criteria of differentiation between the two types were very different from those used by me [13]) – and 1,142 controls. In ischemic CRVO, they found a significant association with diabetes mellitus (p = 0.02), cardiovascular disease (p = 0.007), and arterial hypertension (p = 0.001); in nonischemic CRVO, the only significant associated condition was arterial hypertension (p = 0.002).
I investigated various systemic diseases and medications in 612 patients with CRVO. The findings are discussed in detail elsewhere [14], and following are the salient findings. There were 469 patients with nonischemic CRVO and 143 with ischemic CRVO. In the nonischemic group, the age range was 16–100 (median 62.7) years, 18 % young (<45 years), 38 % middle aged (45–64 years), and 44 % elderly (≥65 years); in the ischemic group, the age range was 31–99 (median 71.0) years, 8 % young, 27 % middle aged, and 65 % elderly. Table 24.1 gives detailed information about the various diseases seen in nonischemic and ischemic CRVO separately and collectively in young, middle-aged, and elderly persons. A comparison of findings in nonischemic and ischemic CRVO showed that there was a significantly greater prevalence of arterial hypertension (p = 0.025), diabetes mellitus (p = 0.011), and a tendency for smoking (p = 0.071) in the ischemic CRVO than in the nonischemic CRVO. There was no difference in ischemic heart disease, cerebrovascular disease, peripheral vascular disease, and venous diseases. Relative to the US white control population (my study had a mainly Caucasian population), the combined group of patients with CRVO and patients with hemi-CRVO (pathogenetically a variant of CRVO [15]) had a higher prevalence of arterial hypertension (p < 0.0001), peptic ulcer (p < 0.0001), diabetes mellitus (in ischemic type only, p < 0.0001), and thyroid disorder (p < 0.0001). But as mentioned above, the presence of a particular associated systemic disease does not necessarily imply a cause-and-effect relationship with that type of CRVO. Based on this study, I think that apart from a routine medical evaluation, an extensive and expensive work-up for systemic diseases is unwarranted in the vast majority of patients.
Table 24.1
Major systemic diseases (and some selected subgroups) present before or at the onset of CRVO
Number ( %) with condition present in each age group and CRVO type | ||||||||||||||||
---|---|---|---|---|---|---|---|---|---|---|---|---|---|---|---|---|
Age < 45 years | Age = 45–64 years | Age ≥ 65 years | All CRVO patients | |||||||||||||
Systemic condition | Nonischemic (n = 86) | Ischemic (n = 11) | Nonischemic (n = 178) | Ischemic (n = 39) | Nonischemic (n = 205) | Ischemic (n = 93) | Nonischemic (n = 469) | Ischemic (n = 143) | ||||||||
Cardiovascular diseases | ||||||||||||||||
Ischemic heart disease | 0 | 0 | 15 | (8.4) | 0 | 28 | (13.7) | 15 | (16.1) | 43 | (9.2) | 15 | (10.5) | |||
Valvular disease and atrial fibrillation | 2 | (2.3) | 0 | 10 | (5.6) | 2 | (5.1) | 19 | (9.3) | 10 | (10.8) | 31 | (6.6) | 12 | (8.4) | |
Peripheral vascular disease | 0 | 0 | 5 | (2.8) | 2 | (5.1) | 14 | (6.8) | 5 | (5.4) | 19 | (4.1) | 7 | (4.9) | ||
Congestive heart failure | 0 | 0 | 1 | (0.6) | 1 | (2.6) | 6 | (2.9) | 4 | (4.3) | 7 | (1.5) | 5 | (3.5) | ||
Hypertension | 5 | (5.8) | 6 | (54.5) | 66 | (37.1) | 20 | (51.3) | 100 | (48.8) | 47 | (50.5) | 171 | (36.5) | 73 | (51.0) |
Endocrine diseases | 2 | (2.3) | 1 | (9.1) | 23 | (12.9) | 10 | (25.6) | 34 | (16.6) | 20 | (21.5) | 59 | (12.6) | 31 | (21.7) |
Diabetes mellitus(DM) | 2 | (2.3) | 0 | 15 | (8.4) | 7 | (17.9) | 19 | (9.3) | 16 | (17.2) | 36 | (7.7) | 23 | (16.1) | |
Adult onset | 0 | 0 | 15 | (8.4) | 6 | (15.4) | 19 | (9.3) | 16 | (17.2) | 34 | (7.2) | 22 | (15.4) | ||
Juvenile onset | 2 | (2.3) | 0 | 0 | 1 | (2.6) | 0 | 0 | 2 | (0.4) | 1 | (0.7) | ||||
Thyroid disorders | 1 | (1.2) | 1 | (9.1) | 8 | (4.5) | 3 | (7.7) | 16 | (7.8) | 7 | (7.5) | 25 | (5.3) | 11 | (7.7) |
Hypertension + DM | 0 | 0 | 5 | (2.8) | 3 | (7.7) | 9 | (4.4) | 11 | (11.8) | 14 | (3.0) | 14 | (9.8) | ||
Cerebrovascular diseases | 1 | (1.2) | 0 | 6 | (3.4) | 1 | (2.6) | 9 | (4.4) | 4 | (4.3) | 16 | (3.4) | 5 | (3.5) | |
CVA | 0 | 0 | 3 | (1.7) | 0 | 6 | (2.9) | 3 | (3.2) | 9 | (1.9) | 3 | (2.1) | |||
TIA | 0 | 0 | 2 | (1.1) | 1 | (2.6) | 2 | (1.0) | 1 | (1.1) | 4 | (0.9) | 2 | (1.4) | ||
Amaurosis Fugax | 1 | (1.2) | 0 | 3 | (1.7) | 0 | 1 | (0.5) | 0 | 5 | (1.1) | 0 | ||||
Venous diseases | 1 | (1.2) | 0 | 1 | (0.6) | 0 | 2 | (1.0) | 2 | (2.2) | 4 | (0.9) | 2 | (1.4) | ||
Deep vein thrombosis | 1 | (1.2) | 0 | 0 | 0 | 0 | 0 | 1 | (0.2) | 0 | ||||||
Pulmonary embolism | 0 | 0 | 0 | 0 | 1 | (0.5) | 1 | (1.1) | 1 | (0.2) | 1 | (0.7) | ||||
Other venous occlusions | 0 | 0 | 1 | (0.6) | 0 | 1 | (0.5) | 1 | (1.1) | 2 | (0.4) | 1 | (0.7) | |||
Hypotensive episode | 0 | 0 | 1 | (0.6) | 0 | 2 | (1.0) | 1 | (1.1) | 3 | (0.6) | 1 | (0.7) | |||
Pulmonary diseases | 1 | (1.2) | 0 | 7 | (3.9) | 7 | (17.9) | 22 | (10.7) | 6 | (6.5) | 30 | (6.4) | 13 | (9.1) | |
COPDa | 0 | 0 | 3 | (1.7) | 4 | (10.3) | 12 | (5.9) | 4 | (4.3) | 15 | (3.2) | 8 | (5.6) | ||
Asthma | 1 | (1.2) | 0 | 3 | (1.7) | 2 | (5.1) | 5 | (2.4) | 2 | (2.2) | 9 | (1.9) | 4 | (2.8) | |
Bronchitis | 0 | 0 | 1 | (0.6) | 0 | 1 | (0.5) | 0 | 2 | (0.4) | 0 | |||||
Other pulmonary disease | 0 | 0 | 1 | (0.6) | 2 | (5.1) | 6 | (2.9) | 0 | 7 | (1.5) | 2 | (1.4) | |||
Hematologic diseases | ||||||||||||||||
Anemia | 2 | (2.3) | 1 | (9.1) | 1 | (0.6) | 4 | (10.3) | 8 | (3.9) | 7 | (7.5) | 11 | (2.3) | 12 | (8.4) |
Erythrocytosis | 0 | 1 | (9.1) | 2 | (1.1) | 0 | 1 | (0.5) | 0 | 3 | (0.6) | 1 | (0.7) | |||
Thrombocytosis | 0 | 0 | 2 | (1.1) | 0 | 1 | (0.5) | 3 | (3.2) | 3 | (0.6) | 3 | (2.1) | |||
Monoclonal gamm | 0 | 0 | 0 | 0 | 0 | 1 | (1.1) | 0 | 1 | (0.7) | ||||||
Polyclonal gamm | 0 | 0 | 0 | 0 | 1 | (0.5) | 0 | 1 | (0.2) | 0 | ||||||
Other hematologic conditions | 0 | 0 | 1 | (0.6) | 0 | 1 | (0.5) | 1 | (1.1) | 2 | (0.4) | 1 | (0.7) | |||
Gastrointestinal diseases | 4 | (4.7) | 0 | 20 | (11.2) | 3 | (7.7) | 29 | (14.1) | 14 | (15.1) | 53 | (11.3) | 17 | (11.9) | |
Peptic ulcer | 2 | (2.3) | 0 | 14 | (7.9) | 2 | (5.1) | 13 | (6.3) | 7 | (7.5) | 29 | (6.2) | 9 | (6.3) | |
Pancreatitis | 0 | 0 | 0 | 0 | 1 | (0.5) | 0 | 1 | (0.2) | 0 | ||||||
GI bleed | 0 | 0 | 1 | (0.6) | 1 | (2.6) | 1 | (0.5) | 1 | (1.1) | 2 | (0.4) | 2 | (1.4) | ||
Other GI diseases | 2 | (2.3) | 0 | 7 | (3.9) | 1 | (2.6) | 17 | (8.3) | 7 | (7.5) | 26 | (5.5) | 8 | (5.6) | |
Renal diseases | 1 | (1.2) | 1 | (9.1) | 6 | (3.4) | 3 | (7.7) | 10 | (4.9) | 7 | (7.5) | 17 | (3.6) | 11 | (7.7) |
Acute renal failure | 0 | 0 | 0 | 1 | (2.6) | 0 | 1 | (1.1) | 0 | 2 | (1.4) | |||||
Chronic renal failure | 0 | 1 | (9.1) | 1 | (0.6) | 3 | (7.7) | 6 | (2.9) | 3 | (3.2) | 7 | (1.5) | 7 | (4.9) | |
Dialysis | 0 | 1 | (9.1) | 0 | 2 | (5.1) | 1 | (0.5) | 0 | 1 | (0.2) | 3 | (2.1) | |||
Other renal diseases | 1 | (1.2) | 1 | (9.1) | 5 | (2.8) | 1 | (2.6) | 4 | (2.0) | 3 | (3.2) | 10 | (2.1) | 5 | (3.5) |
Hyperlipidemia | 1 | (1.2) | 1 | (9.1) | 17 | (9.6) | 6 | (15.4) | 17 | (8.3) | 5 | (5.4) | 35 | (7.5) | 12 | (8.4) |
Rheumatologic conditions | 1 | (1.2) | 3 | (27.3) | 13 | (7.3) | 3 | (7.7) | 25 | (12.2) | 12 | (12.9) | 39 | (8.3) | 18 | (12.6) |
Arthritis | ||||||||||||||||
Rheumatoid | 0 | 0 | 0 | 0 | 1 | (0.5) | 1 | (1.1) | 1 | (0.2) | 1 | (0.7) | ||||
Osteoarthritis | 0 | 0 | 3 | (1.7) | 0 | 4 | (2.0) | 1 | (1.1) | 7 | (1.5) | 1 | (0.7) | |||
Other inflammatory | 0 | 0 | 1 | (0.6) | 0 | 2 | (1.0) | 0 | 3 | (0.6) | 0 | (0.0) | ||||
Not specified | 0 | 1 | (9.1) | 3 | (1.7) | 1 | (2.6) | 8 | (3.9) | 7 | (7.5) | 11 | (2.3) | 9 | (6.3) | |
Others | 0 | 0 | 0 | 0 | 1 | (0.5) | 1 | (1.1) | 1 | (0.2) | 1 | (0.7) | ||||
Systemic lupus erythematosus | 0 | 1 | (9.1) | 0 | 0 | 0 | 0 | 0 | 1 | (0.7) | ||||||
Gout | 0 | 1 | (9.1) | 2 | (1.1) | 2 | (5.1) | 3 | (1.5) | 2 | (2.2) | 5 | (1.1) | 5 | (3.5) | |
Other rheumatologic conditions | 1 | (1.2) | 0 | 5 | (2.8) | 0 | 9 | (4.4) | 0 | 15 | (3.2) | 0 | ||||
Infections | ||||||||||||||||
Tuberculosis | 1 | (1.2) | 0 | 1 | (0.6) | 1 | (2.6) | 2 | (1.0) | 1 | (1.1) | 4 | (0.9) | 2 | (1.4) | |
Syphilis | 0 | 0 | 2 | (1.1) | 0 | 1 | (0.5) | 3 | (3.2) | 3 | (0.6) | 3 | (2.1) | |||
Herpes zoster | 0 | 0 | 1 | (0.6) | 0 | 0 | 2 | (2.2) | 1 | (0.2) | 2 | (1.4) | ||||
Urinary tract infection | 0 | 1 | (9.1) | 7 | (3.9) | 2 | (5.1) | 5 | (2.4) | 3 | (3.2) | 12 | (2.6) | 6 | (4.2) | |
URI/pneumonia | 2 | (2.3) | 0 | 1 | (0.6) | 0 | 2 | (1.0) | 1 | (1.1) | 5 | (1.1) | 1 | (0.7) | ||
Other infectious disease | 1 | (1.2) | 0 | 6 | (3.4) | 3 | (7.7) | 1 | (0.5) | 2 | (2.2) | 8 | (1.7) | 5 | (3.5) | |
Neurological diseases | 4 | (4.7) | 1 | (9.1) | 14 | (7.9) | 2 | (5.1) | 19 | (9.3) | 6 | (6.5) | 37 | (7.9) | 9 | (6.3) |
Seizures | 0 | 0 | 1 | (0.6) | 0 | 4 | (2.0) | 0 | 5 | (1.1) | 0 | |||||
Parkinson’s disease | 0 | 0 | 2 | (1.1) | 0 | 1 | (0.5) | 1 | (1.1) | 3 | (0.6) | 1 | (0.7) | |||
Peripheral neuropathy | 0 | 0 | 1 | (0.6) | 0 | 1 | (0.5) | 2 | (2.2) | 2 | (0.4) | 2 | (1.4) | |||
Psychiatric disorder | 3 | (3.5) | 0 | 3 | (1.7) | 1 | (2.6) | 3 | (1.5) | 1 | (1.1) | 9 | (1.9) | 2 | (1.4) | |
Other neurological disease | 2 | (2.3) | 1 | (9.1) | 7 | (3.9) | 1 | (2.6) | 12 | (5.9) | 2 | (2.2) | 21 | (4.5) | 4 | (2.8) |
Oncologic diseases/tumors | 1 | (1.2) | 1 | (9.1) | 8 | (4.5) | 0 | 27 | (13.2) | 15 | (16.1) | 36 | (7.7) | 16 | (11.2) | |
Intracranial | 0 | 0 | 1 | (0.6) | 0 | 0 | 0 | 1 | (0.2) | 0 | ||||||
Lung | 0 | 0 | 0 | 0 | 0 | 1 | (1.1) | 0 | 1 | (0.7) | ||||||
Gastrointestinal | ||||||||||||||||
Colon | 0 | 1 | (9.1) | 1 | (0.6) | 0 | 6 | (2.9) | 1 | (1.1) | 7 | (1.5) | 2 | (1.4) | ||
Stomach | 0 | 0 | 0 | 0 | 0 | 0 | 0 | 0 | ||||||||
Other GI | 0 | 0 | 0 | 0 | 0 | 0 | 0 | 0 | ||||||||
Genitourinary | 1 | (1.2) | 0 | 1 | (0.6) | 0 | 7 | (3.4) | 2 | (2.2) | 9 | (1.9) | 2 | (1.4) | ||
Prostate | 0 | 0 | 1 | (0.6) | 0 | 8 | (3.9) | 11 | (11.8) | 9 | (1.9) | 11 | (7.7) | |||
Skin | 0 | 0 | 1 | (0.6) | 0 | 1 | (0.5) | 0 | 2 | (0.4) | 0 | |||||
Breast | 0 | 0 | 2 | (1.1) | 0 | 3 | (1.5) | 2 | (2.2) | 5 | (1.1) | 2 | (1.4) | |||
Other neoplasms | 0 | 0 | 1 | (0.6) | 0 | 2 | (1.0) | 1 | (1.1) | 3 | (0.6) | 1 | (0.7) | |||
Surgery <6 months prior to CRVO | 2 | (2.3) | 2 | (18.2) | 4 | (2.2) | 0 | 13 | (6.3) | 3 | (3.2) | 19 | (4.1) | 5 | (3.5) | |
Head and neck | 0 | 0 | 0 | 0 | 3 | (1.5) | 0 | 3 | (0.6) | 0 | ||||||
Abdominal | 1 | (1.2) | 1 | (9.1) | 0 | 0 | 3 | (1.5) | 0 | 4 | (0.9) | 1 | (0.7) | |||
Genitourinary | 1 | (1.2) | 0 | 0 | 0 | 3 | (1.5) | 0 | 4 | (0.9) | 0 | |||||
Other surgery (not eye) | 0 | 1 | (9.1) | 4 | (2.2) | 0 | 4 | (2.0) | 3 | (3.2) | 8 | (1.7) | 4 | (2.8) | ||
Trauma | 1 | (1.2) | 0 | 5 | (2.8) | 0 | 3 | (1.5) | 1 | (1.1) | 9 | (1.9) | 1 | (0.7) | ||
Head injury | 0 | 0 | 2 | (1.1) | 0 | 2 | (1.0) | 0 | 4 | (0.9) | 0 | |||||
Periorbital injury | 0 | 0 | 1 | (0.6) | 0 | 0 | 0 | 1 | (0.2) | 0 | ||||||
Ocular injury | 1 | (1.2) | 0 | 2 | (1.1) | 0 | 1 | (0.5) | 0 | 4 | (0.9) | 0 | ||||
Other trauma | 0 | 0 | 0 | 0 | 0 | 1 | (1.1) | 0 | 1 | (0.7) | ||||||
Smoking | ||||||||||||||||
Smokers | (n = 82) | (n = 10) | (n = 163) | (n = 36) | (n = 183) | (n = 88) | (n = 428) | (n = 134) | ||||||||
Current | 11 | (13.4) | 4 | (40.0) | 55 | (33.7) | 6 | (16.7) | 30 | (16.4) | 10 | (11.4) | 96 | (22.4) | 20 | (14.9) |
Quit <5 years ago | 5 | (6.1) | 0 | 16 | (9.8) | 3 | (8.3) | 9 | (4.9) | 5 | (5.7) | 30 | (7.0) | 8 | (6.0) | |
Quit ≥5 years ago | 8 | (9.8) | 1 | (10.0) | 28 | (17.2) | 9 | (25.0) | 48 | (26.3) | 18 | (20.5) | 84 | (19.6) | 28 | (20.9) |
Pack-years (of smokers) | (n = 20) | (n = 4) | (n = 91) | (n = 18) | (n = 74) | (n = 29) | (n = 185) | (n = 51) | ||||||||
Median pack-years | 10.0 | 11.5 | 40.0 | 27.5 | 30.0 | 30.0 | 30.0 | 29.0 | ||||||||
Medications | ||||||||||||||||
Aspirin | 0 | 0 | 11 | (6.2) | 3 | (7.7) | 19 | (9.3) | 5 | (5.4) | 30 | (6.4) | 8 | (5.6) | ||
Persantine | 0 | 0 | 6 | (3.4) | 0 | 5 | (2.4) | 5 | (5.4) | 11 | (2.3) | 5 | (3.5) | |||
Other NSAIA | 0 | 0 | 10 | (5.6) | 2 | (5.1) | 17 | (8.3) | 5 | (5.4) | 27 | (5.8) | 7 | (4.9) | ||
Oral contraceptivesb | 8 | (21.6) | – | 0 | 0 | 0 | 0 | 8 | (3.8) | 0 | ||||||
Estrogen supplementsb | 2 | (5.4) | – | 6 | (8.7) | 0 | 2 | (2.0) | 3 | (5.9) | 10 | (4.8) | 3 | (4.8) | ||
Vasodilators | 1 | (1.2) | 1 | (9.1) | 8 | (4.5) | 5 | (12.8) | 19 | (9.3) | 10 | (10.8) | 28 | (6.0) | 16 | (11.2) |
Other antihypertensives | 0 | 1 | (9.1) | 12 | (6.7) | 4 | (10.3) | 25 | (12.2) | 12 | (12.9) | 37 | (7.9) | 17 | (11.9) | |
Beta-blockers | 1 | (1.2) | 3 | (27.3) | 13 | (7.3) | 3 | (7.7) | 23 | (11.2) | 11 | (11.8) | 37 | (7.9) | 17 | (11.9) |
Coumadin | 0 | 0 | 2 | (1.1) | 0 | (0.0) | 8 | (3.9) | 3 | (3.2) | 10 | (2.1) | 3 | (2.1) | ||
Digoxin | 0 | 0 | 6 | (3.4) | 5 | (12.8) | 18 | (8.8) | 8 | (8.6) | 24 | (5.1) | 13 | (9.1) | ||
Bronchodilators | 1 | (1.2) | 0 | 2 | (1.1) | 2 | (5.1) | 8 | (3.9) | 4 | (4.3) | 11 | (2.3) | 6 | (4.2) | |
Anti-lipid agents | 1 | (1.2) | 0 | 3 | (1.7) | 1 | (2.6) | 0 | 2 | (2.2) | 4 | (0.9) | 3 | (2.1) | ||
Oral hypoglycemics | 0 | 0 | 5 | (2.8) | 3 | (7.7) | 11 | (5.4) | 8 | (8.6) | 16 | (3.4) | 11 | (7.7) | ||
Insulin | 2 | (2.3) | 0 | 2 | (1.1) | 2 | (5.1) | 6 | (2.9) | 4 | (4.3) | 10 | (2.1) | 6 | (4.2) | |
Psychiatric | 1 | (1.2) | 1 | (9.1) | 11 | (6.2) | 5 | (12.8) | 9 | (4.4) | 0 | 21 | (4.5) | 6 | (4.2) | |
Diuretics | 1 | (1.2) | 3 | (27.3) | 32 | (18.0) | 10 | (25.6) | 40 | (19.5) | 30 | (32.3) | 73 | (15.6) | 43 | (30.1) |
Other medications | 13 | (15.1) | 4 | (36.4) | 29 | (16.3) | 8 | (20.5) | 59 | (28.8) | 41 | (44.1) | 101 | (21.5) | 53 | (37.1) |
While comparing the findings of my study with those of other studies on the subject, it is pertinent to point out that the design of my study differs markedly from all the others. The designs of the various other studies also differ from each other. Study design determines the outcome of a study, so it is hard to make any valid comparison between the various studies. Any comparison is bound to create unnecessary controversy and confusion. For example, the design of the studies by the multicenter Eye Disease Case-Control Study Group [12] differs markedly from my study in demographics, case selection criteria, diagnostic criteria, classification, and categorization used; mode of data collection; control groups used; and methods of assessment of systemic diseases. All these differences evidently make it impossible to compare the findings of my study directly with those of the Eye Disease Case-Control Study Group [12]. This is a good example of the fact that presumably similar studies on a topic are not necessarily parallel and their results are not comparable [16].
There are a number of anecdotal case reports of CRVO with many systemic diseases, including acquired immunodeficiency disease [17–28], arteriovenous fistula of the carotid artery to the cavernous sinus [29–34], acute myeloid leukemia [35], acute retinal necrosis [36], angle-closure glaucoma, Behçet’s disease [37, 38], bone marrow transplantation [39], cannabis smoking [40], cavernous sinus thrombosis [41, 42], Churg-Strauss syndrome [43], contraceptive pills [44–46], cosmetic iris implants [47], Crohn’s disease [48–51], cyanotic heart disease [52], dehydration [53], Eisenmenger syndrome [54], essential thrombocythemia [55, 56], exercise induced [57], following cardiac surgery [58], following pars plana vitrectomy [59], pseudoexfoliation of the lens capsule [60–63], laser in situ keratomileusis [64], glucose-6-phosphate dehydrogenase deficiency [65], headstand posture in Yoga (Sirsasana) [66], hemodialysis [67], human herpes virus [68], high altitude [69, 70], hormone replacement therapy [71], interferon therapy [72–76], intraocular nematode [77], isotretinoin use for acne [78], Leber’s hereditary optic neuropathy [79], licorice ingestion [80], malignant arterial hypertension [81], melanocytoma of the optic disc [82, 83], metastatic adenocarcinoma in the optic nerve sheath [84], migraine [85], multiple myeloma [86, 87], non-Hodgkin’s lymphoma [88–90], occult colon cancer [91], optic disc drusen [92], orbital inflammatory disease [93–96], orbital rhabdomyosarcoma [97], phosphodiesterase type 5 inhibitors [98, 99], playing a wind instrument [100], posterior multifocal placoid pigment epitheliopathy [101–103], posterior scleritis [104–106], preeclampsia [107–109], pregnancy [110], primary pulmonary hypertension [54, 111], pulmonary tuberculosis [112], renal cell carcinoma [113], retinal arteriovenous malformation [114], retrobulbar anesthesia [115–117], sarcoidosis [118], sleep apnea syndrome [119, 120], sickle cell disease [121, 122], Sneddon syndrome [123], Sturge-Weber syndrome [124], superior ophthalmic vein thrombosis [125], systemic lupus erythematosus [126–129], syphilis [130], thrombocytopenic purpura [131, 132], trauma [133, 134], ulcerative colitis [135–138], vaccination [139–141], Wegener’s granulomatosis [142], and Waldenstrom’s disease [143–147]. As stressed above, the presence of a particular associated systemic disease does not necessarily imply a cause-and-effect relationship with CRVO.
Hematologic Abnormalities
A tremendous amount of literature has accumulated over the years on hematologic abnormalities associated with CRVO, but most reports are based either on anecdotal cases or on retrospective retrieval of information from case records of variable numbers of patients seen in routine clinical practice, with only a few planned studies. In spite of that, the role played by various hematologic abnormalities in the etiology and pathogenesis of CRVO still remains unclear and controversial.
I investigated hematologic abnormalities in 341 (nonischemic 279 and ischemic 62) CRVO patients [148]. Virtually all patients in this study were Caucasian, which is consistent with the racial pattern in Iowa. My study dealt with complete blood count, differential white cell count, lipids, blood urea nitrogen, creatinine, uric acid, calcium, phosphorus, glucose, albumin, antinuclear antibody, and special studies on platelet aggregation, antithrombin III, and α2 globulin. Ischemic CRVO showed a significantly higher prevalence of abnormal hematocrit (p = 0.044), hemoglobin (p = 0.018), and blood urea nitrogen (p = 0.025) than nonischemic CRVO, while a significantly higher prevalence of abnormal antinuclear antibody (p = 0.049) was seen in nonischemic CRVO than in ischemic CRVO. There was no significant prevalence of abnormal circulating platelet aggregation, antithrombin III, or α2 globulin.
I also conducted a small pilot study to investigate activated protein C resistance and heterozygosity for the factor V Leiden allele in ten patients with CRVO (seven nonischemic and three ischemic CRVO), aged 20–50 years. Only one of them had a positive result and that was a young woman with a history of miscarriages.
I critically reviewed the literature dealing with thrombophilia in CRVO [148]. In most of the studies, all types of retinal vein occlusion are combined into one group. There are positive and negative studies dealing with anticoagulant proteins, activated protein C resistance and factor V Leiden mutation, antiphospholipid antibodies, methylenetetrahydrofolate reductase mutation and anticardiolipin antibodies, platelet aggregation disorders, other clotting abnormalities, hyperhomocysteinemia, plasma viscosity, and hyperlipidemia. From this review of this contradictory literature, it is reasonable to conclude that, contrary to many claims, we have no definite evidence of a cause-and-effect relationship between different hematologic abnormalities and development of various types of retinal vein occlusion in the vast majority of retinal vein occlusion patients. Moreover, for many of these hematologic disorders, the required treatment is anticoagulants or agents which reduce platelet aggregation. I have many patients whose CRVO developed while they were taking anticoagulants for other cardiovascular disorders [149], which clearly shows that the hematologic disorder(s) which are blamed for CRVO could not have been responsible. Di Capua et al. [150] in a recent large case-control study did not find any association between CRVO and thrombophilic risk factors, including homocysteine levels and anticardiolipin antibodies. Dong et al. [151] reported that their data do not support the hypothesis that raised total homocysteine concentrations are an independent risk factor for CRVO. A chance occurrence of some of these hematologic abnormalities in the retinal vein occlusion cases or the possibility of the findings being due to unrelated concomitant systemic disease cannot be ruled out.
In the literature, it has often been claimed that retinal venous thrombosis is a manifestation of a hypercoagulable state in the patient. Primary hypercoagulable states are attributed to defects in the normal anticoagulant mechanisms. One finds a number of unsupported assumptions, which have caused considerable confusion. For example, it has often been assumed that the presence of an associated hematologic abnormality in patients with CRVO represents a cause-and-effect relationship. Moreover, in most of the previous literature, it was assumed that retinal vein occlusion is a single disease, and the various types of retinal vein occlusion were all grouped together.
Case Report
The following case is instructive and illustrates my usual experience with hematologic evaluation in CRVO. A 50-year-old, perfectly healthy man developed nonischemic CRVO in his right eye which resolved completely in about 18 months. A recurrence of nonischemic CRVO developed in the right eye 21 months later. Nine months after that, nonischemic CRVO developed in his left eye, which converted within 3 months to ischemic CRVO. When he had bilateral CRVO, an extensive hematologic evaluation was performed – including complete blood count; differential white cell count; hemoglobin, hematocrit, and platelet count; erythrocyte sedimentation rate; prothrombin time; partial thromboplastin time; antithrombin III; serum immunoelectrophoresis; serum cryoglobulins; monoclonal protein; serum viscosity; antinuclear antibody; cardiolipin antibody (IGG, IGM) screen; proteins C and S; factor V Leiden; homocysteine level; blood chemistries; fasting glucose; and lipid profile – which revealed no abnormality at all. His systemic evaluation also revealed absolutely no abnormality. If one expects a hematologic abnormality in CRVO, this is the case where one should find it. The lack of abnormal results from special hematologic studies and the hypercoagulability profile seen in this patient are not an exception but are usually the norm in the large cohort of CRVO patients I have seen during the past half century. One or more of these special hematologic and hypercoagulable abnormalities may occasionally be seen in the CRVO cases, which may or may not have any relevance to the development of CRVO, because such abnormalities do occur in a certain proportion of the general population without CRVO, as shown by studies in the literature. On a cost-benefit ratio, my study reveals that it is not worth investigating every patient by performing extensive and expensive special hematologic and hypercoagulability investigations. The routine, inexpensive hematologic evaluation is usually sufficient for CRVO patients.
So from the clinical point of view, what is the practical implication of all this? Ingerslev [152] very well summarized the state of our knowledge on the subject when he stated “Most well characterised (hematologic) risk factors for general venous thrombosis occur sporadically only in RVO (retinal vein occlusion), and it seems these have no major importance in the pathophysiology of RVO.” In view of this, he rightly recommended that “there seems to be no particular reason that this should include a complete haemostasiological investigation like that offered to patients with spontaneous major venous thromboembolism.” Faude et al. [153] in a study of 107 patients with CRVO concluded that activated protein C resistance has no major role in the pathogenesis of CRVO. Routine testing for the presence of factor V Leiden mutant in CRVO is not necessary. Rehak and Wiedemann [154] also reported that there is no evidence to suggest routine testing for heritable thrombophilias in patients with RVO. My study and experience supports this view. This explains why patients rarely develop bilateral CRVO, and even in the same eye, it is rare to have more than one type of retinal vein occlusion. The retinal venous occlusive process would not be so very localized and individualized if, as is often claimed, just one or two particular hematologic or systemic factors were causative of venous thrombosis; in that case, surely the involvement of the retinal veins of both eyes and other veins in the body would be widespread in such an individual. Moreover, development of CRVO due to thrombophilia is an oversimplification and generalization of a complex, multifactorial phenomenon.
Association of Ocular Hypertension and Glaucoma with CRVO and Hemi-CRVO (HCRVO)
The first person to note the role of glaucoma in the development of CRVO seems to have been Verhoeff [155] in 1913, based on his histopathological studies. He postulated that the most important factor is probably the increased intraocular pressure (IOP) compressing and collapsing the wall of the retinal vein, leading to intimal proliferation in the vein. Since HCRVO is a variant of CRVO (see Chap. 25), I investigated the association of ocular hypertension and glaucoma in both at their onset – CRVO in 548 (440 nonischemic and 108 ischemic) patients and HCRVO in 126 (99 nonischemic and 27 ischemic) patients, with no CRVO/HCRVO in the other eye. The findings are discussed at length elsewhere [156].
Table 24.2 gives the prevalence of various types of glaucoma and ocular hypertension in nonischemic and ischemic CRVO and HCRVO. There was no significant difference in the proportion of patients with elevated IOP among the four types of retinal vein occlusion (p = 0.159 – Table 24.2). This provides additional evidence that HCRVO is a variant of CRVO.
Table 24.2
Prevalence of various types of glaucoma and ocular hypertension
Glaucoma type | Total (n = 674) | Nonischemic CRVO (n = 440) | Ischemic CRVO (n = 108) | Nonischemic hemi-CRVO (n = 99) | Ischemic hemi-CRVO (n = 27) |
---|---|---|---|---|---|
Various types of glaucoma | 67 (9.9 %) | 35 (8.0 %) | 13 (12.0 %) | 14 (14.1 %) | 5 (18.5 %) |
Primary open-angle glaucoma | 41 (6.1 %) | 23 (5.2 %) | 7 (6.5 %) | 7 (7.1 %) | 4 (14.8 %) |
Normal-tension glaucoma | 8 (1.2 %) | 1 (0.2 %) | 3 (2.8 %) | 3 (3.0 %) | 1 (3.7 %) |
Pigmentary glaucoma | 3 (0.5 %) | 3 (0.7 %) | 0 (0.0 %) | 0 (0.0 %) | 0 (0.0 %) |
Pseudoexfoliation glaucoma | 9 (1.3 %) | 6 (1.4 %) | 2 (1.9 %) | 1 (1.0 %) | 0 (0.0 %) |
Other types of glaucoma | 6 (0.9 %) | 2 (0.5 %) | 1 (0.9 %) | 3 (3.0 %) | 0 (0.0 %) |
Ocular hypertension | 109 (16.2 %) | 72 (16.4 %) | 14 (13.0 %) | 21 (21.2 %) | 2 (7.4 %) |
Glaucoma + ocular hypertension | 176 (26.1 %) | 107 (24.3 %) | 27 (25.0 %) | 35 (35.4 %) | 7 (25.9 %) |
Comparing the prevalence of primary open-angle glaucoma in CRVO and HCRVO with that reported by Tielsch et al. [157] in the United States’ Caucasian general population and also with that reported in the general population in the Framingham study [158] for persons who were 50 years or older, a significantly higher prevalence of primary open-angle glaucoma was found in CRVO and HCRVO patients aged 50 or older. There was no significant difference in the proportion of patients with glaucoma/ocular hypertension among the four types of retinal vein occlusion (p = 0.159 – Table 24.2). Of the total CRVO and HCRVO patients, 77 (11.4 %) were already known to have elevated IOP prior to developing the vein occlusion. Fifty-three (68.8 %) of these 77 patients were being treated by ocular hypotensive therapy at the time of onset of retinal vein occlusion.
Time to resolution of retinopathy in CRVO among young, middle aged, and elderly with glaucoma/ocular hypertension and without glaucoma/ocular hypertension showed no significant difference.
The IOP of the eye with CRVO/HCRVO at the first visit was compared to that of the fellow normal eye, in the following three groups of patients:
1.
In patients that had normal IOP, there was a significantly lower IOP in the eye with CRVO than in the fellow normal eye (p < 0.0001).
2.
In patients that had elevated IOP at the time of diagnosis of CRVO/HCRVO and were not on ocular hypotensive therapy, the eye with CRVO/HCRVO had a significantly lower IOP than the uninvolved eye (p < 0.0001).
3.
In patients that had had elevated IOP prior to CRVO/HCRVO and were on ocular hypotensive therapy in both eyes, there was no significant difference in IOP between the eye with CRVO/HCRVO and the fellow normal eye (p = 0.145).
The Association of Ocular Hypotension with CRVO
I have found that ophthalmologists generally are not aware of this phenomenon. In my study [156], the overall prevalence of ocular hypotension at the initial visit was significantly (p < 0.0001) higher in glaucoma/ocular hypertension patients not on ocular hypotensive therapy than in non-glaucoma patients where the intraocular pressure in the CRVO/HCRVO eye was lower by 2 mmHg or more compared to the IOP in the fellow normal eye (Fig. 24.4). The magnitude of the fall of IOP also differed significantly between these two groups of patients (p < 0.0001). The eye with CRVO/HCRVO had an IOP that was lower than the IOP of the uninvolved eye by 5–9 mmHg in 25 % and 10 mmHg or more in 11 % of patients with glaucoma/ocular hypertension. In contrast, in patients without glaucoma/ocular hypertension, these were 5 and 0.4 %, respectively. Among the patients without glaucoma, the prevalence of ocular hypotension differed significantly among the four types of retinal vein occlusion (p = 0.007), with the nonischemic HCRVO patients having a lower prevalence of ocular hypotension than the other retinal vein occlusion types. The same was observed among the glaucoma/ocular hypertension patients that were on ocular hypotensive therapy (p = 0.015). Among the glaucoma/ocular hypertension patients not on ocular hypotensive therapy, no significant difference in the prevalence of ocular hypotension was seen among the four types of retinal vein occlusion (p = 0.374). Of those patients that had ocular hypotension at the initial visit, IOP equalized to within 1 mmHg between the two eyes in 72 % during the follow-up period. There was a significant (p < 0.0001) reduction in the prevalence of ocular hypotension from the initial visit to the time when retinopathy had resolved. Cupping of the optic disc at the initial visit was seen in 61 % of nonischemic CRVO, 54 % of ischemic CRVO, 75.0 % of nonischemic HCRVO patients, and 100 % of ischemic HCRVO. I found not uncommonly, in eyes with raised intraocular pressure, that when CRVO developed, intraocular pressure usually dropped in the involved eye to a normal level (mostly about 16 mmHg), while in the fellow uninvolved eye, it was still elevated. I have jokingly told my residents and fellows that the most effective treatment to lower intraocular pressure in ocular hypertension and glaucoma is to produce CRVO!
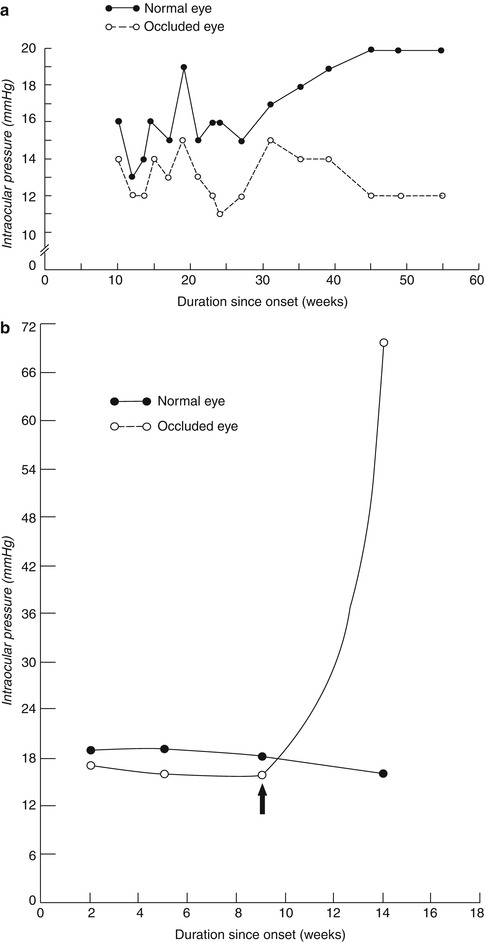
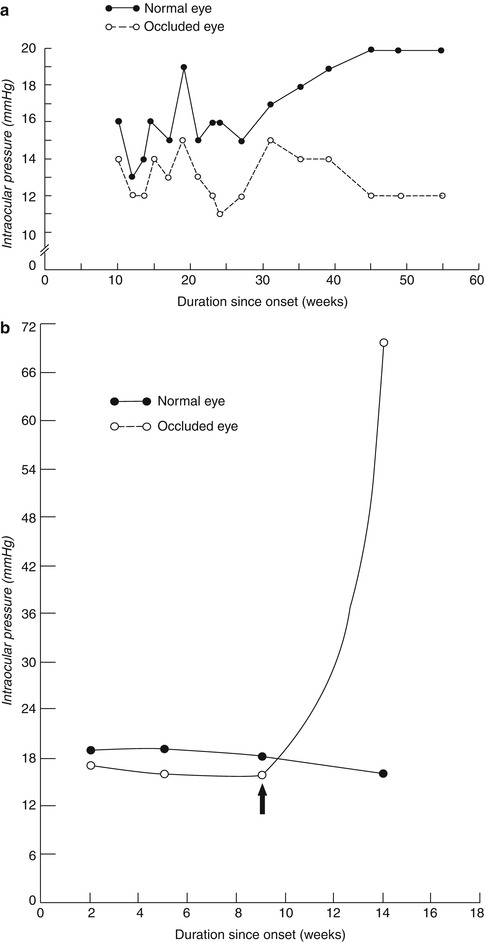
Fig. 24.4
Intraocular pressures in the involved eye and the fellow normal eye (a) in nonischemic CRVO and (b) in ischemic CRVO. In (b), arrow indicates the start of development of neovascular glaucoma
My findings confirmed previous reports of an increased prevalence of glaucoma in CRVO patients. I tried to eliminate the various confounding factors common in previous studies, to find a more realistic prevalence rate by including only patients seen consecutively, as a part of a planned study on CRVO. I excluded neovascular glaucoma secondary to ischemic CRVO. In addition, I also divided CRVO into ischemic and nonischemic, using strict criteria discussed below. The role of glaucoma/ocular hypertension in the pathogenesis of CRVO/HCRVO is discussed in the pathogenesis of CRVO below.
Moore [159] first reported in 1922 that when CRVO develops in an eye, the intraocular pressure in that eye is usually lower than that in the fellow normal eye; since then, several reports have confirmed that association. However, the reported prevalence (9–83 %) of ocular hypotension in CRVO eyes and the extent of it have varied widely in the literature. This may be due to the number of patients examined as well as to other variables among different studies, as suggested by my study.
Several studies have reported that after a variable length of time, the IOP in the two eyes usually becomes equal again [159–162]. In my study, the IOP equalized in 72 % of the CRVO patients that had hypotony at the initial visit. This corresponded to the decrease in the prevalence of ocular hypotension, from initial visit to resolution of retinal vein occlusion, in patients without glaucoma/ocular hypertension. In ischemic CRVO, if the eye starts to develop neovascular glaucoma, the IOP in the involved eye starts to go up, and ultimately it goes much higher than that in the fellow normal eye (Fig. 24.4b).
Pathogenesis of the Development of Ocular Hypotension in CRVO
So while the association of active retinopathy with ocular hypotension in CRVO is well established in patients, and in experimentally produced retinal vein occlusion [10], its exact pathogenesis still remains an enigma. Several theories have been postulated to explain this phenomenon [162–166]. The explanation that I favor is: the development of CRVO → retinal hypoxia → liberation of a “hypotensive factor” by the hypoxic retina → fall of IOP. Interestingly, in diabetic patients, IOP is also usually lower in an eye with proliferative retinopathy than in the fellow eye without retinopathy [167–170]. The nature of the “hypotensive factor” is still not clearly understood. In 1982 [163], I stated that the evidence indicates that the “hypotensive factor” most probably is prostaglandins; other studies later showed that prostaglandins do lower IOP, and that is now a well-established mode of treatment for ocular hypertension.
Clinical Importance of the Findings of My Study
1.
Interestingly, very few of my patients with CRVO had a high IOP in the involved eye in spite of its presence in the fellow uninvolved eye. Thus, for many patients, CRVO seems to lower IOP from a high to a normal level.
2.
Ophthalmologists should be aware that patients with CRVO have a higher than normal prevalence of glaucoma/ocular hypertension and that the presence of ocular hypotension in the involved eye may mislead them. It is a good practice to suspect that all patients with CRVO have glaucoma unless proven otherwise, as other authors [160, 164] have also stressed.
3.
I have found that most ophthalmologists advise CRVO patients to use IOP-lowering eye drops in the involved eye, under the prevalent impression that lowering the IOP helps to improve the retinal blood flow in that eye. There is no scientific evidence to support that impression. First, in my studies of more than 700 patients with CRVO during the past 40 years, I have found that IOP is usually already as low as it will go in most of those eyes, so that the addition of IOP-lowering drops usually has no significant effect. Second and most importantly, in an eye with CRVO and normal IOP, unlike in normal eyes, lowering the IOP cannot improve retinal blood flow if one takes into consideration the various factors influencing the ocular blood flow [171].
4.
Most importantly, if the fellow eye has ocular hypertension, that eye needs ocular hypotensive therapy for two reasons: (a) to prevent the development of glaucomatous changes [172], and (b) my study [156] and others [155] have shown a significant association between CRVO and ocular hypertension/glaucoma. Thus, ocular hypertension is a risk factor for the development of CRVO. In a multifactorial disease, reducing as many risk factors as possible helps to reduce the chances of developing the disease. Therefore, it is desirable to lower IOP in eyes with ocular hypertension to reduce that risk. Unfortunately, ophthalmologists tend to put IOP-lowering drops in the wrong eye!
Pathogenesis of CRVO
A good understanding of the pathogenesis of a disease is absolutely fundamental to fully grasp its clinical features and its logical management. I have discussed the pathogenesis of CRVO elsewhere [173–177].
Experimental Studies
To explore the pathogenesis of CRVO, I conducted an experimental study in rhesus monkeys. My findings are discussed in detail elsewhere [174]; following is a brief account. I occluded the central retinal vein at its exit from the optic nerve sheath (Fig. 24.2) in the following five groups: group 1, CRVO alone; group 2, CRVO with simultaneous central retinal artery occlusion; group 3, CRVO with transient clamping of the central retinal artery for either 2–2½ h (group 3A) or 6–7½ h (group 3B); and group 4, CRVO with segmental retinal ischemia (by branch retinal artery occlusion in one sector only).
The various types of retinal vascular occlusion revealed the following:
Group 1: Central retinal vein occlusion alone produced only a mild venous stasis retinopathy (i.e., nonischemic CRVO).
Group 2: Simultaneous CRVO and central retinal artery occlusion did not produce ischemic CRVO retinopathy but produced a picture of central retinal artery occlusion.
Group 3A: CRVO with 2–2½-h retinal ischemia produced nonischemic CRVO and not ischemic CRVO retinopathy.
Group 3B: CRVO with 6–7½-h retinal ischemia produced a picture greatly resembling that of ischemic CRVO.
Group 4: In sectoral retinal ischemia combined with CRVO, the ischemic part developed changes resembling ischemic CRVO, but the nonischemic part showed a mild form of nonischemic CRVO.
Retinal capillary obliteration on fluorescein angiography was seen in eyes of groups 2, 3B, and 4 (in group 4, only in the ischemic part of the retina), starting 1–3 weeks after the occlusion, patchy at first and extensive after about 3 months.
There was no associated retinal or optic disc neovascularization in these eyes, even on follow-up of up to 9 months.
Clinicopathological [178] and ultrastructural [179] studies of simultaneous occlusion of the central retinal vein and artery in this study are described elsewhere.
These results show that fundus changes greatly resembling ischemic CRVO were produced only when the CRVO was combined with either prolonged retinal ischemia (of 6–7½-h duration – group 3B) or segmental retinal ischemia (group 4) with ischemic CRVO developing in the ischemic sector only and rarely in group 3A.
Compared to the clinical situation, there is a limitation in this experimental study. In the clinically seen CRVO, the site of occlusion in the central retinal vein is in the optic nerve, but in this experimental model, it was where the central retinal vein exits the optic nerve sheath (Fig. 24.2); however, it is not possible to produce occlusion in the optic nerve experimentally. The site of occlusion in the central retinal vein is an important factor in determining the available collateral channels that can drain the retinal blood in the event of occlusion, and that in turn determines whether ischemic or nonischemic CRVO develops. The central retinal vein has multiple prominent tributaries throughout its course within the optic nerve and at its point of exit from the nerve (Figs. 24.1 and 24.5). The branches in the prelaminar region communicate with the peripapillary choroidal veins and those within the optic nerve with the pial veins. In the event of occlusion, the branches anterior to the site of occlusion show compensatory enlargement and develop into collaterals. When the occlusion is in the lamina cribrosa or close to it, the only available communications are in the prelaminar region and are insufficient to cope with the entire volume of retinal blood flow, producing embarrassment in the arterial circulation. This predisposes the eyes of susceptible individuals to develop ischemic CRVO. In contrast to this, when the site of occlusion is farther back in the optic nerve, more collaterals are available, and minimal or no secondary arterial embarrassment is produced; that results in the development of nonischemic CRVO. Thus, the severity of the clinical findings depends partly on the site of venous occlusion – the farther back the occlusion, the greater the number of collaterals available and the less severe the ischemia. In addition, a young healthy vascular bed has a tremendous potential for developing collaterals quickly, compared to the old and arteriosclerotic vascular bed. In the animal model, also, the vascular occlusion was of sudden onset and nonprogressive, while in humans, it is a gradually progressive process as the thrombus in the central retinal vein slowly increases in size. These factors might have influenced the difference in the development of ischemic CRVO in my experimental model and required prolonged ischemia.
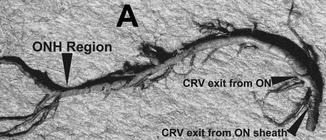
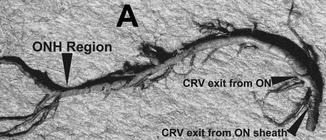
Fig. 24.5
Neoprene cast of the central retinal vein in my study shows its entire course from the optic nerve head to its exit from the optic nerve sheath. Note the presence of a large number of prominent venous tributaries within the optic nerve and none in the optic nerve head region in this specimen. At the left end are its retinal branches (Reproduced from Hayreh et al. [180]). Abbreviations used: CRV central retinal vein, ON optic nerve, ONH optic nerve head
My study and all the available information strongly support the important role of ischemia in the production of ischemic CRVO. In the light of this study [174], in my paper, I discussed in detail the pathogeneses of nonischemic and ischemic CRVO.
Clinical Studies
Except for a rare case where the disease that resulted in the development of CRVO is known, its pathogenesis is generally ill understood and controversial. Many theories have been postulated to explain it, but none has stood the test of time. There is almost a universal tendency to blame one or more factors as the cause/causes of CRVO, and generalizations are often made from an anecdotal association, but associations do not necessarily have a cause-and-effect relationship. Available evidence strongly suggests that the pathogenesis of CRVO, like that of many other ocular vascular occlusive disorders [181], is a multifactorial process. It seems that some risk factors predispose an individual or an eye to CRVO (predisposing risk factors), while others act as the final insult to produce clinically evident disease (precipitating risk factor(s)). The various risk factors for CRVO may be local (in the eye or the central retinal vein), systemic, or hematologic; they may vary widely from person to person or even from eye to eye, and it is not essential for every person with CRVO to have the same risk factors nor, conversely, will all persons with some of the same risk factors develop CRVO. Only when an eye and an individual have the critical number of risk factors required for the development of CRVO does the CRVO develop. This explains why bilateral CRVO is rare [182]. If, as is usually claimed, just one or two particular hematologic or systemic factors were causative, then surely a patient would have widespread involvement of the CRVO in both eyes and other types of retinal vein occlusion in the two eyes at least, and the retinal venous occlusive process should not be so very localized. Only when this basic concept of multifactorial causation is understood can one attach appropriate significance to the various risk factors.
In CRVO, the various risk factors may be divided into the following three categories:
1.
Local Risk Factors
Verhoeff, in 1907 [183] in his histopathological study, found that the primary cause of CRVO was endothelial proliferation in the central retinal vein. Endothelial proliferation in the central retinal vein causing narrowing of its lumen has also been reported by others [184]. Green et al. [185], based on their histopathological study of CRVO, postulated that the flow through the narrow segment of the vein within the lamina cribrosa becomes turbulent in these cases, which in turn damages the endothelium in the retrolaminar portion of the vein, thus leading to platelet aggregation and finally thrombus formation at the level of and just posterior to the lamina cribrosa. Williamson [186], based on his color Doppler imaging of the central retinal artery and vein in the region of the lamina cribrosa and optic nerve posterior to that, postulated what he called a “throttle” mechanism in the central retinal vein in the region of the lamina cribrosa. According to him, the presence of a constriction of the vein in the region of the lamina cribrosa can be inferred from the presence of higher blood velocities at this site than further back in the optic nerve. In CRVO, there may be a more uniform narrowing of the vein along its course in the nerve. Kang et al. [187] in their study reported an arterial-like appearance of the venous endothelium in the posterior lamina cribrosa, where pressure gradient forces are predicted to be greatest and the central retinal vein lumen diameter is known to be narrowest; this implicates that as a site of altered hemodynamic stress. They postulated that heterogeneity of venous endothelium may have relevance for understanding CRVO.
The other important factor is that in the center of the optic nerve, the central retinal artery and vein lie side by side enclosed in a common fibrous tissue envelope (Fig. 24.6). Klien and Olwin [189] postulated the following three occlusive mechanisms in CRVO: (a) occlusion of the vein by external compression by sclerotic adjacent structures (i.e., central retinal artery and fibrous tissue envelope) and secondary endothelial proliferation, (b) occlusion by primary venous wall disease (degenerative or inflammatory in nature), and (c) hemodynamic disturbances produced by a variety of factors (e.g., subendothelial atheromatous lesions in the central retinal artery, arterial spasm, sudden reduction of blood pressure, blood dyscrasias, etc., and further aggravated by arteriosclerosis or unfavorable anatomical relations). Possibly sclerotic changes in the lamina cribrosa may also contribute. These produce stagnation of the flow in the vein and result in primary thrombus formation in susceptible eyes. In this connection, it is relevant to take into account Virchow’s triad for thrombus formation: (i) slowing of the bloodstream, (ii) changes in vessel wall, and (iii) changes in the blood.
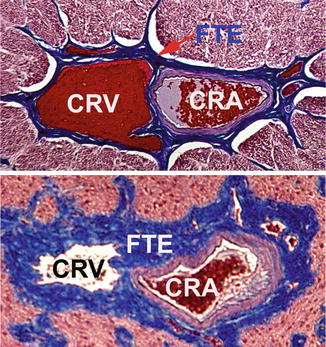
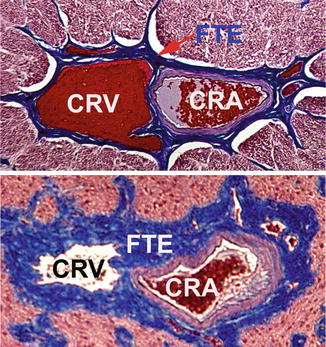
Fig. 24.6
Transverse section through the center of the intraorbital part of the optic nerve showing the central retinal artery and vein lying side by side in close apposition, enclosed by a common fibrous tissue envelope (Reproduced from Hayreh et al. [188]). Abbreviations used: CRA central retinal artery, CRV central retinal vein, FTE fibrous tissue envelope
As discussed above, it is well established that CRVO is significantly more common in patients with high intraocular pressure and glaucoma. The pressure in the central retinal vein at the optic disc depends upon the IOP, the former being always somewhat higher than the latter to maintain blood flow. A rise of IOP would produce retinal venous stasis and sluggish retinal venous outflow – one of the factors in Virchow’s triad for thrombus formation. In studies on about 700 patients with CRVO, I have also found a high incidence of CRVO in normal-tension glaucoma and in treated primary open-angle glaucoma with normal IOP. Verhoeff [155], in 1913, based on his histopathological studies, postulated that the most important factor responsible for the development of CRVO is probably the IOP compressing and collapsing the wall of the retinal vein, leading to intimal proliferation in the vein. Our morphologic studies [188] of the optic nerve, in glaucomatous optic neuropathy experimentally produced by chronic high IOP in rhesus monkeys, showed that in them, as compared to age-matched normal animals, there was a marked thickening of the fibrous tissue envelope and wall of the central retinal artery (due to arteriosclerosis), resulting in marked narrowing of the lumen of the central retinal vein (Fig. 24.6). In 1936, Jensen [190] described arteriosclerosis of the central retinal artery accompanying an obstructed vein in 20 of 23 cases of CRVO. In the optic nerve, the central retinal vein has a much lower intravascular pressure and has a very thin wall (Fig. 24.6) as compared to the central retinal artery, and those factors make the central retinal vein more vulnerable to compression and narrowing. These morphologic changes in the optic nerve may play an important role in the high incidence of CRVO in glaucoma and arterial hypertension.
As mentioned above, the site of occlusion of the central retinal vein within the optic nerve and consequently the availability of collateral channels would (Fig. 24.1) also determine the type and severity of the CRVO, i.e., the farther back the occlusion in the optic nerve, the more collaterals are available and less severe the CRVO.
2.
Systemic Risk Factors
As discussed above, in 612 patients with CRVO in my study [14], a comparison of findings in nonischemic and ischemic CRVO showed that there was a significantly greater prevalence of arterial hypertension (p = 0.025) and diabetes mellitus (p = 0.011) in the ischemic CRVO than in the nonischemic CRVO. There was no difference in ischemic heart disease, cerebrovascular disease, peripheral vascular disease, and venous diseases. As compared to a control population, there was higher prevalence of arterial hypertension (p < 0.0001) and diabetes mellitus in CRVO (in the ischemic type only, p < 0.0001). Elman et al. [11], in a cross-sectional study of 197 patients with CRVO, found a significantly higher prevalence of arterial hypertension (p < 0.03) and diabetes mellitus (<0.005) when compared to that in the National Health Interview Survey. The Eye Disease Case-Control Study Group [12] reviewed 258 patients with CRVO (84 ischemic and 148 nonischemic CRVO 26 not classified – criteria of differentiation not known) and 1,142 controls. In ischemic CRVO, they found a significant association of diabetes mellitus (p = 0.02), cardiovascular disease (p = 0.007), and arterial hypertension (p ≤ 0.001). In nonischemic CRVO, significant associated conditions were arterial hypertension (p = 0.002) and fibrinogen levels (p = 0.01). Use of estrogen in women was not found to be significant. The odds ratio for ischemic CRVO was 4.8 for arterial hypertension, 2.7 for diabetes mellitus, 2.1 for cardiovascular disease, and 2.1 for α1 globulin. The odds ratio for nonischemic CRVO was 1.8 for arterial hypertension and 1.8 for diabetes mellitus.
It is well established that arterial hypertension and diabetes cause vascular changes. Such vascular changes may occur in the central retinal artery and vein; and those changes could contribute to the development of CRVO.
3.
Hematologic Risk Factors
Thrombus formation at the site of damaged endothelium in RVO is due to aggregation of platelets and fibrin formation. Conversion of fibrinogen to fibrin involves a cascade of reactions involving a large number of clotting factors. To prevent in vivo coagulation, there is the anticoagulant system, the most important factors of which are protein C, protein S, and antithrombin III. Normal endothelium has thrombomodulin which inactivates thrombin. A complex of thrombin with thrombomodulin activates protein C, which inhibits coagulation by degrading coagulation factors Va and VIIIa [191]. However, in some persons, activated protein C does not inhibit coagulation, and this resistance to activated protein C is due to a point mutation in the factor V gene which is inherited dominantly. Injury to the endothelium alters the balance between the coagulation and anticoagulation systems and results in thrombosis. It has been postulated that imbalance of the various systems involved in this cascade can cause thrombosis. That has led to a large number of thrombophilia studies. However, as discussed above, there is no consistent evidence that one or the other thrombophilic factor is always detected on systemic evaluation in CRVO. It would seem possible that one or another of these factors may play a role locally in the lumen of the central retinal vein when there is a predisposing abnormality in the wall of the central retinal vein; however, it is not essential that those factors must be present in large amounts in the entire body, unless, of course, there is systemic venous occlusive disorder. That may explain why CRVO studies evaluating those in systemic blood do not find them.
For many of these hematologic disorders, the required treatment is anticoagulants or agents which reduce platelet aggregation. My study [149] showed that these treatment modalities increase retinal hemorrhages and adversely influence the outcome of various types of retinal vein occlusion. Not only that but I have many patients with CRVO who developed it while taking anticoagulants for other cardiovascular disorders, which clearly shows that not only do the anticoagulants have no beneficial effect but also that the systemic hematologic disorder(s) which are blamed for RVO could not have been responsible.
From this discussion of the risk factors, it emerges that in elderly persons, sclerotic changes in the central retinal artery and the fibrous tissue envelope around the central retinal vessels within the optic nerve (Fig. 24.6) and secondary endothelial proliferation in the central retinal vein cause narrowing of the lumen of the central retinal vein. Arteriosclerosis of the central retinal artery has a significant role in CRVO. This produces circulatory stasis and stagnation thrombosis. Green et al. [185], based on their histopathological study of CRVO, postulated that the flow through the narrow segment of the vein within the lamina cribrosa becomes turbulent in these cases, which in turn damages the endothelium in the retrolaminar portion of the vein, thus leading to platelet aggregation and finally thrombus formation at the level of and just posterior to the lamina cribrosa. Hematologic factors locally can also contribute to this process. Also, hemodynamic disturbances on the arterial side may play an important role in the development of this thrombosis. This is because blood flow in the retinal vessels depends upon the perfusion pressure (perfusion pressure = mean arterial blood pressure minus the venous pressure). With venous stasis, secondary to narrowing of the lumen of the central retinal vein (from partial thrombosis and/or other reasons described above), the venous pressure proximal to the site of thrombosis rises, and that results in a fall of perfusion pressure and sluggish circulation in the central retinal vein. A fall in systemic arterial blood pressure would further lower the perfusion pressure in the retinal vascular bed. My studies with ambulatory blood pressure monitoring have established that there is a significant fall of blood pressure during sleep [192, 193]. This may convert a partial thrombosis in the central retinal vein to complete thrombosis, because of poor, sluggish circulation during sleep (i.e., Virchow’s triad). I [194] and others [195] have found that many CRVO patients wake up with poor vision, strongly suggesting that nocturnal arterial hypotension plays an important role as the final insult precipitating CRVO in persons who are already susceptible. Some of these patients experience transient recurrent thrombosis of the central retinal vein before they finally develop permanent thrombosis; this is suggested by the fact that some of the CRVO patients complain of episodes of amaurosis fugax when they wake up from sleep or during the day [196] – in both cases, the vision clears after a short interval. My explanation for these episodes of amaurosis fugax is that, as the thrombus progresses to completely occlude the central retinal vein, it causes sudden stoppage of blood flow in the closed retinal circulatory system and that results in transient ischemia of the retina and associated visual loss. This sudden stoppage of venous outflow would also result in a sudden rise in the blood pressure to the arterial level in the retinal vascular bed and proximal to the site of central retinal vein thrombus. Since it is a fresh thrombus, it cannot withstand this sudden high arterial blood pressure, and it pops out, like a champagne cork, resulting in restoration of retinal circulation and normal visual function. A gradual and progressive increase in the size of the thrombus in the central retinal vein and nocturnal arterial hypotension for many hours during sleep finally, one day, produce permanent, irreversible occlusion of the central retinal vein.
In young persons, all the available evidence suggests that phlebitis of the central retinal vein is responsible for thrombosis in most cases; in them, the vitreous shows large amounts of cells, favoring an inflammatory etiology [175]. CRVO due to phlebitis has been given different eponyms including “papillophlebitis” [197], “benign retinal vasculitis” [198], “mild retinal and papillary vasculitis” [199], and “optic disc vasculitis – type II” [200].
Site of Occlusion in Nonischemic CRVO
In my study of 620 patients with CRVO, 81 % had nonischemic CRVO [182]. It seems that the site of occlusion in the central retinal vein in this type is neither in the lamina cribrosa nor in the adjacent retrolaminar region, but further back. As discussed above, the severity of the retinopathy would depend on the site of occlusion and the number of available collaterals – the farther back the occlusion, the milder the retinopathy, because of the availability of more and more collateral channels (Fig. 24.1).
Site of Occlusion in Ischemic CRVO
It seems that in this type of CRVO, the site of occlusion is most probably in the region of the lamina cribrosa or immediately posterior to that, as shown by most of the histopathological reports (since enucleated eyes invariably come from patients with ischemic CRVO and not nonischemic CRVO) [185]. When the site of occlusion in the central retinal vein is at the lamina cribrosa or just posterior to that, that leaves no collaterals (Fig. 24.1), resulting in a closed loop circulation in the retinal vascular bed. Since the central retinal artery is still pumping blood at normal arterial pressure while the vein is occluded without any collaterals, the result is complete or marked circulatory stasis and retinal ischemia. With retinal ischemia, there is also ischemic capillaropathy. As retinal circulation is restored (although sluggish), there is an associated rise in intraluminal pressure in the ischemic retinal capillaries; that ruptures the weakened ischemic capillaries and produces extensive retinal hemorrhages [194]. Ischemia of the retina is associated with marked visual loss [180].
The other mechanism of production of ischemic CRVO may be a conversion from nonischemic CRVO, as discussed below.
Conversion of Nonischemic CRVO to Ischemic CRVO
In my study [182] of 550 episodes of nonischemic CRVO, 11 % converted to an ischemic type. The cumulative proportion of episodes that converted to the ischemic type was 9.4 % within 6 months and 12.6 % within 18 months from onset of nonischemic CRVO (Fig. 24.7). When episodes were categorized by age (<45, 45–64, and ≥65 years) at onset of nonischemic CRVO, onset at 65 years or older showed a significantly higher rate of conversion to ischemic type compared to onset at younger than 45 years (p = 0.0158) and onset at 45–64 years (p = 0.0038). Of the 253 episodes, 16.2 % converted when the first onset was at 65 years or older. The cumulative proportion of conversion in this age group was 13.2 % at 6 months and 18.6 % at 18 months (Fig. 24.7). Of the 93 episodes, five converted when the first onset was at younger than 45 years. The last observed conversion for this group occurred at 18 weeks, with the cumulative proportion of conversion being 6 %. Of the 203 episodes with onset at age 45–64 years, 5 % converted; at 6 months and 18 months from onset, the cumulative proportion of conversion was 7 and 8 %, respectively.
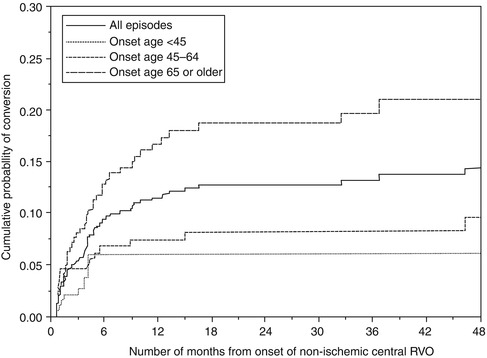
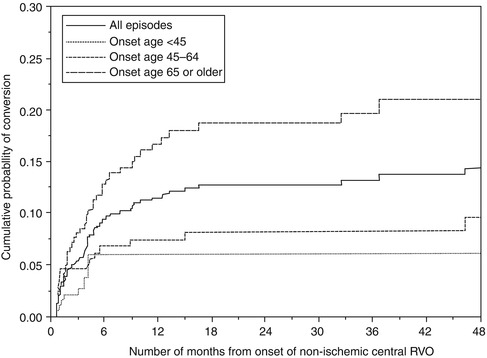
Fig. 24.7
Kaplan-Meier survival curves for cumulative probability of conversion of nonischemic CRVO to the ischemic type in the total group and also in the three age groups. Vertical axis gives cumulative probability of conversion. Horizontal axis gives the number of months from the onset of nonischemic CRVO (Reproduced from Hayreh et al. [182])
This conversion can happen either overnight or gradually. I speculate that it may be caused by two mechanisms:
1.
Blood flow in the retina depends upon perfusion pressure (arterial pressure minus venous pressure). In nonischemic CRVO, the perfusion pressure is low due to a rise in venous pressure. A further precipitous fall in perfusion pressure from marked nocturnal arterial hypotension [192, 193] would produce retinal ischemia during sleeping hours, and that may convert a nonischemic CRVO to ischemic CRVO in susceptible individuals, particularly elderly persons with cardiovascular and other systemic risk factors, taking arterial hypotensive medications. This seems to be the most frequent sequence in eyes with overnight change from nonischemic to ischemic CRVO.
2.
All the available evidence indicates that in nonischemic CRVO, the site of thrombosis is farther back in the optic nerve. In these cases, one additional factor may be gradual extension of the thrombotic process in the central retinal vein toward the optic disc up to or close to the lamina cribrosa, involving and eliminating the available collaterals in the optic nerve (Fig. 24.1) which previously protected these eyes from developing ischemic CRVO. This would convert nonischemic CRVO (usually gradually) to ischemic CRVO.
Conclusion
The above discussion shows that CRVO is a multifactorial disease, with different risk factors playing roles in its development; the different factors may act in different combinations in different eyes. Therefore, no generalization can be made. There is no one culprit causing CRVO (except in rare cases where it is associated with a specific disease (see above)).
Differentiation of Ischemic from Nonischemic CRVO
This is an important issue in the diagnosis, clinical features, and management of CRVO because of the very different visual outcome and management of ischemic CRVO which carries potentially serious blinding complications. Although the concept of the two types of CRVO is now widely accepted, the criteria for their diagnosis and the differentiation between the two types are still highly controversial. I [13] conducted a prospective study in 128 consecutive untreated patients (140 eyes) to determine the criteria for differentiating the two types of CRVO. I evaluated the usefulness of the following six routine, clinical tests in differentiating between the ischemic and nonischemic types:
A.
Functional tests: (1) visual acuity, (2) visual fields, (3) relative afferent pupillary defect (RAPD), and (4) electroretinography (ERG)
B.
Morphologic tests: (1) ophthalmoscopy and (2) fluorescein fundus angiography
My objective was to assess the sensitivity and specificity of these clinical tests, to help us differentiate the two types of CRVO at the onset or during the very early stages. It is during these early stages that these patients are seen and one has to decide upon their management. Natural history studies on CRVO have shown that retinopathy in CRVO is not a stationary phenomenon but is constantly evolving [194]. The findings from these six tests, therefore, must be analyzed in relation to the duration of the CRVO since onset. For this reason, I divided the cases into three groups: group I, examined within 90 days after the onset of CRVO; group II, eyes examined 91–365 days after the onset; and group III, whose CRVO had developed more than 1 year before examination for this study. I felt this grouping satisfactorily represented the early (group I), intermediate (group II), and late (group III) phases of the disease.
The following parameters for the six tests were analyzed:
1.
Visual acuity: This was tested by Snellen visual acuity chart.
2.
Visual fields: This was tested in visual fields plotted with a Goldmann perimeter. For each of the three isopters (i.e., I-2e, I-4e, and V-4e) plotted, the analysis was done whether they were normal or showed the presence of central scotoma and/or peripheral visual field defect or whether one or more targets were not seen.
3.
Relative afferent pupillary defect: This was measured by using the “swinging flashlight test” [201]. Neutral density photographic filters were placed in front of the normal eye until the pupillary responses were equal in both eyes. Filters used were 0.3, 0.6, 0.9, 1.2, and 2.1 log units.
4.
Electroretinography: Data on scotopic and photopic implicit time and amplitude of a- and b-waves were analyzed [202].
5.
Ophthalmoscopy: The extent of retinal hemorrhages, cotton-wool spots, macular and optic disc edema, and preretinal hemorrhages were evaluated.
6.
Fluorescein fundus angiography: The following parameters were analyzed in the angiograms. Retinal capillary obliteration or nonperfusion was graded. Other angiographic parameters included were fluorescein leakage, microaneurysms and other intraretinal microvascular abnormalities, optic disc and retinal neovascularization, macular retinal pigment epithelial degeneration, submacular choroidal neovascular membrane, and optic disc venous collaterals.
The results of this study are discussed at length elsewhere [13]. Following is a brief summary.
Visual Acuity: Best sensitivity and specificity for the differentiation of ischemic from nonischemic CRVO for the entire sample and for group I (<90 days) was associated with a visual acuity of 20/200 or worse.
Visual Fields: For differentiation of ischemic from nonischemic CRVO, in visual fields tested with Goldmann perimeter, the following were the best parameters to differentiate:
(a)
Could not see I-2e but could see I-4e and V-4e targets in group 1 and 2 – p-value <0.001
(b)
Any defect with V-4e – p-value <0.001
(c)
Central scotoma and peripheral defect with I-4e – p-value <0.001
(d)
A central scotoma with V-4e – p-value <0.001
A common objection by many ophthalmologists to the use of manual kinetic perimetry performed with a Goldmann perimeter in my study is that now automated perimetry is the standard method to plot visual fields and not kinetic perimetry. But automated perimetry (Humphrey 30-2 or 24-2 SITA) provides information only up to about 24–30° in the periphery. Kinetic perimetry, by contrast, provides peripheral visual field information all the way to about 80–90° temporally, 70° inferiorly, 60–70° nasally, and 50–60° superiorly. Thus, in ischemic CRVO, the visual fields plotted with manual kinetic perimetry provide information far superior to automated perimetry about the peripheral visual field defects, for two reasons: (i) for evaluating visual functional disability, because peripheral visual fields are essential for “navigation” and day-to-day living in spite of central scotoma, which is invariably present in ischemic CRVO, and (ii) also for differentiation of the two types of CRVO. It is unfortunate that kinetic perimetry is mostly no longer used to evaluate visual field defects.
Relative Afferent Pupillary Defect: In this study, the sensitivity and specificity of the RAPD for the detection of ischemic CRVO were found to be 88 and 90 %, respectively, when RAPD of >0.70 log units was used as the cutoff point, and 80 and 97 %, respectively, when RAPD of ≥0.90 log units was used as the cutoff point [202]. Duration of the CRVO had no statistically significant influence on RAPD.
Electroretinography: For differentiating ischemic from nonischemic CRVO, in both the photopic and the scotopic ERG, the b-wave amplitude had a sensitivity of 80–90 % and a specificity of 70–80 %; the b-/a-wave amplitude ratio, a sensitivity of 60–70 % and a specificity of 70 %; the a-wave amplitude, a sensitivity of 60–70 % with a specificity of 60–70 %; the a-wave implicit times, 28–55 and 70–80 % for sensitivity and specificity, respectively; and the b-wave implicit time, 35–52 % sensitivity and 70–80 % specificity. Analyses of data revealed no statistically significant influence of duration of the CRVO on the various ERG parameters.
Table 24.3 summarizes the sensitivity and specificity of these four functional tests to differentiate ischemic from nonischemic CRVO at onset.
Table 24.3
Sensitivity and specificity of the four functional tests in ischemic CRVO
Ischemic CRVO | |||
---|---|---|---|
Functional tests | Sensitivity (%) | Specificity (%) | |
Visual acuity | ≤20/400 | 91 | 88 |
Peripheral visual field | I-2e not seen | 97 | 73 |
Defective V-4e | 100 | 100 | |
Relative afferent pupillary defect | ≥0.9 log units | 80 | 97 |
Electroretinography | b-wave amplitude <60 % | 80 | 80 |
Ophthalmoscopy: The only parameters to give reasonable sensitivity and specificity were retinal hemorrhages (particularly hemorrhages in the macular and posterior retina) during the first 3 months, marked macular edema during the 3- to 12-month period, and retinal venous sheathing after 1 year.
Fluorescein fundus angiography: Since most ophthalmologists use this test to differentiate ischemic from nonischemic CRVO, a detailed discussion of its pros and cons is called for. Fluorescein fundus angiography during the acute, early phase of CRVO did not provide adequate information in at least one third of the eyes in my study, because of extensive retinal hemorrhages, unsatisfactory angiograms, or our inability to perform angiography due to media opacities or other reasons. It is important to point out that isolated, small, focal capillary obliteration in nonischemic CRVO was seen in 9.5 % in the macular region and in 6.4 % elsewhere in the retina in that study.
For differentiation of ischemic from nonischemic CRVO, retinal capillary obliteration is the fundamental information required from angiography. During the early stages of CRVO, angiography has many serious limitations in providing this information; these include extensive retinal hemorrhages, poor-quality angiograms, and inability to perform angiography for a variety of reasons. Even when retinal capillaries are satisfactorily seen on fluorescein angiograms, the findings may be misleading, for the following reasons:
1.
Retinal capillary obliteration is a progressive phenomenon. Available evidence indicates that it takes at least 3–4 weeks, if not longer, after the development of ischemic CRVO for retinal capillaries to develop obliteration [174, 184, 203]. So if a patient is seen during the first 2–3 weeks after the onset of ischemic CRVO, fluorescein fundus angiography may show perfectly normal filling of the retinal capillaries in spite of retinal ischemia (examples demonstrating this phenomenon are shown in Figs. 24.8 and 24.9). I have seen a few eyes with mild ischemic CRVO in my natural history studies that developed slowly progressive retinal capillary obliteration many months or even years after the onset of CRVO (Figs. 24.10 and 24.11). Under these circumstances, the presence of normal retinal capillary perfusion initially may mislead one into believing that one is dealing with nonischemic CRVO when it is in fact ischemic CRVO.
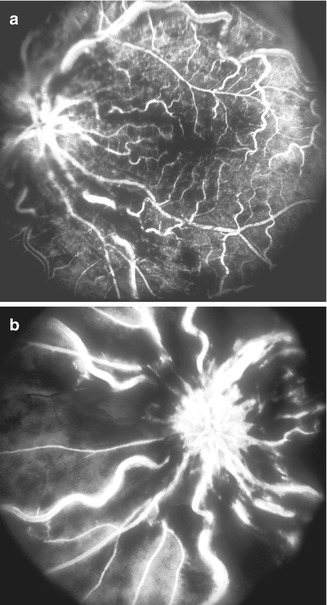
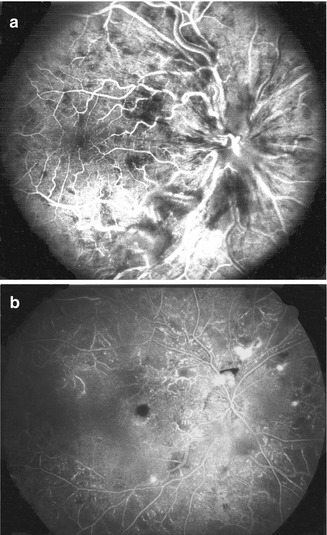
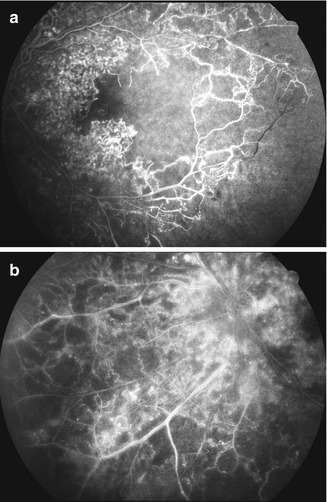
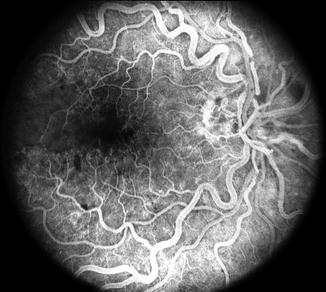
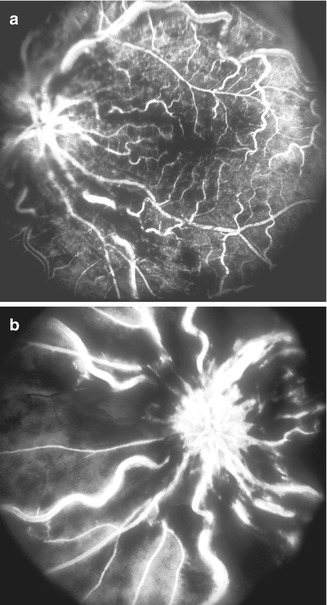
Fig. 24.8
Left eye with ischemic CRVO at initial visit (a) showed intact retinal capillaries on angiography. (b) shows extensive retinal capillary obliteration when seen 2¼ months later (Reproduced from Hayreh [176])
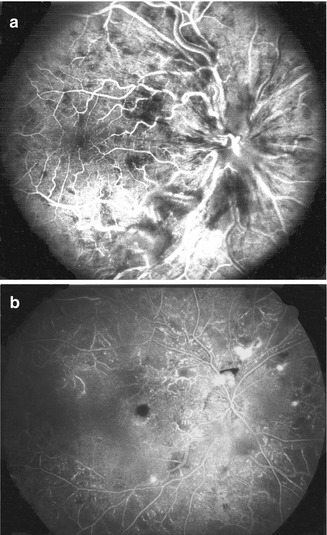
Fig. 24.9
Right eye with ischemic CRVO at initial visit (a) showed intact retinal capillaries on angiography. (b) shows development of extensive retinal capillary obliteration later on (Reproduced from Hayreh [176])
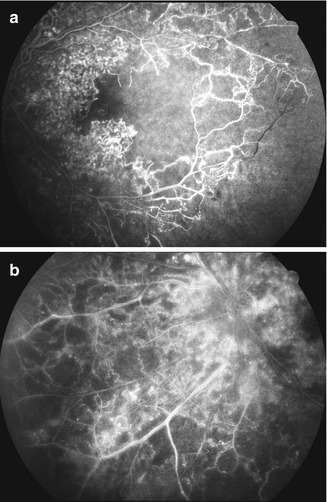
Fig. 24.10
Left eye of a 63-year-old woman, who initially started with nonischemic CRVO, which gradually converted to ischemic type. Fluorescein fundus angiograms (a, b) 5 years after the onset of nonischemic CRVO show extensive retinal capillary obliteration. Note in (a) a triangular pattern of capillary obliteration involved the macular region that extended to the periphery
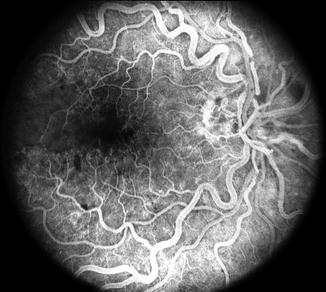
Fig. 24.11
Right eye of a 72-year-old man, who initially started with nonischemic CRVO, which gradually converted to ischemic type. Fluorescein angiogram 10 months after the onset of CRVO shows a good example of a triangular pattern of retinal capillary obliteration involving the macular region that extended to the periphery, with sheathed veins in that region
2.
It is well established now that in various retinopathies associated with retinal capillary obliteration, e.g., diabetic retinopathy, ischemic CRVO, branch retinal vein occlusion, and others, the retinal capillary obliteration starts first in the peripheral retina and then slowly progresses toward the posterior pole. In most ophthalmologists’ offices, standard fluorescein fundus angiography covers only the central 30° or 60° of the posterior pole, i.e., the optic disc and macular region (Figs. 24.12a and 24.13a). Although a standard angiogram with a 60° camera covers a much larger area, it may still provide no information about the peripheral retina (Figs. 24.12b and 24.13b). Magargal et al. [203] calculated an “ischemic index” from fluorescein angiograms covering a 30° or 45° area of the posterior pole and used that as the primary criterion to differentiate CRVO into the ischemic and nonischemic types. But this “ischemic index” would give no information about the peripheral retinal capillary nonperfusion.
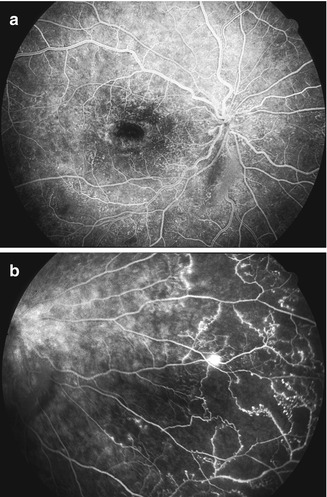
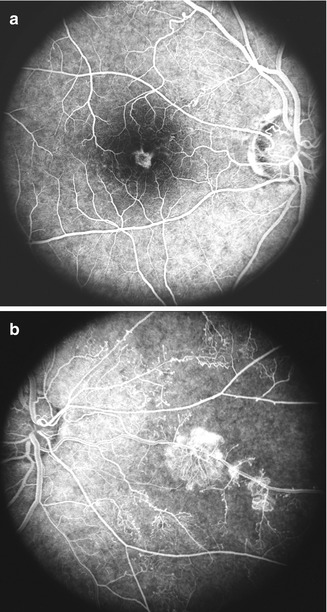
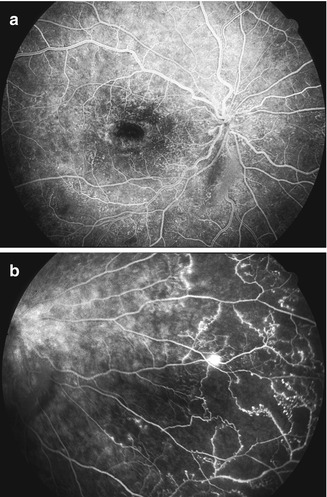
Fig. 24.12
Fluorescein fundus angiograms of a right eye with ischemic CRVO 25 months after onset, (a) shows no retinal capillary obliteration in the posterior pole, but the nasal periphery (b) shows extensive retinal capillary obliteration with retinal neovascularization. At initial visit, the eye showed no evidence of retinal capillary obliteration (Reproduced from Hayreh et al. [13])
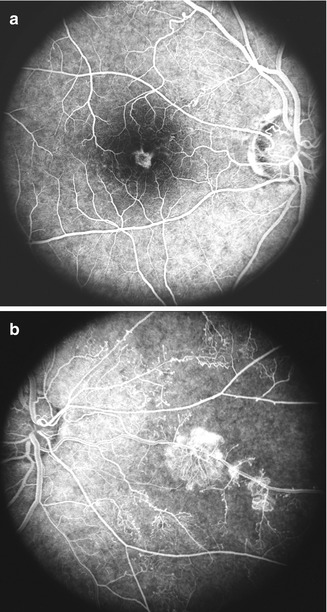
Fig. 24.13
Fluorescein fundus angiograms of the right eye of a 38-year-old man with ischemic CRVO show in (a) almost normal-looking capillary network, but in (b) there is extensive retinal capillary obliteration in the nasal retina, with retinal neovascularization
3.
Welch and Augsburger [204] studied the reproducibility and accuracy of eight retinal specialists in assessing the extent of retinal capillary nonperfusion on fluorescein angiography in CRVO and found that the proportion of agreement with the correct classification was less than 60 % for all the specialists. Johnson et al. [205], in a forced choice analysis of ophthalmologists to decide which fluorescein angiograms belonged to the neovascular group in their CRVO patients with and without iris neovascularization, found that only 36 % of patients who developed iris neovascularization could be distinguished from those who did not. This shows that even if there are perfectly satisfactory angiograms, there are problems in their evaluation.
A test which provides reliable information in only 50–60 % of cases is clinically unreliable and not always useful during the early, acute phase of CRVO when this information is critical; however, one must point out that once the required information on retinal capillary nonperfusion is available, fluorescein fundus angiography is an extremely helpful test with a high sensitivity and specificity.
Overall Order of Reliability of Various Tests
Thus, my study [13] showed that RAPD is a highly reliable test in uniocular CRVO (with a normal fellow eye), followed closely by ERG in all cases, and the combined information from these two objective tests can make such a differentiation in almost all cases. Perimetry, followed by visual acuity, proved to be the next most reliable parameters. Since fluorescein fundus angiography, when used during the early, acute phase, could not provide satisfactory information on retinal capillary obliteration in at least one third of the patients (and in some of the rest the information supplied was misleading), it performed much worse overall than any of the functional tests; however, when angiography gave definite data on substantial retinal capillary obliteration, it was an extremely useful indicator of ischemic CRVO. I have found the ophthalmoscopic appearance to be the least reliable, most misleading parameter, because of its constantly changing pattern [194]. It is evident from the above discussion that functional tests are more useful than morphologic tests.
Should Ocular Neovascularization Be Used as an Endpoint?
My study [206] of 673 nonischemic and 239 ischemic CRVO eyes showed that in ischemic CRVO, within 6 months from the time of onset, the cumulative probability of development of iris neovascularization was 49 %, angle neovascularization 37 %, neovascular glaucoma 29 %, retinal neovascularization 9 %, and disc neovascularization 6 % (see below). (Neovascularization was not seen in any eye with nonischemic CRVO.) Thus, not all eyes with ischemic CRVO develop neovascularization.
Misconception that Fluorescein Fundus Angiography Is the Best Test to Differentiate Ischemic from Nonischemic CRVO and No Other Test Is Required
As discussed above, for differentiation of ischemic from nonischemic CRVO, the fundamental information required from fluorescein angiography is the extent of retinal capillary obliteration. My study showed that, during the early stages of CRVO, fluorescein angiography provides reliable information on differentiation in at best only 50–60 % of cases, because of various limitations discussed above – that makes this clinically an unsatisfactory test.
There is a further serious problem with the use of retinal capillary nonperfusion in the diagnosis of ischemic CRVO. In the literature, a 10-disc area of retinal capillary obliteration on fluorescein fundus angiography has become the gold standard to differentiate the two types of CRVO [207–210]. But my study showed that this is not a valid criterion at all. The presence of isolated, small, focal retinal capillary obliteration is compatible with nonischemic CRVO. I discussed at length the flaws in using this criterion of “10-disc area of retinal capillary obliteration” [211]. The results of a large multicenter CRVO study [207] supported my conclusions; that study showed that eyes with less than 30 disc diameters of retinal capillary nonperfusion and no other risk factor are at low risk for developing iris/angle neovascularization (i.e., ischemic CRVO), “whereas eyes with 75 disc diameters or more are at highest risk.” Thus, 10-disc area of retinal capillary obliteration on fluorescein angiography is a totally unreliable parameter in differentiating ischemic from nonischemic CRVO during the early stages – the time when one needs that information. It can result in incorrect diagnosis, prognosis, and management. However, unfortunately, “10-disc area of retinal capillary obliteration” still continues to be used and propagated, causing unreliable results and further controversy.
Demographic Characteristics
Several studies have reported on the demographic characteristics of CRVO. I investigated this; the findings are discussed at length elsewhere [182]. Briefly, there were 500 nonischemic and 184 ischemic CRVO patients – a ratio of 73 % versus 27 %, respectively. In nonischemic CRVO, there were 56 % males, and age range at onset was 14–88 (median 59) years in males and 15–89 (median 61) years in females; in the entire group of nonischemic CRVO, 18 % were young (<45 years), 37 % middle aged (45–64 years), and 44 % elderly (≥65 years). In ischemic CRVO, there were 54 % males, and age range at onset was 27–88 (median 66) years in males and 16–92 (median 71.5) years in females; in the entire group of ischemic CRVO, 7 % were young, 25 % middle aged, and 68 % elderly. This shows that (i) no age is immune to CRVO, contradicting a prevalent impression that CRVO does not develop in young persons and (ii) ischemic CRVO is more common in the older age group than nonischemic CRVO. Compared to men, there was a higher (p < 0.001) proportion of women in the elderly age group than in other age groups. There was no evidence that CRVO developed more commonly in one side or the other.
There is strong evidence to suggest that nonischemic CRVO may be even more common than what my study [182] found, because a number of nonischemic CRVOs may remain asymptomatic and resolve spontaneously, without the patient ever consulting an ophthalmologist. For example, retinociliary venous collateral vessels on the optic disc (a footprint of CRVO) are often found during a routine ophthalmic evaluation in totally asymptomatic patients or in glaucoma patients. That the ratio of nonischemic to ischemic CRVO is 3:1 is highly important clinically, because nonischemic CRVO is a comparatively benign disease, whereas ocular neovascularization and other seriously blinding complications are found only in ischemic CRVO. The Kaplan-Meier estimate of the cumulative proportion of eyes that developed a second episode of nonischemic CRVO in the same eye is 0.9 % within 2.5 years and 2.2 % within 5 years from onset of the first episode. The Kaplan-Meier estimate of the cumulative proportion of eyes that developed nonischemic CRVO in the fellow eye is 6.6 % within 2 years from onset in the first eye. This completely contradicts the conclusion of Williamson [212] that “CRVO causes severe impairment of vision in a significant proportion of patients because of the frequent bilateral disease.”
Seasonal Variations in the Onset of CRVO
This has been reported in some studies. Lavin and Dhillon [213], in a retrospective study of 105 CRVO patients from Britain, found a significantly (p < 0.001) higher incidence during the 6-month period from September to February than for the 6-month period from March to August. They stated that variation in onset of CRVO was very similar to the variations in seasonal temperature. The seasonal variation in their study was similar for both ischemic and nonischemic CRVO. Peduzzi et al. [214] reported from a retrospective study of 127 Italian patients with “retinal vein occlusion” that the incidence of retinal vein occlusion was significantly higher (p < 0.005) during the cold months (42.5 %) than during the warm (26.7 %) and intermediate (30.7 %) months, with no significant difference between the two latter groups. Ho et al. [215] from Taiwan in a retrospective study reported a significant seasonality, with a peak in January for each age group and each gender group, as well as for the total sample.
In view of these reports, I investigated seasonal variation in the development of CRVO in 439 ischemic and 179 nonischemic CRVO patients or 653 (467 nonischemic and 186 ischemic) CRVO eyes seen in my clinic [216]. By calendar months, distribution of occurrences of onset is shown in Fig. 24.14 and chi square goodness-of-fit test statistic and corresponding p-value. For each of the different types of CRVO, the test results showed no significant departure from the assumed model of equal rate of occurrence of CRVO. The other analyses, by quarters of the year, by seasons of the year, and by temperature, showed similar results.
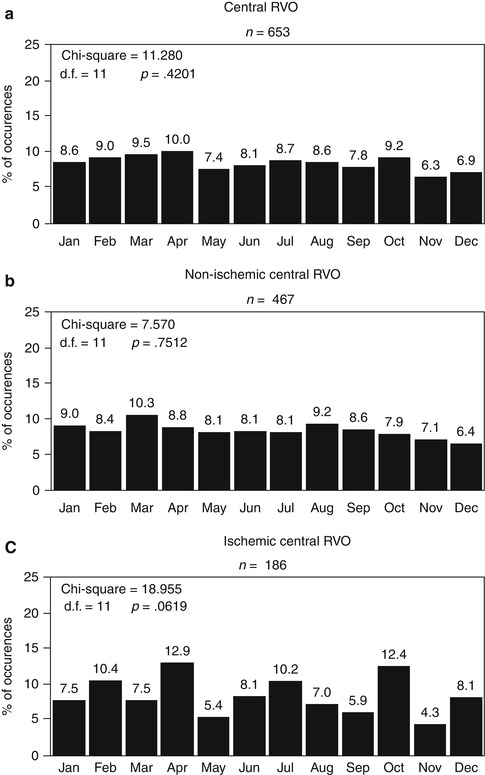
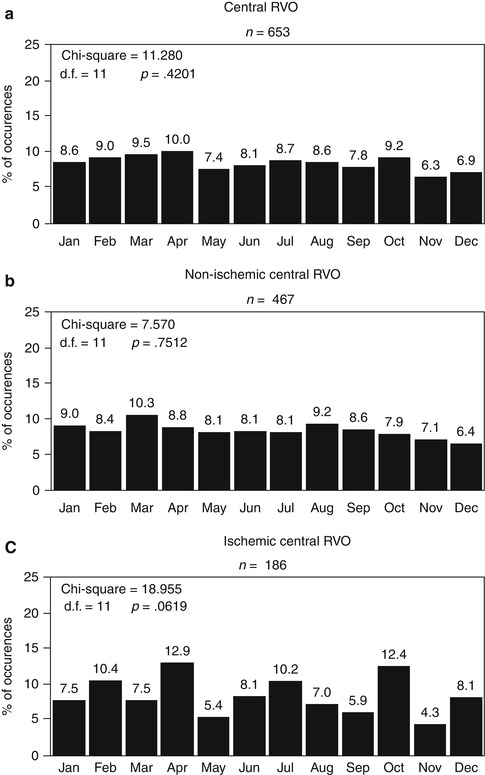
Fig. 24.14
The distribution of occurrence of onset of CRVO by calendar months with chi square of goodness-of-fit test statistic and corresponding p-value. (a) is of all CRVOs, (b) of nonischemic CRVO, and (c) of ischemic CRVO (Reproduced from Hayreh et al. [216])
My study [216] does not bear out the finding of seasonal variation in the development of CRVO reported in two previous studies [213, 214]. The study by Lavin and Dhillon [213] was from London, England, and based on retrospective review of information from 105 cases of CRVO. The report by Peduzzi et al. [214] was from Modena, Italy, and based on a retrospective study of 127 patients with “retinal vein occlusion” – the authors give no information about the number of various types of retinal vein occlusion included in their study. Lavin and Dhillon [213] argued that a seasonal incidence of CRVO may be comparable with thrombotic lesions in other organs, because myocardial infarctions and cerebrovascular accidents are more frequent in winter than other seasons and show close correlations with temperature [217–222] and that cold temperature is associated with an increased risk of thrombosis [219].
It could conceivably be argued that differences in living conditions in Britain and Italy, on the one hand, and in the Midwestern United States, on the other, could be responsible for my not finding any seasonal variation. Such a hypothesis is, however, hard to maintain. The winter temperatures in Iowa are much lower (average: high −1 to +8°C and low −1 to −11°C), for much longer, than in London (England) (average: high 7–11°C and low 2–8°C) or Modena (Italy) (average: high 9 to l4°C and low 2–8°C). Buildings and cars which are well heated during winter are universal in Iowa compared with the other two countries, so that the indoor temperature in Iowa is likely to be much warmer than in Britain and Italy during winter. It might be just possible to claim that this difference is responsible for my not seeing any seasonal variation. This possibility has also been suggested by a study indicating that the lowest seasonal variation occurs in countries with very cold winters (e.g., Scandinavia) which would imply that insulation and indoor heating may be preventive factors [223]. However, it is hard to find much support for this argument. A British study found that elderly people living in centrally heated warden-controlled accommodation showed similar seasonal variation in mortality rates to those living in their own (non-centrally heated) homes [224]. Also, I can attest from years of residence in Britain and Iowa that the British and other Europeans dress much more warmly at home than Iowans do, so that the effect of central heating in Iowa would be counterbalanced by the warmer clothing in Europe. Moreover, the hypothetical beneficial effects of central heating in Iowa would be counteracted by the extreme cold often experienced when a person goes outdoors during winter, whether of necessity or for recreation, as was also pointed out by Keatinge [224]. Further, Keatinge et al. [225] in their study in England and Wales found that with an increase in proportion of the households with central heating from 13 to 69 % from 1964 to 1984, there was no significant decrease in excess winter mortality from coronary and cerebrovascular disease. The study by Stout and Crawford [222] from Belfast, Northern Ireland, suggested that central heating there may not greatly increase minimum indoor temperature or influence core body temperature at all. The centrally heated accommodation in Iowa therefore cannot explain the marked disparity between my results and those of the other two studies. A more likely explanation of the disparity of the results between my study and the two European studies lies in their relatively small samples and the retrospective nature of their data collection, while my study was a prospective study in a large cohort of patients.
Conclusion
My prospective study on a large sample of CRVO showed no seasonal variation in its development.
Clinical Features
Diagnosis of CRVO is not difficult, because it has classical clinical features. The main problem is differentiation of nonischemic from ischemic CRVO. This differentiation is crucial for the correct management of CRVO because of the very high risk of much more serious complications, including blindness, following ischemic CRVO. The differentiation is discussed above.
Symptoms
Patients with nonischemic CRVO may have no symptoms, and it may be detected as an incidental finding on a routine ophthalmic examination. Retinal venous stasis with mild retinal hemorrhages per se is asymptomatic. Almost invariably, it becomes symptomatic only when there is involvement of the foveal region by development of macular edema and, rarely, hemorrhages.
In nonischemic CRVO, when a visual symptom is reported, it may be vague blurring of vision, usually involving the central vision, with normal peripheral vision. The blurring is usually more marked on waking in the morning, improving to a variable extent after a few hours or in the afternoon [194]. The onset of symptoms is usually gradual because of gradually progressive macular edema, but they may be discovered suddenly on waking one morning. It is not rare for a patient to complain of episodes of amaurosis fugax before the onset of constant blurring. In my study of 864 eyes with CRVO, it occurred in 5 % [196]. I found that during episodes of amaurosis fugax, the patient may complain of a purple blur, particularly when nonischemic CRVO is associated with cilioretinal artery occlusion (see below). In my study of 37 eyes with CRVO associated with cilioretinal artery, the prevalence of amaurosis fugax was 38 % [196]. In nonischemic CRVO alone, these episodes of amaurosis fugax represent intermittent complete occlusion of the central retinal vein, as discussed previously.
In ischemic CRVO, on the other hand, there is always marked deterioration of vision; this is frequently noticed suddenly on waking one morning, though not always. As in nonischemic CRVO, some patients may complain of episodes of amaurosis fugax before the development of constant visual blurring. When nonischemic CRVO changes to the ischemic type, there may be sudden worsening of vision, or it may get worse only gradually.
Visual Status in CRVO
I investigated this in 667 consecutive patients (697 eyes) with CRVO seen in my clinic. Visual fields were plotted with a Goldmann perimeter. The findings are discussed at length elsewhere [180], and here is a brief account.
Initial Visual Acuity and Visual Fields
Visual acuity and visual field findings at the initial visit, from time of onset of visual loss, are given in Table 24.4. Comparing visual status at the initial visit showed a significantly worse initial visual acuity and visual field defect in eyes with ischemic CRVO compared to those with nonischemic CRVO (both p < 0.0001). Of the eyes first seen within 3 months of onset, initial visual acuity of 20/200 or worse was observed in 99 % of eyes with ischemic CRVO and 22 % in those with nonischemic CRVO.
Table 24.4
Visual acuity and visual field at initial visit by time from onset of visual loss
Visual assessment | Time from onset of visual loss to initial visit | ||
---|---|---|---|
≤3 months | >3–6 months | >6–12 months | |
Nonischemic CRVO | |||
Visual acuity | (n = 492 eyes) | (n = 70 eyes) | (n = 26 eyes) |
20/15–20/20 | 115 (23 %) | 9 (13 %) | 6 (23 %) |
20/25–20/30 | 86 (18 %) | 7 (10 %) | 1 (4 %) |
20/40–20/60 | 122 (25 %) | 18 (26 %) | 3 (12 %) |
20/70–20/100 | 61 (12 %) | 17 (24 %) | 7 (27 %) |
20/200–400 | 96 (20 %) | 16 (23 %) | 6 (23 %) |
CF or worse | 12 (2 %) | 3 (4 %) | 3 (12 %) |
Visual field defect | (n = 478 eyes, 14 missing) | (n = 69 eyes, 1 missing) | (n = 25 eyes, 1 missing) |
Minimal | 321 (67 %) | 40 (58 %) | 18 (72 %) |
Mild | 117 (24 %) | 19 (28 %) | 5 (20 %) |
Moderate | 38 (8 %) | 7 (10 %) | 2 (8 %) |
Marked | 2 (0.4 %) | 2 (3 %) | 0 (0 %) |
Severe | 0 (0 %) | 1 (1 %) | 0 (0 %) |
Ischemic CRVO as first diagnosis | |||
Visual acuity | (n = 85 eyes, 3 missing) | (n = 13 eyes, 2 missing) | (n = 5 eyes, 1 missing) |
20/15–20/20 | 0 | 0 | 0 |
20/25–20/30 | 0 | 0 | 0 |
20/40–20/60 | 0 | 0 | 0 |
20/70–20/100 | 1 (1 %) | 1 (8 %) | 0 |
20/200–400 | 18 (21 %) | 2 (15 %) | 1 (20 %) |
CF or worse | 66 (78 %) | 10 (77 %) | 4 (80 %) |
Visual field defect | (n = 80 eyes, 8 missing) | (n = 13, 2 missing) | (n = 5, 1 missing) |
Minimal | 0 | 0 | 1 (20 %) |
Mild | 6 (8 %) | 0 | 0 |
Moderate | 39 (49 %) | 3 (23 %) | 0 |
Marked | 32 (40 %) | 8 (62 %) | 4 (80 %) |
Severe | 3 (4 %) | 2 (15 %) | 0 |
Forty-four percent of eyes with ischemic CRVO had an initial visual field defect grade of marked to severe, compared to 0.4 % of eyes with nonischemic CRVO. With V-4e isopter, 55 % of the eyes seen within 3 months of onset with ischemic CRVO presented with scotoma, compared to only 5 % of the eyes with nonischemic CRVO (p < 0.0001). Most of the scotomas were central scotoma, which was found in 41 % of eyes with ischemic CRVO and 4 % of eyes with nonischemic CRVO. For the peripheral visual field, I-2e target was seen by 89 % of the eyes with nonischemic CRVO compared to only 7 % of the eyes with ischemic CRVO and I-4e target by 99 and 74 %, respectively. Peripheral visual field defect was present in 3 % of eyes with nonischemic CRVO and in 17 % of eyes with ischemic CRVO. For ischemic CRVO, the type of peripheral visual defect most frequently observed was peripheral inferior nasal defect, which was present in only 10 % of the eyes.
This shows that a distinction between the two types of CRVO is crucial and fundamental in determining the visual outcome of CRVO. It is most unfortunate that this basic fact has been ignored in the vast majority of studies dealing with the visual outcome in CRVO.
This wide difference in visual outcomes between the two types of CRVO is not at all surprising if one considers the basic facts about the pathogenesis of visual loss in the two types. The primary cause of poor visual acuity in nonischemic CRVO is macular edema, while in ischemic CRVO, retinal ischemia is usually the major factor, with macular edema a minor factor. Macular retinal ganglion cells are most vulnerable to ischemic damage [226]. It is well known that the macular region has more than one layer of retinal ganglion cells, unlike the rest of the retina, and it is the thickest part of the retina – maximum thickness being close to the foveola. Experimental [226, 227] and clinical [228] studies on central retinal artery occlusion have shown that ischemic damage is most marked in the central part of the macular retina, which results in the development of central scotoma, most commonly the only visual field defect seen in patients following transient central retinal artery occlusion [228]. Once the retinal ganglion cells in the macular region have suffered irreversible ischemic damage, there is little chance of any visual acuity improvement, even if the macular edema resolves, since it is not the primary cause of poor visual acuity in these cases. This is evident from the fact that, in my study, resolution of macular edema had a significant effect on visual improvement in nonischemic CRVO (visual acuity, p < 0.0001; visual fields, p = 0.037) but not in ischemic CRVO (visual acuity, p = 0.55; visual fields, p = 0.83).
Natural History of Visual Outcome in CRVO
Understanding the natural history of a disease is paramount to its management. If it is not understood, natural recovery may be attributed to a treatment which is actually ineffective. Although the clinical entity of CRVO has been known since 1878 [2, 229] and it is a common, visually disabling disorder, there is little firm information in the literature on the natural history of its visual outcome. Those few reports in the literature which deal with the natural history of visual outcome in CRVO [230–239] have definite flaws. First, it is well established now that CRVO is of two types: nonischemic and ischemic CRVO (see above), with very different outcomes. In all the previous natural history studies, either the two clinical entities were lumped together into one category or the criteria used to differentiate CRVO into its two types were inadequate, such that the findings are based on a mixture of the two types, which makes the results unreliable. Second, the retinopathy in CRVO involves the entire retina and not only the macular region. Unfortunately, all the previous CRVO studies dealt only with visual acuity, without any data on the outcome of visual fields, which provide information about the function of the entire retina. Although visual acuity is critical in fine work, the peripheral visual fields are equally, if not more, essential for normal “navigation” and day-to-day living. Following is a brief review of the previous studies on the natural history of visual outcome in CRVO.
Moore [230], in his study of 18 eyes with CRVO mostly followed for several years, found almost invariably marked deterioration in visual acuity. It seems that most of his cases had ischemic CRVO. He recorded the visual fields in his cases and commented: “Perhaps the most striking fact with regards to the field of vision in central vein thrombosis is the relative frequency with which the peripheral fields are but little constricted, and this is true not only when they were taken within a short time of the thrombosis but they may remain nearly full for many years.” This is supported by my study and is an important finding.
Zegarra et al. [231] in a study of 25 eyes, followed for 1–8 years, found that in 10 eyes with “nonischemic CRVO,” the final visual acuity was 20/30 or better in 50 % and 20/60 in 30 %, while among the “ischemic CRVO” eyes, 82 % had a final VA of 20/400 or less.
Quinlan et al. [232] in a retrospective study of 107 nonischemic and 61 ischemic CRVO eyes (using only the criterion of ≥5-disc diameter area of capillary nonperfusion to differentiate the two types of CRVO) and a follow-up of 6 months to 6 years (mean 22 months) reported the following: in “nonischemic CRVO” eyes, initial visual acuity varied from 20/15 to counting fingers; among them, in eyes with initial visual acuity of 20/40 or better, the final visual acuity was 20/200 or less in 21 %, and in those with initial visual acuity of 20/200 or less, it was 20/200 or less in 88 %. In this group, 15 % improved by 3 or more lines from the baseline, and 31 % lost 3 or more lines. In all eyes with “ischemic CRVO,” initial visual acuity was 20/100 or less; the final visual acuity was 20/200 or less in 93 %, counting fingers or less in 54 %, and hand motion or less in 36 %. They concluded that good initial visual acuity provided no guarantee of a satisfactory visual outcome.
Chen et al. [233] in a case series of 59 eyes with “nonischemic CRVO,” followed for at least 1 year (average 2.5 years), found that visual acuity improved by two or more lines in 15 %, remained stable in 56 %, and decreased in 29 %. They concluded that “nonischemic CRVO” frequently results in significant, permanent visual loss. They also found that initial visual acuity had no predictive value for progression.
The multicenter Central Vein Occlusion Study Group [237] reported their results on visual acuity in 714 eyes with CRVO. The only criterion used to classify their cases into ischemic CRVO was the presence of at least a 10-disc area of retinal nonperfusion [238]. However, to evaluate the natural history of visual outcome, they combined their ischemic and nonischemic CRVOs into one group. They divided their cases into three groups based on initial visual acuity: group 1 with visual acuity of 20/40 or better (29 %), group 2 with acuity between 20/50 and 20/200 (43 %), and group 3 with acuity worse than 20/200 (28 %). The final visual acuity in group 1 was stable in 65 % and deteriorated in 35 % – in 10 % to worse than 20/200; in group 2, it improved to better than 20/40 in 19 %, stayed the same in 44 %, and deteriorated to worse than 20/200 in 37 %; and in group 3, in 79 % it was less than 20/200, in 19 % improved to 20/50 to 20/200, and in 1 % to 20/40. They concluded that “Visual acuity at baseline is a strong predictor of visual acuity at 3 years” for eyes in group 1 and 3 but a poor predictor for group 2.
In a SCORE study [239], where all CRVO eyes were combined without any differentiation into ischemic and nonischemic, in the 73 eyes without any treatment, at 12 months of follow-up, visual acuity improved in 26 %, remained the same in 19 %, and deteriorated in 55 %.
In my study [180], I categorized patients into ischemic and nonischemic CRVO based on combined information from the functional and morphologic tests discussed above. A detailed account of the changes in visual acuity and visual fields in my study during follow-up is discussed elsewhere [180]. Table 24.5 summarizes the data on visual acuity and visual fields achieved at 15 months and 2–5 years of follow-up and overall final follow-up in eyes without macular edema. Final visual acuity and visual field defect at the final visit after macular edema had resolved showed worse visual acuity and visual field defect in eyes with ischemic CRVO compared to those with nonischemic CRVO (both p < 0.0001; Table 24.5). There were 85 % of the ischemic CRVO eyes with visual acuity of 20/200 or worse compared to 17 % of those with nonischemic CRVO. There were 50 % of eyes with ischemic CRVO that had final visual field defect grade of marked to severe, compared to only 1 % of eyes with nonischemic CRVO. With V-4e isopter, scotoma was found in 78 % of the eyes with ischemic CRVO compared to only 6 % of the eyes with nonischemic CRVO (p < 0.0001).
Table 24.5
Visual acuity and visual field achieved at 15 months and 2–5 years of follow-up and overall final follow-up in eyes without macular edema
Visual assessment | Nonischemic CRVO | Ischemic CRVO as first diagnosis |
---|---|---|
Visual acuity | ||
15 months a | (n = 93) | (n = 9) |
20/15–20/20 | 43 (46 %) | 1 (11 %) |
20/25–20/30 | 25 (27 %) | 0 (0 %) |
20/40–20/60 | 9 (10 %) | 1 (11 %) |
20/70–20/100 | 5 (5 %) | 0 (0 %) |
20/200–400 | 10 (11 %) | 0 (0 %) |
CF or worse | 1 (1 %) | 7 (78 %) |
2–5 years | (n = 146) | (n = 13) |
20/15–20/20 | 61 (42 %) | 0 (0 %) |
20/25–20/30 | 29 (20 %) | 0 (0 %) |
20/40–20/60 | 20 (14 %) | 0 (0 %) |
20/70–20/100 | 9 (6 %) | 0 (0 %) |
20/200–400 | 25 (17 %) | 5 (38 %) |
CF or worse | 2 (1 %) | 8 (62 %) |
Final b | (n = 316) | (n = 26) |
20/15–20/20 | 138 (44 %) | 0 (0 %) |
20/25–20/30 | 54 (17 %) | 1 (3 %) |
20/40–20/60 | 45 (14 %) | 2 (6 %) |
20/70–20/100 | 26 (8 %) | 1 (3 %) |
20/200–400 | 46 (15 %) | 9 (35 %) |
CF or worse | 7 (2 %) | 13 (50 %) |
Visual field defect | ||
15 months a | (n = 90 eyes) | (n = 9) |
Minimal | 81 (90 %) | 0 (0 %) |
Mild | 8 (9 %) | 2 (22 %) |
Moderate | 0 (0 %) | 3 (33 %) |
Marked | 0 (0 %) | 2 (22 %) |
Severe | 1 (1 %) | 2 (22 %) |
2–5 years | (n = 142 eyes) | (n = 17) |
Minimal | 122 (86 %) | 0 (0 %) |
Mild | 18 (13 %) | 3 (18 %) |
Moderate | 1 (1 %) | 7 (41 %) |
Marked | 1 (1 %) | 4 (23 %) |
Severe | 0 (0 %) | 3 (18 %) |
Final b | (n = 312 eyes) | (n = 28) |
Minimal | 271 (87 %) | 1 (4 %) |
Mild | 24 (8 %) | 4 (14 %) |
Moderate | 13 (4 %) | 9 (32 %) |
Marked | 3 (1 %) | 6 (21 %) |
Severe | 0 (0 %) | 8 (29 %) |
Factors Influencing the Visual Outcome in CRVO
1.
Effect of Foveal Pigmentation and Epiretinal Membrane
Development of foveal pigmentation and epiretinal membrane following chronic macular edema in nonischemic CRVO adversely influences the visual outcome after resolution of macular edema (foveal pigmentation (odds ratio 20.84; p < 0.0001) and epiretinal membrane (odds ratio 9.54; p = 0.0006)); this is an important piece of information. However, in ischemic CRVO, there was no significant association of change in visual acuity with foveal pigmentation and epiretinal membrane (both p > 0.86) because of ischemic damage to begin with, in the macular region in those eyes.
2.
Effect of Neovascular Glaucoma on Visual Outcome in Ischemic CRVO
In my study, neovascular glaucoma developed in 36 % of the ischemic CRVO eyes [206]. As expected, that resulted in further deterioration of visual outcome (visual acuity p = 0.002; visual fields p = 0.008) compared to those without neovascular glaucoma.
3.
Effect of Age
Chen et al. [233], in a study of 59 eyes, found that the factors significantly related to visual outcome were initial visual acuity (p = 0.0001) and age – older patients having a worse visual outcome (p = 0.0029). Glacet-Bernard et al. [240] in 120 CRVO eyes found older age a prognostic factor for poor visual outcome. Similarly, in my study, no patients aged <45 years with initial visual acuity of 20/60 or better had visual acuity deterioration, and among those with initial visual acuity of 20/70 or worse, visual acuity improved in 80 %. In contrast, in those ≥45 years, 15 % of those with initial visual acuity of 20/60 or better experienced deterioration, while only 56 % of those with initial visual acuity of 20/70 or worse showed any improvement. Thus, increasing age was associated with visual acuity deterioration.
The reports of visual outcome in young individuals vary widely from study to study. Priluck et al. [234] in a retrospective study of 42 patients with CRVO who were aged 40 or younger found that their final visual prognosis could not be predicted by the severity of the venous occlusion at the time of diagnosis. Fong et al. [235] in a retrospective study of 103 cases of CRVO in young, nondiabetic adults, followed for at least 6 months, found that 32 % had a final visual acuity of 20/200 or worse and 6 % no light perception. Giuffré et al. [236], in a study of 20 patients with CRVO aged 40 years or less, found that the visual prognosis of young people with CRVO is often poor. In contrast to all those studies, in my study [180], none of those under the age of 45 years, with initial visual acuity of 20/60 or better, had visual acuity deterioration, and in those with initial visual acuity of 20/70 or worse, it improved in 80 %. In those 45 years or older, with initial visual acuity of 20/60 or better, it deteriorated in 15 %, and among those with initial visual acuity of 20/70 or worse, it improved in 56 %. This shows that the visual prognosis for young people is much better than for those 45 years or older.
4.
Effect of Systemic Diseases
In my study [180], among the systemic diseases, only cerebrovascular disease (odds ratio 8.94; p = 0.036) and diabetes mellitus (odds ratio 6.04; p = 0.049) showed a significant association with visual acuity deterioration in eyes with initial visual acuity of 20/60 or better. There was no significant association with arterial hypertension (p = 0.14), ischemic heart disease (p = 0.58), or smoking (p = 0.31). In eyes that presented with initial visual acuity of 20/70 or worse, there was no significant association between visual acuity improvement and arterial hypertension (p = 0.84), cerebrovascular disease (p = 0.82), ischemic heart disease (p = 0.47), diabetes mellitus (p = 0.58), or smoking (p = 0.24).
5.
Effect of Development of Retinociliary Collaterals
There is a prevalent impression among ophthalmologists that development of retinociliary collaterals has a beneficial effect on the course and visual outcome of CRVO, because it improves the circulation in the central retinal vein by bypassing the occlusion – some have claimed visual improvement after radial optic neurotomy, due to development of these collaterals. Priluck et al. [234] in a retrospective study of 42 patents with CRVO, aged 40 or younger, stated that the presence of collaterals closely correlated with a favorable prognosis. However, Giuffré et al. [236, 241], in 94 patients with CRVO, found that the visual acuity of the eyes with CRVO that developed collaterals was not significantly different from the visual acuity of the eyes without collaterals. Quinlan et al. [232], in a retrospective study of 168 eyes with CRVO, also found that the collaterals were not related to any improvement in visual acuity. Garcia-Arumi et al. [210], in their radial optic neurotomy study, found no significant difference in visual acuity between those with and without these collaterals. Thus, the literature gives firm evidence that development of retinociliary collaterals does not make any difference in the visual outcome in CRVO.
In my study [180], 46 % of nonischemic CRVO eyes developed the collaterals with a median time of 15 months. In eyes with ischemic CRVO, 41 % developed retinociliary collaterals with median time of 13 months. My study revealed an interesting phenomenon, not previously described. Nonischemic CRVO (in eyes with initial visual acuity of 20/70 or worse) showed the following:
(i)
The eyes that developed collaterals had poorer initial visual acuity than the eyes that did not (52 % vs. 35 %).
(ii)
Resolution of macular edema took much longer in those with collaterals than in those without (median time 40 months vs. 21 months).
(iii)
After resolution of macular edema, 35 % of the eyes that developed collaterals showed no change in visual acuity, 43 % improved, and 22 % deteriorated. Compared to that, in the eyes without collaterals, this was 6, 76, and 6 %, respectively.
Eyes with ischemic CRVO had similar results.
These findings pose an intriguing question about the retinociliary veins in CRVO: why is the presence of cilioretinal collaterals related to poor initial and final visual acuity? The subject of the site of occlusion in CRVO is discussed above. The closer the site of occlusion to the lamina cribrosa, the fewer tributaries are available in the optic nerve to establish collateral circulation (Fig. 24.1) and the worse the retinopathy. In this case, there is a far greater stress on the few available tributaries in the prelaminar region to develop into collaterals than when the site of occlusion is far back in the optic nerve. Therefore, eyes with retinociliary collaterals on the optic disc have a much greater chance of having a severe type of retinopathy, with much worse visual acuity and more marked macular edema than do those without collaterals, as shown by my study. The severity of retinopathy in turn determines the visual acuity outcome. Therefore, not surprisingly, the higher the prevalence of retinociliary collaterals on the optic disc, the smaller is the chance of visual improvement and the greater the chance of visual deterioration – where there are no retinociliary collaterals, there are plenty of venous tributaries available within the optic nerve and no need to develop collaterals on the disc.
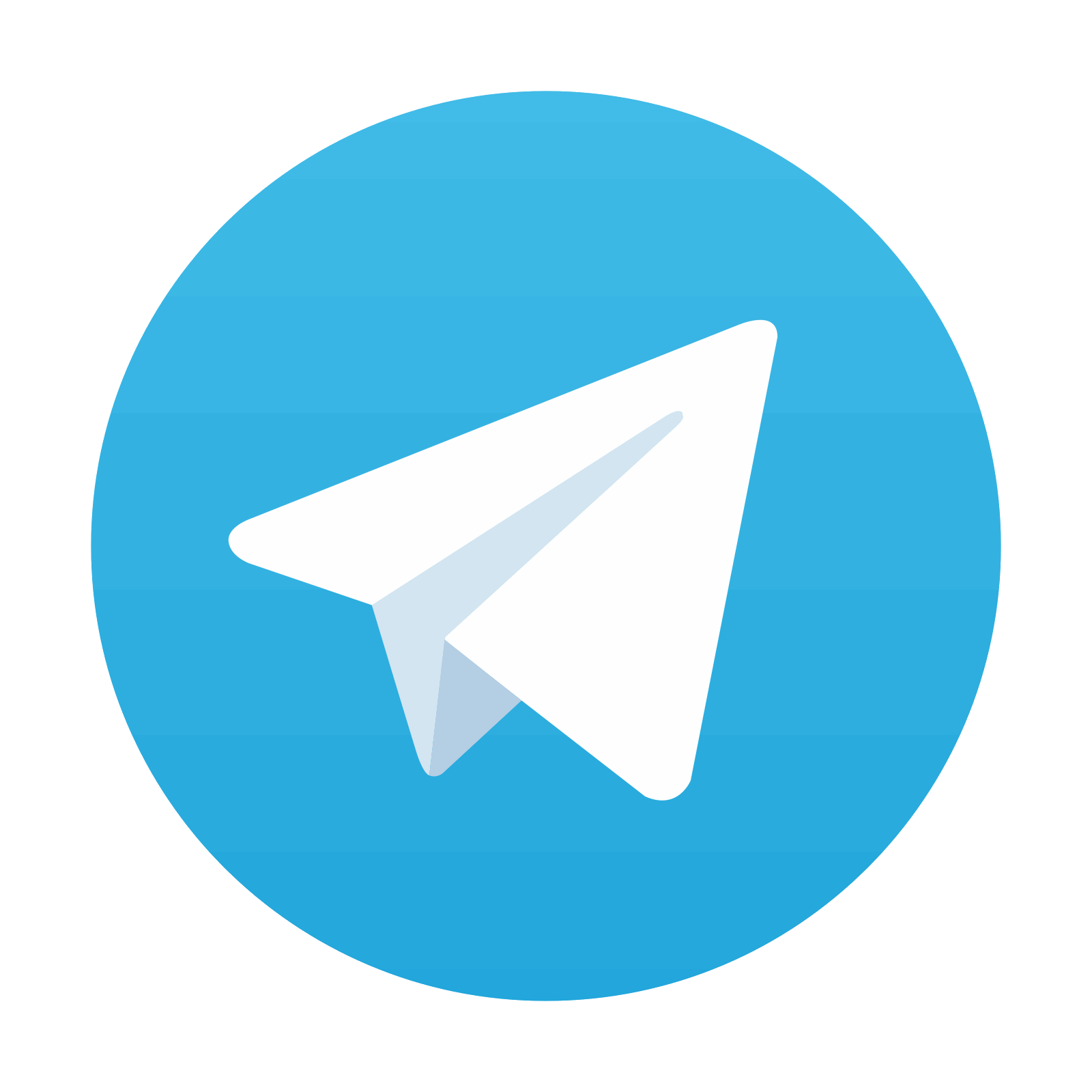
Stay updated, free articles. Join our Telegram channel

Full access? Get Clinical Tree
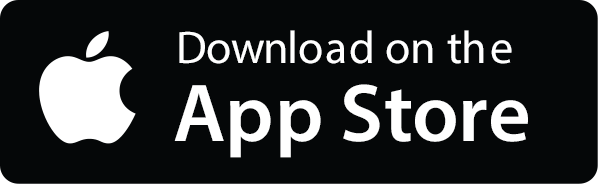
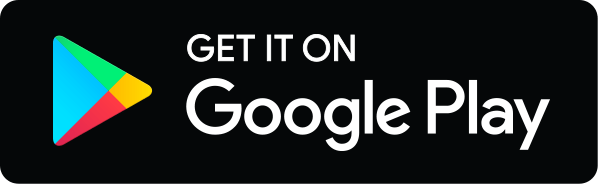
