Cataracts and Systemic Disease
ANNA K. JUNK MD
DONALD A. MORRIS MD
Cataracts are frequently found in association with other types of ocular pathology and may or may not be associated with systemic disease. Lens pathology may evolve secondary to a genetic defect and reveal carrier status, as in Lowe’s syndrome. A distinct appearance of the lens may allow a diagnosis of concurrent systemic disease. Exogenous agents, pharmaceuticals, toxins, radiation, infections, and metabolic disorders can cause cataracts and systemic disease. For this review we conducted literature searches using PubMed.
OCULOCEREBRORENAL SYNDROME OF LOWE (OCRL)
In 1952 Lowe and co-authors1 were the first to recognize congenital cataracts, infantile glaucoma, developmental and mental retardation, and aminoaciduria as a clinical entity. OCRL has been mapped to Xq24–q26 on the X-chromosome.2 Mutations in the OCRL1 gene result in the Lowe’s syndrome phenotype. The gene encodes for a 105 kD protease, phosphatidylinositol (4,5) bisphosphate (PtdIns [4,5]P2) 5-phosphatase (PIP2 5-phosphatase). PIP2 5-phosphatase, which is located in the Golgi apparatus, is defective in one to 10 males per million in the United States. Different mutations in OCRL1 may lead to less severe phenotypes with respect to mental retardation and renal dysfunction. OCRL can be confirmed by DNA diagnosis and skin fibroblast analysis for PIP2 5-phosphatase.3
The dense bilateral lens opacities may present as a biconvex-shaped cataract or as posterior, polar, nuclear, or total cataract. The discoid lens shape may result from loss of lens material due to a posterior lens capsule defect4 or defective lens fiber formation and subsequent degeneration.5 The cataracts are present at birth, and glaucoma may be present congenitally or develop within the first 3 years of life. Other ocular findings may include corneal opacity, mitotic pupil, enophthalmos, and hypotonia.6 The cognitive impairment presents with a discrete behavioral phenotype that includes temper tantrums, irritability, complex repetitive behaviors, and unusual mannerisms. Severe renal Fanconi’s syndrome may lead to progressive renal impairment. Most boys will develop a distinctive facies and habitus, and attain a height of less than 5 feet because of developmental retardation. Female carriers manifest characteristic but usually asymptomatic lenticular opacities that will correctly identify carrier status with 100% sensitivity in postpubertal females. These opacities are typically small, irregularly shaped, off-white or gray in color, nonrefractile in appearance, and distributed around the lens equator, more anteriorly than posteriorly (Figs. 1 and 2). Most importantly, and distinctively, these opacities are clustered in radial bands or wedges in the peripheral cortex of the lens and are visible by retroillumination. Typically, the opacities are moderately dense for one or two clock-hours, are then less numerous or even absent for another clock-hour or two, and so on. These opacities must be differentiated from the polychromatic, iridescent “crystals” found in Steinert’s myotonic dystrophy (DM), gray-white random opacities in carriers of X-linked adrenoleukodystrophy, sutural opacities in Nance-Horan’s (NH) syndrome, snowflake granules beneath the anterior and posterior capsule in diabetes mellitus, highly uniform white dots of hypoparathyroidism, and equatorial opacities in cataracta coronaria or ceruleana. Some females also manifest a dense white, central, posterior cortical cataract in the precapsular area. Although the posterior central cataract is apparently congenital, the equatorial and anterior cortical punctate opacities are uncommon in prepubertal female Lowe’s syndrome carriers.7 Carrier status may also be confirmed by DNA diagnosis.
NEUROFIBROMATOSIS 2 (NF2)
Neurofibromatosis 2 (NF2) is an autosomal-dominant (AD) disorder caused by mutations that inactivate the NF2 tumor suppressor gene. Multiple central and peripheral nervous system tumors and ocular abnormalities are common in NF2, and bilateral vestibular schwannomas (acusticus neurinoma) are pathognomonic for the disease. Constitutional nonsense or frameshift NF2 mutations are associated with severe NF2 (i.e., earlier onset of symptoms and more tumors), splice site mutations with variable disease severity, and missense mutations with mild disease. Cataracts are the most common nontumor and ocular manifestation in NF2, and are prevalent in about 60% to 80% of NF2 patients. In animal models, lens fiber cells that are more differentiated express less NF2 protein than the epithelial regions of the lens, which suggests that the NF2 protein may play a role in lens epithelial cell migration or elongation. In one study,8 the overall prevalence of cataracts in NF2 was 33%, but was significantly lower in patients with somatic mosaics and in individuals with new, as yet unknown mutations, and onset of symptoms above the age of 20 years than in patients with classic NF2, nonsense, or frameshift mutations. Of the NF2 patients with cataracts in that study, 29% were diagnosed with cataracts at an age below 10 years, and 47% were diagnosed under the age of 20 years. In 70% of the patients cataract diagnosis preceded nonocular signs or symptoms of NF2. Cataracts typically present as posterior subcapsular plaque-like opacities (Figs. 3 and 4). Other ocular manifestations include retinal hamartomas, optic nerve sheath tumors, fibrotic maculopathies, and perineural calcification of the optic nerve.9
![]() Fig. 3. NF2. Paracentral plaque-like retrolental opacity in slit-lamp view. (Courtesy of Dr. F.D. Ellis, Zionsville, Indiana.) |
X-LINKED CATARACT (NANCE-HORAN’S (NH) SYNDROME)
Three types of X-linked cataracts have been reported: congenital total, which presents with posterior sutural opacities in heterozygotes; congenital, with microcornea or slight microphthalmia; and the congenital cataract-dental syndrome or NH syndrome.10 Current linkage analysis is suggestive of allelic heterogeneity in all three types of X-linked cataract. The NH syndrome locus is located within or close to the Xp22.1–Xp22.2 region.11,12 Sutural cataracts have been found in female carriers of NH syndrome, and affected males present with total congenital cataract, microcornea, dental abnormalities, and dysmorphic features. One-third of affected individuals have mental retardation.13 The gene for X-linked isolated congenital cataract has been mapped to Xp22, and thus lies within the NH locus. Two-thirds of affected patients in one series had complex congenital heart disease.14
LEBER’S CONGENITAL AMAUROSIS (LCA)
LCA, which occurs in three of 100,000 newborns worldwide, presents with severe vision loss at or near birth. Associated ocular features may include eye poking (also known as the oculodigital sign of Franceschetti), ptosis, strabismus, high hyperopia, high myopia, cataracts,15 keratoconus/keratoglobus, microphthalmos, macular coloboma, pigmentary retinopathy and maculopathy, disc edema, and retinal vascular attenuation. Of great interest are the occasional patients with LCA who have a completely normal retinal appearance. A severely reduced or nondetectable electroretinogram (ERG) obtained early in the disease process is an absolute diagnostic prerequisite. Autosomal-recessive (AR) inheritance is commonly found. LCS is variably associated with mental retardation. Six genes, which participate in a wide variety of retinal pathways, have been shown to be mutated in LCA: retinoid metabolism (RPE65), phototransduction (GUCY2D), photoreceptor outer segment development (CRX), disk morphogenesis (RPGRIP1), zonula adherens formation (CRB1), and cell-cycle progression (AIPL1).16 Longitudinal studies of visual performance show that most LCA patients remain stable, some deteriorate, and rare patients exhibit improvements. Histopathological analyses reveal that most patients undergo extensive degenerative retinal changes. Some have an entirely normal retinal architecture, whereas others have primitive, poorly developed retinas. Gene therapy for RPE65-deficient dogs was shown to partially restore sight, and provides the first real hope of treating this devastating blinding condition.17
WILSON’S DISEASE
Wilson’s disease is an AR disorder of copper metabolism that manifests in the first or second decade of life with a prevalence of 1:30,000. The abnormal gene (ATP7B) is located on chromosome 13q and encodes a copper-transporting ATPase with two functions: transport of copper into the plasma protein ceruloplasmin, and elimination of copper through the bile.18 The hepatocyte’s Golgi apparatus fails to incorporate copper into ceruloplasmin, leading to toxic copper concentrations in the central nervous system, liver, kidney, and eye. Forty percent of patients may present initially with ocular findings. If ocular signs are present, Kayser-Fleischer’s corneal ring is most common, and is found in up to 95% of patients with Wilson’s disease and eye manifestations. Kayser-Fleischer’s ring is a golden-brown line (1–3 mm wide) located in the deep corneal periphery adjacent to the limbus. Its color has been described as orange-brown, brown-green, golden-yellow, blue, ruby-red, or a mixture of these colors. Visible copper sulfur deposits in the inner third of Descemet’s membrane typically begin superiorly and then proceed inferiorly until they complete Kayser-Fleischer’s ring, leaving an oval of central clear cornea inside.
Sunflower cataract is named after the patel-shaped alignment of tiny anterior subcapsular copper deposits. It is a rare manifestation of Wilson’s disease (Fig. 5) and is present in 20% to 30% of patients with ocular manifestations. The copper-red to blue-green deposits form a disk-shaped central opacity in the anterior lens capsule, which is usually denser in the pupillary opening, with spoke-like radiations toward the periphery. Posterior lens capsule deposits appear in a fern-like pattern.19 The lens opacities may reverse on a low copper diet and treatment with systemic D-penicillamine.20
Extrapyramidal-motor disturbances of Wilson’s disease include akinetic rigidity and choreatic, athetotic, and dystonic hyperkinesias. Facial hypomimic, dystonic, and myoclonic symptoms lead to an “indifferent expression.” In late stages the patient may be paralyzed, while sensory functions remain preserved. Cerebellar symptoms include nystagmus, blepharospasm, dysarthria, resting, and intentional flapping tremor. Sixty percent of patients develop psychiatric symptoms. They appear irritable and aggressive, and display diminished intellectual performance, mood changes, depression, and paranoid-hallucinatory syndromes. Hepatosplenomegaly, acute or chronic hepatitis, and cirrhosis complicated by icterus, splenomegaly, esophageal varices, and ascites may develop. Hemolytic anemia, renal osteopathy, and secondary osteomalacia are late manifestations, as is a dark-brown to green discoloration of the skin. Other systemic diseases associated with hypercupremia may lead to similar eye findings.
HEREDITARY HYPERFERRITINEMIA-CATARACT SYNDROME (HHCS)
HHCS is an AD-inherited cataract associated with elevated serum ferritin levels but otherwise normal iron levels and hematologic parameters. It was first described in 1995.21,22 Linkage studies have mapped HHCS to chromosome 19q13, the light chain of ferritin (FTL),23 and the major intrinsic protein of lens fiber membrane (MP19).24 The minimum prevalence of HHCS is estimated at 1:200,000.25 To date, 11 different point mutations and four small deletions in a highly conserved sequence of the iron-responsive element (IRE) in the FTL gene have been identified in HHCS families. These mutations lead to the characteristic high expression levels (10-fold above normal serum levels) of poorly regulated L-ferritin.26 Overexpression and accumulation of L-ferritin in lens fibers results in a highly distinctive, progressive cataract that varies in age of onset and severity.27 Though congenital and infantile cataracts have been reported, HHCS is most commonly initially diagnosed in childhood and adolescence when patients present with symptoms of glare and severe photophobia that exceeds visual loss. The opacities consist of multiple peripheral, white, dust-like (pulverulent) dots and larger anterior cortical axial flecks, small crystalline aggregates, and translucent vacuoles that are best visualized by retroillumination. In early stages (i.e., in neonates and infants) the axial anterior cortex and far periphery are involved first. With progression the midperiphery of the lens will develop small punctate opacities while axial cortical aggregates tend to coalesce into radially oriented spokes. A few opacities may appear in the nucleus and posterior cortex of the lens. Regular polyhedral, crystalline, diffractive deposits composed of L-ferritin crystals are seen under light microscopy.28 No pathologic consequences of ferritin accumulation in other tissues have been reported to date, and no systemic treatment is required.
PHENYLKETONURIA (PKU)
The association of PKU with cataract is controversial.29 PKU is an AR disorder caused by mutations in the gene encoding for phenylalanine hydroxylase, prevalent in 1:4,000 to 1:40,000 newborns. Prenatal and postnatal diagnosis is available and early dietary restriction of phenylalanine in infants and children will avoid severe complications like mental retardation. A study of 46 untreated patients with PKU did not provide evidence of a positive correlation with cataracts. Another series detected cataracts in six of 11 middle-aged patients with untreated PKU suggesting that the lens opacities may be due to self inflicted trauma or treatment with high dose of thioridazine hydrochloride. Additional findings included phthisis and choroidal hypopigmentation.30 Bilateral lamellar cataracts were reported in one case of PKU.31
MYOTONIC DYSTROPHY (DM)
The most common type of muscular dystrophy in adults, DM, is an AD disorder based on noncoding microsatellite expansion. As in other disorders caused by microsatellite expansion, there is evidence of anticipation (i.e., with each generation the disease severity increases and age of onset decreases) and a tendency toward maternal transmission of a severe congenital form of the disease. An unstable repeat sequence of triplet nucleotides (CTG) on chromosome 19q13.3 has been identified as the cause in the majority of families (DM1). It results in CTG expansion at the 3′ untranslated portion of the Dystrophia myotonica-protein kinase gene (DMPK). DM2 has been mapped to chromosome 3q21, where it causes an untranslated CCTG expansion (mean = ∼5,000 repeats) located in intron 1 of the zinc finger protein 9 (ZNF9) gene.32 Both mutations will result in the typical phenotype of DM in adults, which is characterized by hyperexcitability of skeletal muscle (myotonia) and muscle degeneration, a conduction defect in cardiac muscle cells, endocrine disorders, and a typical iridescent cataract. The accumulation of these repeat motif expansion RNAs appears to express a preferential affinity to some RNA-binding proteins at the expansion, and thus causes a global disruption in RNA splicing and cell metabolism. A third mutation for DM has been postulated but has not yet been identified.33
In general, patients with DM2 show less anticipation and a milder phenotype, and lack a congenital form. Affected individuals (DM1 and DM2) present with myotony and a characteristic pattern of weakness involving the facial, neck flexor, finger flexor, and hip girdle muscles. Severe arrhythmias or progressive cardiomyopathy may be fatal. Hypotestosteronism and oligospermia in males, and insulin insensitivity are frequent endocrinologic features. A specific set of serological changes is present, which includes low γ-globulin, elevated creatine kinase, and elevated follicle stimulating hormone (FSH) in males. In young adults, dust- and flake-like iridescent and highly refractile multicolored “needles” crisscross the anterior and posterior cortexes of the lens (the so-called “christmas-tree cataract”). The colors vary according to the angle of the incident light, and only a dim outline of the cataract is seen under retroillumination (Figs. 6 and 7). Under the scanning electron microscope the needles are smooth, rectangular, plate-like elements that are bordered by membranes and amorphous material, and run crisscross through the lens. The needles have a high sulfur content and pronounced S-S, CS-SC, and C-S vibrations under energy-dispersive x-ray and Raman microanalysis.34 They increase and accumulate with age, and may eventually result in a mature cataract. Rarely, macular and tapeto-retinal pigment epithelial degeneration, and optic atrophy are associated with DM.
![]() Fig. 6. Christmas-tree cataract. Multiple small, irregularly sized opacities in the superior and posterior superficial cortex in a 43-year-old male patient with DM (Scheimpflug image). |
![]() Fig. 7. Christmas-tree cataract. Dust- and flake-like iridescent and highly refractile multicolored “needles” crisscross the anterior lens cortex in a patient with DM. |
Familial mitochondrial myopathy should be differentiated from DM. Patients present with variable facial weakness, ranging from mild forms to severe progressive ophthalmoplegia associated with facial, pharyngeal, and limb muscle involvement and premature bilateral cataract that requires removal in infancy, adolescence, or early adulthood. Biopsies of skeletal muscles indicate myopathy, and histochemistry and electron microscopy reveal abnormal mitochondria in type I fibers. The syndrome is associated with the HLA haplotype (A2–B21).35
LYSOSOMAL STORAGE DISORDERS
Most lysosomal storage diseases are associated with deficiencies of glycosidases that are involved in the catabolism of the sugar chains of glycolipids, oligosaccharides, and glycoproteins. They include single-enzyme deficiencies, the most frequent being Gaucher’s disease (caused by deficient acid β-glucosidase), Fabry’s disease (enzyme defect: α-galactosidase A), Tay-Sachs’ disease (β-hexosaminidase A deficiency), and functional deficiencies of multiple enzymes (reviewed in Ref. 36). Less common lysosomal disorders include mucopolysaccharidosis (MPS) I (Hurler/Scheie’s disease, enzyme defect: α-iduronidase), MPS type IVA (Morquio type A; enzyme defect: N-acetylgalactosamine-6-sulfate-sulfatase), GM1-gangliosidosis/MPS IVB (Morquio type B; enzyme defect: β-galactosidase), mucolipidosis II/III (enzyme defect: phosphotransferase), MPS VI (Maroteaux-Lamy’s syndrome, enzyme defect: arylsulfatase B), and α-mannosidosis. Extensive clinical heterogeneity is seen in lysosomal storage disorders with regard to the age of onset and severity of symptoms, the organs involved, and central nervous system effects. Clinically related AR disorders include mucolipidosis, sialidosis, and galactosialidosis (the latter two are both due to deficiency of lysosomal sialidase).37 While corneal opacities are observed frequently in MPS, cataracts have been reported in Hurler’s syndrome, Morquio’s syndrome (types A and B), Fabry’s disease, and α-mannosidosis. The lysosomal storage disorders associated with cataracts are detailed below.
MUCOPOLYSACCHARIDOSIS (MPS) I (HURLER’S SYNDROME)
MPS type I (MPS I) is an AR disorder caused by a deficiency in lysosomal hydrolase, α-L-iduronidase (IDUA). It results in a failure to degrade the glycosaminoglycans dermatan sulfate and heparan sulfate. MPS I presents within a spectrum of clinical phenotypes, in which Hurler’s and Scheie’s syndromes represent the two extremes. The gene encoding IDUA is located on the short arm of chromosome 4, and over 50 mutations have been identified in affected patients. Mutation analysis may aid in the prognosis, but in most cases the distinction between various subtypes is made on clinical grounds. Patients who are diagnosed at a young age (<18 months) will usually turn out to have a Hurler phenotype, whereas children and adults with Scheie’s syndrome (which involves a milder clinical course and normal life expectancy) are often diagnosed at a later age (>5 years). Intracellular degradation of heparan sulfate and dermatan sulfate are impaired and result in excessive urinary excretion and excess storage. Fibroblasts of MPS I patients with different clinical phenotypes differ in their kinetic parameters of α-iduronidase and glycosaminoglycan accumulation.38 The clinical features of Hurler’s syndrome (the rapidly progressive, severe form of MPS I) include dwarfism, coarse facies, synophrys, saddle nose, hypertrichosis, mental retardation, and deafness, which are associated with death in the first decade. Diffuse corneal clouding, which is denser in the limbal periphery than in the corneal center, is the most common ocular finding, but a retinitis pigmentosa (RP)-like picture has also been reported and may be associated with posterior subcapsular cataract. Bone marrow transplantation (BMT) has become a treatment of choice for some patients with Hurler’s syndrome, and has the potential to alter the natural history of the disease.39 The response to this therapy in different organs is variable. The brain can be protected as long as BMT is performed before the onset of irreversible pathology (18 months to 2 years of age). Hepatosplenomegaly resolves readily, and airway and cardiac muscle functions improve. Cartilage and bone do not respond well to BMT and therefore patients may require corrective surgery. Cardiac valves will deteriorate with time, corneal clouding never clears completely, and posterior subcapsular cataracts will progress as a consequence of BMT.40 Enzyme replacement therapy (ERT) for MPS I has been tested in phase I/II and III clinical trials, and was demonstrated to be effective in patients with attenuated forms of the disease (intermediate/Scheie phenotype).41 The lack of apparent central nervous system penetration by enzymes currently limits its use in patients with the Hurler phenotype.
MUCOPOLYSACCHARIDOSIS (MPS) IV (MORQUIO’S SYNDROME)
Morquio’s syndrome is a rare AR MPS with a prevalence of 1:76,000. It is characterized by a reduced activity of N-acetylgalactosamine-6-sulfate-sulfatase (type A), or β-galactosidase (type B). The deficiency of β-galactosidase is also responsible for GM1-gangliosisdosis, the severe form of which will lead to fetal hydrops. Fibroblast cultures of a skin biopsy can be used to differentiate between Morquio types A and B since they show reduced activity of N-acetyl-galactosamine-6-sulfate-sulfatase or β-galactosidase, respectively.42 Clinically, both enzyme defects lead to a lysosomal storage disease with accumulation of keratan sulfate and chondroitin-6-sulfate in connective tissue, the skeletal system, and the teeth. The central nervous system is not involved. Consequently, abnormalities of the skeletal system (dwarfism), aortic valve disease, and dental abnormalities occur. Ophthalmologically, uniformly distributed diffuse corneal opacification is recognized in early childhood. Only in adulthood do the corneal changes become clinically significant. Alterations of the trabecular meshwork may occur and lead to glaucoma.43 Lens opacities have been reported.44 Rarely, Morquio’s syndrome may be associated with optic atrophy and tapeto-retinal degeneration.45
FABRY’S DISEASE
Fabry’s disease (angiokeratoma corporis diffusum) results from any one of multiple mutations in the X-linked α-galactosidase A gene, with a prevalence of 1:40,000 and an average age at diagnosis of 25 to 30 years. The mutations are kindred-specific, often spontaneous, and result in varying degrees of functional enzyme deficiency, leading to glycosphingolipid deposits, especially in vascular and reticuloendothelial tissue. Symptoms of anhydrosis, acroparesthesias, rash, and renal disease are suggestive of Fabry’s disease. A recent series of 67 patients46 reported acroparesthesia, which was described as burning or painful “pins and needles” in 100% of males (homozygotes), or numbness and increased sensitivity in 53% of females (heterozygotes), as the most constant clinical feature. The total or partial inability to sweat, which often culminates in “overheating” and precipitation of acroparesthesia, is present in 93% of male patients versus 11% of female patients. Gastrointestinal symptoms (most commonly chronic diarrhea) is present in 90% of affected male patients compared to 11% of females. Cardiovascular symptoms are common and include ischemic heart disease, intermittent claudication, paroxysmal palpitations, atrial fibrillation, and episodic dyspnea. Renal involvement (most commonly present as proteinuria) may require renal transplantation. Ninety-three percent of males and 13% of females develop a characteristic angiokeratomatous rash on the lower trunk and upper thighs. Males often exhibit a waxy, pallid-sallow complexion, thick eyelids, and a subtle coarsening of facial features. In one study,46 triangular anterior subcapsular cataract was present in 48% of males and 14% of females. Posterior subcapsular cataract was detected in 9% of homozygous patients, but in none of the heterozygous individuals. Conjunctival vascular tortuosity and ampulliform vessel dilatations were the most common ocular sign of Fabry’s disease, being present in 100% of males and 83% of females, followed by cornea verticillata in 96% and 76% of males and females, respectively, and retinal vascular tortuosity in 88% and 21% of males and females, respectively.
Another series of 32 homozygous male patients with Fabry’s disease (mean age = 37 years) reported a best-corrected visual acuity of 20/20 in 75% of the patients.47 In this series cornea verticillata was diagnosed in 44% of the eyes, and a corneal haze was found in 84%. Corneal haze was considered to be a sign of disease progression. Twelve patients had bilateral posterior “Fabry’s” cataracts (feathery opacities that radiate subcapsularly from the posterior pole along the posterior suture lines). In five patients, bilateral anterior subcapsular opacities were present as well. Thirty-seven percent of the patients had an enlarged blind spot in the perimetry, which presumably was related to subclinical optic neuropathy.
SIALIDOSIS TYPE 1 (MUCOLIPIDOSIS I)
Lysosomal sialidase (EC 3.2.1.18) has a dual physiological function: it participates in intralysosomal catabolism of sialylated glycoconjugates, and is involved in the cellular immune response. Mutations in the sialidase gene NEU1 (located on chromosome 6p21.3) result in a AR disorder, sialidosis, which is characterized by the progressive lysosomal storage of sialylated glycopeptides and oligosaccharides. Sialidosis type I is a milder, late-onset, normosomatic form of the disorder. Type I patients develop visual defects, myoclonus syndrome, cherry-red macular spots, ataxia, hyperreflexia, and seizures. The severe early-onset form, sialidosis type II, is also associated with dysostosis multiplex, a Hurler-like phenotype, mental retardation, and hepatosplenomegaly.37 Patients typically present in the second decade of life with dysarthria, limb ataxia, and intention myoclonus. Usually an eye examination reveals cherry-red spots at the macula. Two cases with additional perinuclear (deep cortical) cataracts or punctate subcapsular cataracts have been reported.48
MANNOSIDOSIS
α-Mannosidosis is an AR lysosomal storage disease caused by a deficiency of lysosomal α-D-mannosidase. Lysosomal α-D-mannosidase is involved in the catabolism of glycoproteins through the sequential degradation of mannose and complex oligosaccharides.49 Clinically, patients present with hepatosplenomegaly, mental retardation, and mild dysostosis, and must be differentiated from patients with Hurler-Scheie’s syndrome. Rarely, patients may present with scattered punctate opacities in the entire lens50 or posterior spoke-like cataracts.51 A conjunctival biopsy may confirm the diagnosis if fibroblasts and endothelial cells contain membrane-bound vacuoles and vesicles comprised of homogeneous osmiophilic globules.
SYNDROMES ASSOCIATED WITH ALTERED LENS GEOMETRY
ALPORT’S SYNDROME (AS)
AS is a genetically heterogenous disorder arising from mutations in the genes that code for basement membrane type IV collagen. Clinically, the syndrome is characterized by progressive nephropathy, sensorineural hearing loss, and ocular abnormalities, including anterior lenticonus and posterior subcapsular cataracts. AS is associated with X-linked (Xq22, 80% to 85% of cases), AR (approximately 15% of cases), and rarely AD (chromosome 2q) inheritance. Autosomal forms have been attributed to defects in the COL4A3 or COL4A4 gene. The X-linked mutations have been mapped to defects in the α-5-chain of type IV collagen (COL4A5) gene, which compromise both COL4A3 and COL4A4.52 A subtype of X-linked Alport’s syndrome (XLAS), in which diffuse leiomyomatosis is an associated feature, reflects deletion mutations involving the adjacent COL4A5 and COL4A6 genes. All reported mutations lead to abnormalities in the basement membranes of the glomerulus, cochlea, retina, lens capsule, and cornea, which eventually constitute the typical phenotype.
Anterior lenticonus (Figs. 8 and 9) is considered an integral part of AS. The anterior capsule thins and allows the lens to bulge into the anterior chamber, using the pupil as a mold.53 Electron microscopy of the renal glomeruli and anterior lens capsule can confirm capsular thinning and dehiscences.54,55 Anterior lenticonus is typically diagnosed in the second and third decades of life, when it causes clinically significant decreased vision,56 but it may be present in adolescence and result in spontaneous rupture of the anterior lens capsule.57 Posterior subcapsular cataract occurs quite frequently; however, many patients receive glucocorticosteroids for their renal condition, which may play an etiologic role in these cataracts. Additional ocular features described in XLAS include other corneal dystrophies, microcornea, corneal arcus, iris atrophy, posterior lenticonus, spontaneous lens rupture, spherophakia, a poor macular reflex, fluorescein angiogram hyperfluorescence, electrooculogram and ERG abnormalities, and retinal pigmentation abnormalities.58
FECHTNER’S SYNDROME
Fechtner’s syndrome is an AD macrothrombocytopenia that results from a mutation on chromosome 22 in the MYH9 gene. It encodes for the nonmuscle myosin heavy chain IIA (NMMHC-A),59 which is expressed in the platelets, kidney, leukocytes, and cochlea. Fechtner’s syndrome, which is also reported as a variant of AS, shares with the latter the triad of nephritis, sensorineural hearing loss, and eye abnormalities, and presents the additional features of macrothrombocytopenia and polymorphonuclear inclusions (called Döhle-like bodies). May-Hegglin’s anomaly, Sebastian’s syndrome, and Epstein’s syndrome are macrothrombocytopenias caused by distinct mutations of MYH9; however, unlike Fechtner’s syndrome, they are not associated with cataract. They are also distinguished from Fechtner’s syndrome by different combinations of clinical and laboratory signs, although considerable overlap exists. A recent report60 suggested that May-Hegglin’s anomaly, Sebastian’s syndrome, Epstein’s syndrome, and Fechtner’s syndrome are not distinct entities, but rather form a single disorder with a continuous clinical spectrum that varies from mild macrothrombocytopenia with leukocyte inclusions to a severe form complicated by hearing loss, cataracts, and renal failure. The term “MHY9-related disease” was proposed in that study.
POSTERIOR LENTICONUS
Posterior lenticonus (or lentiglobus) is a unilateral or bilateral condition caused by asymmetrical thinning and posterior bowing of the posterior lens capsule centrally or peripherally. This has variable effects on the adjacent lens cortex: opacification sometimes occurs, or it may manifest as a high degree of astigmatism that can be irregular but without cataract. More severe cases are associated with a progressive opacity of the lens lamellae in the abnormal area, sometimes with a dense discoid opacity of the posterior pole. Although sporadic cases may occur, many are inherited as an X-linked or AD trait. It is therefore important to perform a slit-lamp examination of a patient’s relatives. Posterior lenticonus has been associated with microcornea, hyperglycinuria, Duane’s syndrome, and anterior lentiplanus.13,61
CATARACT ASSOCIATED WITH MUTATIONS IN CHOLESTEROL METABOLISM ENZYMES
Inherited defects in enzymes of cholesterol metabolism are associated with cataracts. This association results from the lens’ ongoing requirement for on-site synthesis of cholesterol. Impaired synthesis can lead to alteration of the lens membrane structure. The lens cell membrane contains the highest cholesterol content of any known membrane. Smith-Lemli-Opitz’s syndrome (SLOS), cerebrotendinous xanthomatosis (CTX), mevalonic aciduria, and Conradi-Hunermann-Happle’s (CHH) syndrome all involve mutations in cholesterol metabolism enzymes, and affected patients can develop cataracts.62
SMITH-LEMLI-OPITZ’S SYNDROME (SLOS)
SLOS (or RSH, after the initials of the last names of three patients) is an AR disorder of cholesterol biosynthesis. It involves a broad spectrum of phenotypic abnormalities caused by mutations of the 7-dehydrocholesterol reductase gene (DHCR7) on chromosome 11, and is diagnosed in 1:15,000–20,000 live-born infants.63 A prenatal diagnosis can be made by detection of elevated 7-dehydrocholesterol or of SLOS-causing mutations in the DHCR7 gene.64 The clinical phenotype of SLOS includes a lethal form called “idiopathic” hydrops. Neurological findings include microcephaly with cerebral dysgenesis and demyelinization, agenesis of the corpus callosum, and cerebellar vermis dysgenesis. Cleft palate, different forms of congenital heart defects, pyloric stenosis and/or Hirschsprung dysganglionosis, adrenal (cortical) insufficiency, cholestatic liver disease, limb malformations, and genital ambiguity in genetic males are also found. Congenital cataracts are present in 20% of affected patients. Other ophthalmologic signs observed in SLOS include blepharoptosis, epicanthal folds, strabismus, downslanting small palpebral fissures, mild exophthalmos, retinal pigment epithelial defects, pale disks, nystagmus, microcornea, aniridia, postlenticular membrane, Duane’s retraction syndrome, absence of lacrimal puncta, opsoclonus-like movements, corneal enlargement, abnormal iris insertion, bilateral optic pits, sclerocornea, and optic nerve demyelination.65
CEREBROTENDINOUS XANTHOMATOSIS (CTX, ESSENTIAL HYPERCHOLESTEROLEMIC XANTHOMATOSIS)
CTX is an AR neurometabolic disease caused by a deficiency of the mitochondrial sterol 27-hydroxylase. The metabolic defect causes reduced bile acid synthesis and increased plasma and tissue cholestanol levels, which left untreated will lead to the classic phenotype of juvenile cataracts, neurological dysfunction, and tendon xanthomas. It is crucial to diagnose CTX before neurological deterioration occurs to prevent brain damage that can lead to severe mental and neurological pathologies, and death.66 Children with unexplained bilateral cataracts and chronic diarrhea should be worked up to rule out CTX. Treatment with chenodeoxycholic acid will normalize laboratory parameters, stop the diarrhea, and prevent progression of the disease. After a year of treatment the motor development delay disappears, the intelligence quotient improves, and EEG abnormalities disappear.67 In the natural course of the disease, cataracts become evident between the first and third decades, and are characterized by small irregular corticonuclear opacities, anterior polar cataracts, and dense posterior subcapsular cataracts.68 Histologically they contain numerous membranous structures with lipid-filled vacuoles. Bilateral optic atrophy and premature retinal senescence (drusen and retinal pigment epithelial defects) have been reported. The vitreous may contain suspended yellowish flakes resembling cholesterol crystals.69 Palpebral xanthelasmas, corneal lipoid arcus, and proptosis have been reported. Other systemic manifestations include mental retardation, pyramidal and cerebellar signs, brain atrophy, atherosclerosis and myocardial infarction, pulmonary insufficiency, and osteoporosis.
CONRADI-HUNERMANN-HAPPLE’S (CHH) SYNDROME (X-CHROMOSOMAL DOMINANT CHONDRODYSPLASIA PUNCTATA TYPE II)
CHH syndrome (X-chromosomal dominant chondrodysplasia punctata type II) is an X-linked dominant disorder that is characterized by ichthyosis, chondrodysplasia punctata, cataracts, and short stature. The disease occurs almost exclusively in females. It is presumed to be lethal in males; however, only a few affected males have been reported. CHH shows increased disease expression in successive generations (i.e., anticipation). Cholesterol biosynthesis is deficient due to a 3-β-hydroxysteroid-δ8,δ7-isomerase deficiency based on mutations in the emopamil binding protein (EBP) gene.70,71
MEVALONIC ACIDURIA
Mevalonic aciduria is caused by a genetic deficiency of mevalonate kinase, and is characterized by very high mevalonic acid plasma levels, developmental malformations, and cataracts. Chronic exposure of the lens to mevalonic acid can induce cataracts due to a toxic effect on the lens cell membranes, resulting in an apparent progressive increase in membrane permeability.72
DISORDERS ASSOCIATED WITH IMPAIRED DNA REPAIR MECHANISMS
Deoxyribonucleic acid (DNA) repair is a fundamental process in maintaining the integrity of genomic DNA that is continuously challenged by intrinsic or environmentally induced alterations. Consequently, failure of DNA repair may affect normal growth and development, aging, programmed cell death (apoptosis), and uncontrolled cell proliferation (cancer). The structure and integrity of DNA may be altered spontaneously because of intrinsic instability of chemical bonds in DNA, or endogenously during cell metabolism. External factors such as ionizing radiation, ultraviolet (UV) light, or chemicals predispose affected individuals to skin and eye pathologies. The following AR-inherited disorders can be distinguished clinically and in DNA repair assays: xeroderma pigmentosum (XP), Cockayne’s syndrome, trichothiodystrophy (TTD), Bloom’s syndrome, Rothmund-Thomson’s syndrome, and Werner’s syndrome.73 Of these, TTD and Cockayne’s, Rothmund-Thomson’s, and Werner’s syndromes are associated with cataract formation, and are discussed in detail below.
COCKAYNE’S SYNDROME
Cockayne’s syndrome is a progressive neurological disorder that is characterized in infancy by growth failure (“cachectic dwarfism”), retinal degeneration, and increased sensitivity to sunlight. Depending on which gene is affected, two clinical phenotypes can be distinguished: type I (the “classic” type), with postnatal onset, and type II (the “severe” type), in which symptoms are present at birth and may lead to death by the age of 6 or 7 years. The skin manifestations include increased photosensitivity with peeling and scarring, and loss of subcutaneous fat. Mental retardation, microcephaly, severe neurological progressive impairment, sensorineural deafness, ataxia, spasticity, and intracranial calcifications are common neurological manifestations. Growth failure, major cachectic dwarfism, sexual immaturity, and dysmorphic features such as enophthalmos, beaklike nose, and narrow mouth and chin characterize the phenotype of Cockayne’s syndrome. As first described by Cockayne,74 one of the syndrome’s hallmarks is the pigmentary retinal degeneration found in 60% to 100% of patients. A “salt and pepper” type fundus, bone spicules, and optic atrophy have been reported. ERG shows reduced scotopic and photopic responses that appear to correlate with fundus changes and the age of the patient. Cataracts, which are present in 15% to 36% of patients, may be cortical, posterior subcapsular, or nuclear. Cataracts noted at birth or within the first 3 years of life indicate a poor prognosis, as do iris hypoplasia and microphthalmos. Enophthalmos due to lack of orbital fat is common, as are miotic pupils with a poor response to mydriatics. Strabismus, nystagmus, and decreased or absent lacrimation are also associated with the syndrome.75
Laboratory tests for (prenatal) diagnosis are available and allow for the differentiation of inherited DNA repair disorders. Results of the classic DNA repair test and post-UV radiation unscheduled DNA synthesis (UDS), a measure of the nucleotid excision repair (NER) pathway, are not abnormal in Cockayne’s syndrome. However, the results of the transcription blockage test, which examines recovery of RNA synthesis (RRS) 24 hours after UV exposure, will be abnormal.76 Treatment is symptomatic.
TRICHOTHIODYSTROPHY (TTD)
Ichthyosis (scaling of the skin), brittle hair and nails (sulfur-deficient proteins), and growth and mental retardation are manifestations of TTD. The clinical features of TTD are variable, although abnormalities are generally noted from birth. Photosensitivity is present in 50% of cases. As in Cockayne’s syndrome, skin cancer is not associated with TTD. Polarizing light microscopy shows a “tiger tail” pattern caused by a partial or complete absence of the cuticular layer, as seen under scanning electron microscopy. Similarly to patients with Cockayne’s syndrome, TTD patients may display a bird-like face, receding chin, beaked nose, protruding ears, and mild to severe growth retardation. Mental retardation, spasticity, tremor, ataxia, and neurodysmyelination are the neurological manifestations of TTD. Immunodeficiency and decreased fertility may be associated. Cataract is the main ophthalmic feature reported (mostly as small punctate opacities present throughout the lens, and rarely as zonular cataracts).77 Decreased lacrimation, pigmentary degeneration of the retina, nystagmus, and optic disk atrophy (associated with encephalopathy) are rarely associated. Brittle eyelashes may induce keratitis due to abnormal orientation of the lashes. DNA testing on fibroblast cultures shows various results depending on the pathway involved, and may not be diagnostic in 50% of cases. Prenatal diagnosis by fetal hair biopsy has been reported. Three genes have been identified as causing TTD: the yet-uncloned TTD-A78; TTD-B, a rare mutation in the XP-B gene; and TTD-D, caused by mutations on XP-D gene.79 The location of a mutation in the XP-B or XP-D gene appears to determine the clinical phenotype.80 Recently two patients with clinical symptoms of both XP and TTD were reported.81
ROTHMUND-THOMSON’S SYNDROME
Rothmund-Thomson’s syndrome is characterized by early poikiloderma, growth deficiency, and increased predisposition to cancer, (most commonly osteosarcoma). Skin manifestations begin as large, ill-defined areas of erythema that occur shortly after birth. The erythema leads to atrophy of the skin, with telangiectasias and hyper- and hypopigmentation aggravated by exposure to light. Most patients show growth retardation, dystrophy of the nails and teeth, hypogonadism, and alopecia. Early graying, hair loss, and absence or sparseness of eyelashes and eyebrows are common. Bilateral cataracts have been reported to be a common feature (73% in one large series82). The lens opacities are subcapsular, and display rapid onset within 2 to 3 months, most commonly at the age of 2 to 4 years. In a recent series involving 41 affected individuals, however, only two patients with cataracts were identified.83 Occasionally bilateral glaucoma, retinal coloboma, and chorioretinal atrophy have been described.84 Rothmund-Thomson’s syndrome is caused by mutations in the DNA helicase gene RECQL4.85 Therapy is limited to early detection of osteosarcoma with baseline radiographs after the age of 5 years, and avoidance of sun exposure.
WERNER’S SYNDROME
Premature aging, endocrine disturbances (e.g., diabetes and hypogonadism), and osteoporosis (progeria of the adult) are hallmarks of Werner’s syndrome. The syndrome typically manifests after puberty. Arteriosclerosis and osteoporosis are common, and myocardial infarction or cancer may lead to early death in the fourth to sixth decades. The skin becomes shiny and taut, with atrophy of the underlying subcutaneous tissue. The hair often grays before the patient reaches 20 years of age. Loss of orbital fat leads to deep-set eyes, and the nose becomes beak-shaped. Bilateral cataracts appear at a median age of 30 years.86 Subcapsular, cortical, nuclear, zonular, and punctate lens opacities have been reported. Bullous keratopathy is a common postoperative complication and may necessitate penetrating keratoplasty.87 Werner’s syndrome is caused by mutations in the RECQL2 gene encoding for a DNA helicase.88
DISORDERS WITH DERMATOLOGIC MANIFESTATIONS
ANHIDROTIC ECTODERMAL DYSPLASIA (CHRIST-SIEMENS-TOURAINE’S SYNDROME)
Also known as Christ-Siemens-Touraine’s syndrome, this rare disease presents as a congenital absence of the sweat, sebaceous, and mucous glands, with sparse hair, poorly developed teeth, and growth retardation. Ocular symptoms vary from congenital anophthalmos,89 keratopathy, and adult onset cataract due to anti-lens antibodies90 to atrophic rhinitis without ocular involvement. Hypohidrotic/anhidrotic ectodermal dysplasia (HED/EDA) has been ascribed to at least three genes involved in NF-κ-B activation: ectodysplasin (EDA1), EDA-receptor (EDAR), and EDAR-associated death domain (EDARADD). During hair follicle morphogenesis, EDAR is activated by ectodysplasin and uses EDARADD as an adapter to build a signal transducing complex that leads to NF-κ-B activation.91
Two further mutations in the NF-κ-B family are known to cause anhidrotic ectodermal dysplasia with immune deficiency: X-linked recessive anhidrotic ectodermal dysplasia with immunodeficiency (XL-EDA-ID) is caused by hypomorphic mutations in the gene encoding NEMO/IKK-gamma, the regulatory subunit of the I-κ-B-kinase (IKK) complex. AD EDA-ID (AD-EDA-ID) is caused by a hypermorphic mutation in the gene encoding the inhibitory protein IκBα92.
INCONTINENTIA PIGMENTI (IP, BLOCH-SULZBERGER’S SYNDROME)
IP (Bloch-Sulzberger’s syndrome) was the first genetic disorder to be ascribed to NF-κB dysfunction. IP is an X-linked dominant genodermatosis antenatally lethal in males. Females present with abnormal skin pigmentation (100%), dental (90%), skeletal (40%), central nervous (40%) and ocular (35%) abnormalities. Other dermatological signs include alopecia and nail dystrophy. Ocular abnormalities consist of proliferative vitreoretinopathy, retinal detachment, strabismus, congenital cataract, microphthalmia, optic nerve atrophy, and iris hypoplasia. A complex rearrangement of the NEMO (NF-κB essential modulator) gene accounts for 85% of IP patients, and results in undetectable NEMO protein and absent NF-κB activation.91
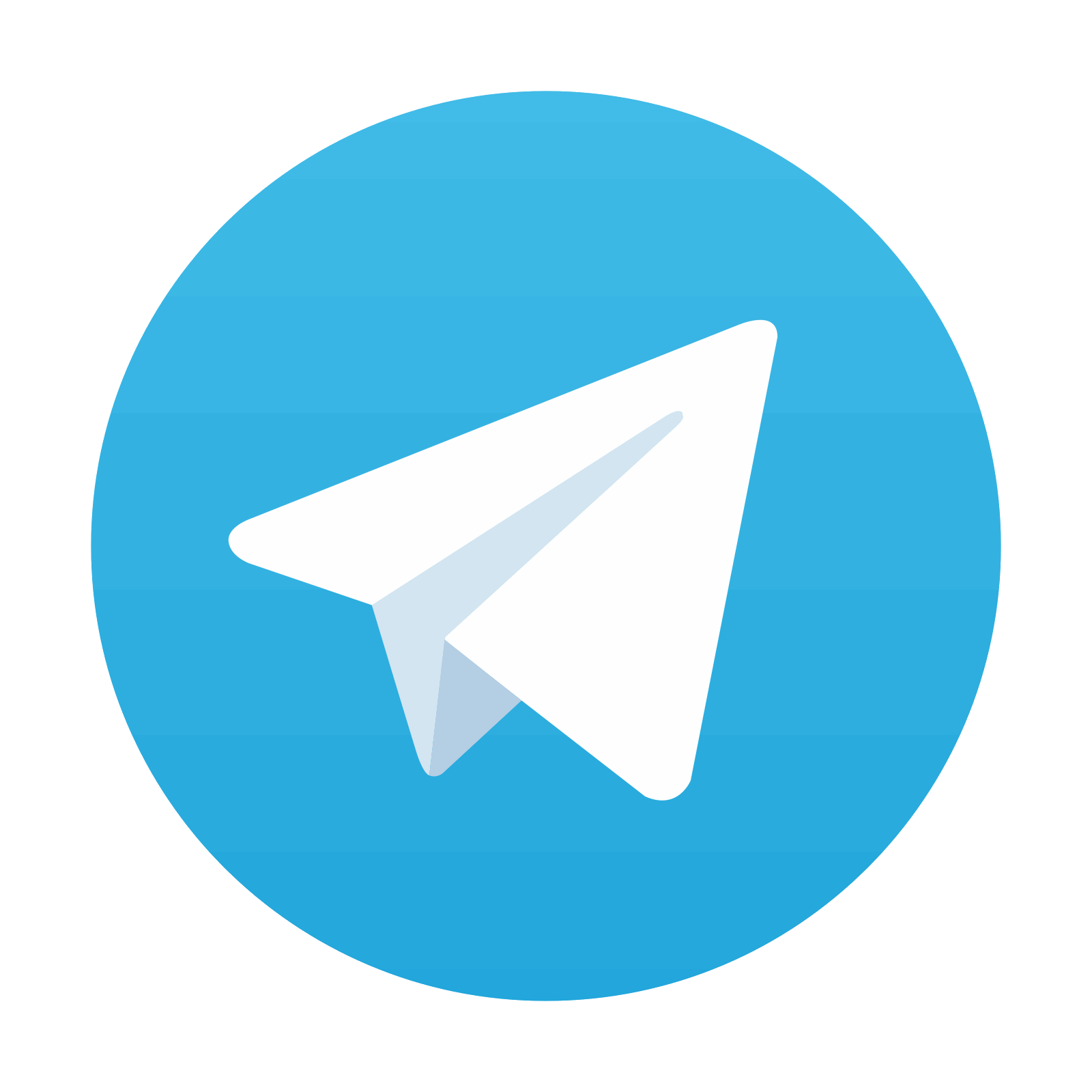
Stay updated, free articles. Join our Telegram channel

Full access? Get Clinical Tree
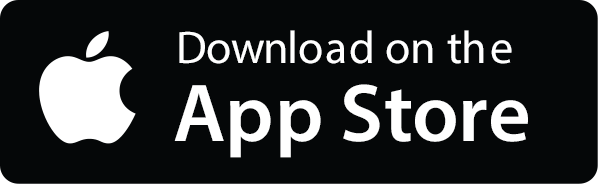
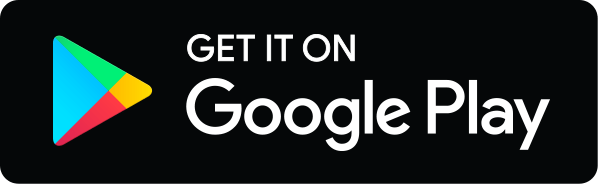