Fig. 6.1
Diabetes is a vascular disease that can obliterate the capillary bed in the retina. Diabetic retinopathy begins with hemorrhages in the blood vessels around the retina (pictured above as red spots in the mild, or non-proliferative stage). The retinal tissue responds to ischemia by producing a high level of vascular endothelial growth factor (VEGF). Following this, abnormal blood vessels begin to form (white arrow, bottom left), a process called neovascularization. The new vessels are unusually fragile and may produce further hemorrhaging (bottom center), a precursor to widespread scarring (bottom right). Ultimately, diabetic retinopathy may lead to retinal detachment
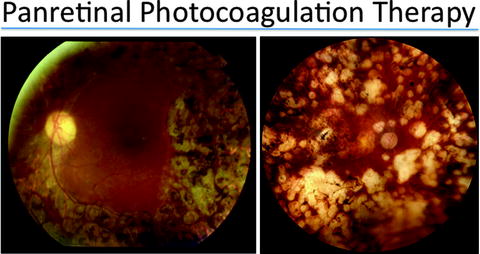
Fig. 6.2
Panretinal photocoagulation has been successful in treating diabetic retinopathy. Through this laser treatment, the ischemic retina is ablated and the production of vascular endothelial growth factor (VEGF) is slowed. The mechanism for this treatment involves 1200 to 1600 tiny laser burns scattered throughout the outer retina, eliminating photoreceptors and RPE. After panretinal photocoagulation, areas of inner retina formerly deprived of oxygen due to the poor perfusion of inner retinal vessels begin to receive more oxygen. A decreased number of hypoxic cells decreases VEGF, halting neovascularization. The macula, or the center of the retina, is typically not treated with laser (right), but in some cases, laser may also be applied to this area (left)
Caballero et al. [21] examined the effects of intraocular transplantation of human CD34+ cells in rodent models of ischemic retinal disease, including neonatal hyperoxia-induced retinopathy, retinal ischemia-reperfusion in adult mice (temporary central retinal artery occlusion induced by ophthalmic hypertension) and streptozocin-induced diabetes in adult rats. Transplanted cells co-localized with damaged host vasculature in all three models and vessel caliber and patency was improved in transplanted eyes compared to controls. Interestingly, there was no qualitative difference between intraocular and systemic route of administration, consistent with the ability of these cells to migrate to sites of tissue injury from peripheral blood.
Interestingly, BMSC transplantation may have applications in non-ischemic retinal disease. Otani et al. [22, 23] studied the effects of intravitreal injection of BMSCs in two models of inherited retinal degeneration, the rd1 mouse and the rd10 mouse. In both models, bone marrow-derived Lin− cells improved retinal thickness, retinal vascular density, and retinal function as measured by ERG. These findings suggest that transplantation of these cells may have effects not directly attributable to angiogenesis. In support of this hypothesis is the finding that BMSC-derived cells found at the site of RPE damage differentiate into RPE cells [14]. Genetic analysis of these cells suggest that this is not the result of fusion of bone marrow-derived cells with unlabeled, differentiated host cells.
If BMSCs capable of mitigating damage from eye injury already exist in the body normally, why would therapeutic transplantation be beneficial? One simple rationale is that the normal reparative response is limited by the number of available BMSCs. Since BMSCs can be expanded ex vivo, greater numbers of these cells may augment the normal host response [24]. A second rationale is that certain pathologic settings, such as diabetes, could affect the availability or ability of BMSCs to participate in restorative anigogenesis. Fewer HSCs are found in the peripheral blood of diabetic human subjects [25, 26] and in rats with experimental diabetes [27], suggesting that fewer of these stem cells may be available to participate in injury response in peripheral tissues. Hyperglycemia has been shown to impair survival and function of circulating blood-derived progenitor cells in vitro [28]. Further, transplantation of CD34+ cells isolated from diabetic subjects did not show beneficial effects seen with cells from nondiabetic subjects in rodent models of ischemic retinopathy [21].
Conclusion
Recent advances in our understanding of the role of BMSCs in neovascularization have refined our understanding of the response to tissue injury and laid the foundation for BMSC-based therapeutic angiogenesis. Furthering our knowledge of the roles of specific subsets of BMSCs in angiogenesis and the factors that regulate the process will be critical to further development of this therapeutic approach. BMSC-based therapy holds promise for the treatment of ischemic retinal disease, but special consideration must be given to the potentially deleterious effects of pathologic neovascularization seen in these diseases. Future work will hopefully help improve our understanding of and ability to manipulate differences between pathologic and therapeutic angiogenesis in retinal disease.
References
1.
Srour EF et al (1991) Human CD34+ HLA-DR− bone marrow cells contain progenitor cells capable of self-renewal, multi-lineage differentiation, and long-term in vitro hematopoiesis. Blood Cells 17:287–295PubMed
2.
Sutherland HJ et al (1989) Characterizaion and partial purification of human marrow cells capable of initiating long-term hematopoiesis in vitro. Blood 74:1563–1570PubMed
3.
4.
Asahara T et al (1997) Isolation of putative progenitor endothelial cells for angiogenesis. Science 275:964–967PubMedCrossRef
< div class='tao-gold-member'>
Only gold members can continue reading. Log In or Register a > to continue
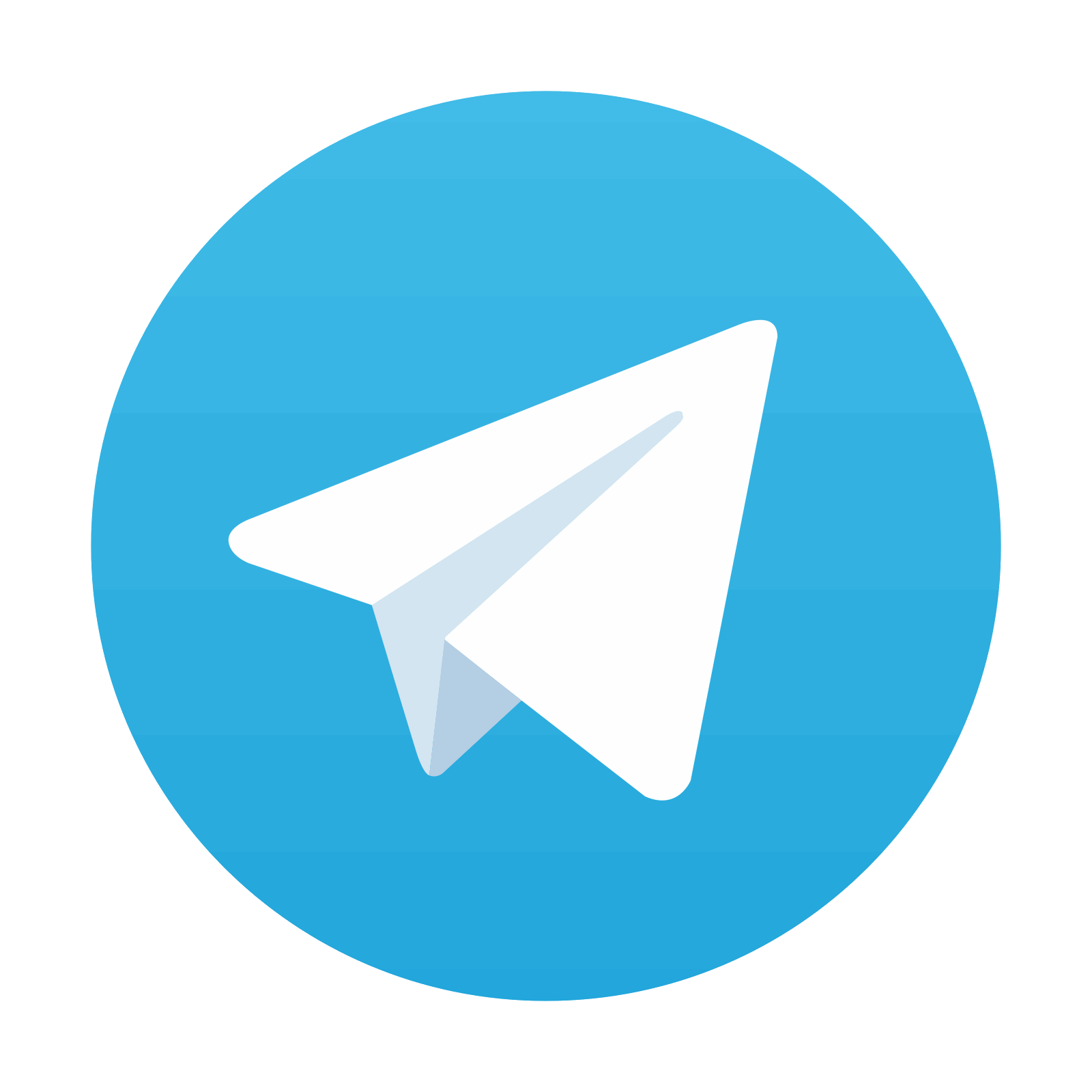
Stay updated, free articles. Join our Telegram channel

Full access? Get Clinical Tree
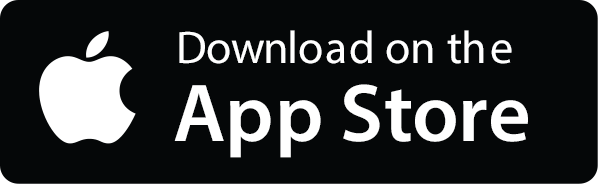
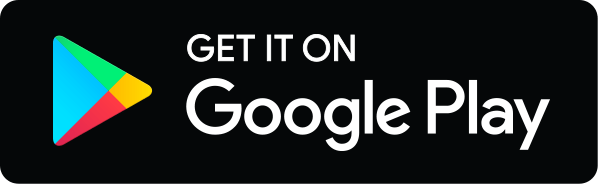