(1)
Department of Ophthalmology and Visual Sciences, University of Iowa Hospitals and Clinics, Iowa City, IA, USA
Leber [1] in 1903 first wrote the classical description of the vascular system of the eye; it was mainly based on whole mount specimens injected with India ink or gelatin dye in the blood vessels. Subsequent studies have mostly either confirmed or supplemented his findings.
It is well established that the anterior segment of the eye is supplied by the anterior ciliary arteries (ACAs) and long posterior ciliary arteries (long PCAs).
Anterior Ciliary Arteries
ACAs are branches of the muscular arteries in the four recti. I investigated the arteries to the four recti by intra-arterial injection of liquid latex in the late 1950s in 59 human cadaver orbits [2]. My study showed that the major source of muscular arteries to the various recti are branches of the medial and lateral muscular arteries, which in turn are branches of the ophthalmic artery; less common are those that arise either directly from the ophthalmic artery or from its other branches. Their site of origin from the ophthalmic artery, mode of origin, and the muscle(s) supplied by them are discussed at length elsewhere [2]. A medial muscular artery was present in all specimens, but the lateral muscular artery was seen in only 17 %. The medial muscular artery is a big artery and arises directly from the ophthalmic artery as an independent branch in 74 % and in the rest mostly in common with the posterior ciliary artery (PCA). In some specimens, the muscular artery to one or more of the recti arises from the ophthalmic artery by a common trunk with one of the PCAs. The medial muscular artery usually supplies the medial, lateral, and inferior recti, and the lateral muscular artery usually supplies the lateral and superior recti [2]. Table 6.1 shows the number of arterial branches to the various recti seen in my study [2].
Name of rectus muscle | One branch (%) | Two branches (%) | Three branches (%) | Four branches | Five branches |
---|---|---|---|---|---|
Lateral rectus | 40 | 45 | 12 | 5 % | None |
Superior rectus | 53 | 36 | 12 | None | None |
Medial rectus | 19 | 12 | 45 | 22 % | 2 % |
Inferior rectus | 90 | 8 | 2 | None | None |
When a muscular artery arises in common with a PCA, this has clinical importance, because giant cell arteritis almost invariably involves the PCAs [3–20]. In such a situation, if the common trunk of the muscular artery and PCA is involved by the giant cell arteritis and occluded, it can result in ischemia of the rectus/recti supplied by the occluded artery; that manifests as diplopia – a well-known symptom of giant cell arteritis. Since ACAs are branches of the muscular arteries, their occlusion can result in anterior segment ischemia, which has been reported in giant cell arteritis by many studies [21–47].
I also investigated the muscular arteries to the recti in rhesus monkeys by intra-arterial liquid neoprene latex injection [48]. As in human specimens (see above), there were three modes of origin of the muscular arteries to the recti from the ophthalmic arteries:
1.
The Medial Muscular Artery: As in humans, this is a big artery, and it usually supplies the medial, inferior, and lateral recti. In the specimens I studied, I did not find any lateral muscular artery, but that may be due to study of a small sample.
2.
Independent Muscular Arteries Arise Directly from the Ophthalmic Artery: They always supply the superior and medial recti and rarely the lateral rectus.
3.
Muscular Branches Arising from Other Orbital Branches of the Ophthalmic Artery: They may supply one or more recti.
Leber [1] reported that there are usually two ACAs associated with each rectus, except the lateral rectus which has only one. Ashton and Smith in 1953 [49] in their human cast studies described the ACAs after leaving the surface of the sclera, dividing into the following branches (Fig. 6.1):
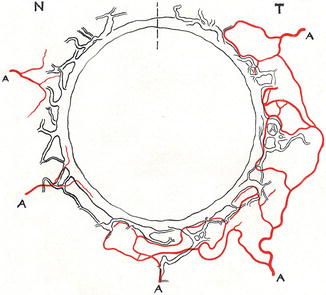
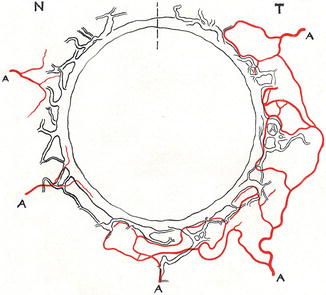
Fig. 6.1
Drawing of the neoprene cast of the canal of Schlemm and diagrammatic representation of incomplete arterial circle close to the canal. Dotted vertical line above indicate superior part of the eyeball (Reproduced from Ashton and Smith [49]) A artery, N nasal, T temporal
1.
Small Episcleral Twigs: These form the episcleral limbal plexus and send branches to the conjunctiva.
2.
Small Intrascleral Branches: They contribute to form an incomplete arterial circle of the canal of Schlemm, situated in close proximity to the canal (Fig. 6.1). There was no afferent connection between the canal and the arterioles, and they do not deal with the aqueous flow.
3.
Large Terminal Perforating Branches: These give branches to the vascular plexus in the ciliary muscle, and within the ciliary body, they give branches anteriorly to the major arterial circle of the iris and posteriorly to the anterior choroid. These recurrent choroid branches were first described by Leber [1] in 1903, and subsequently by many other studies. They also reported that the ACAs and their branches typically run in company with the veins. This arrangement is seen all over the sclera, and the small arterial branches running toward the canal of Schlemm not infrequently use the same scleral tunnel as the anastomosing outlets of the canal.
In the literature dealing with the anatomy of the ACAs, there are several reports based on scanning electron microscopy studies of casts. In rhesus monkeys, Shimizu and Ujiie [50] reported that the ACAs are located in the episcleral tissue and perforate the sclera to enter the ciliary body a few millimeters behind the corneal limbus. According to them, the ACAs did not communicate with the major arterial circle of the iris but divided into numerous branches to supply the ciliary muscle in the vicinity of the site of perforation. However, others [49, 51] have stated that perforating branches of the ACAs terminate in the anterior part of the ciliary body and anastomose with long PCAs to form the major arterial circle of the iris. Van Buskirk [51], like Leber [1], reported that in rhesus monkeys there are seven ACAs which arise from the recti (two from each rectus except one from the lateral rectus – Fig. 6.2). After leaving the rectus muscle, the ACAs first supply the episcleral tissues, and then perforate the sclera and anastomose with the long PCAs. ACAs arising from the lateral rectus often have no perforating branches [51, 52]. After leaving the rectus muscles, the ACAs interconnect via their lateral-most branches in the episclera at the limbus to form the episcleral circle [52]. Branches of the ACAs perforate the limbal sclera and enter the ciliary muscle. Heymann et al. [53] studied ACAs emerging from the 4 recti in a radioanatomical study of 25 human eyes. They found that there were numerous individual variations. Following were the predominant findings: the ACA from the lateral rectus is usually thin and located in the superior or inferior third of the muscle. In other recti, the ACAs were rather big and frequently observed in pairs. They tend to be found on each lateral side of the muscle. By and large, vertical ACAs look bigger and more numerous than the horizontal ACAs. Johnson et al. [54], on a photographic analysis of ACA distribution in the distal parts of 20 lateral and 22 medial rectus muscles of patients, found one major artery in the lateral rectus in 15 % and in the medial rectus in 18 %, two major arteries in the lateral rectus in 50 % and in the medial rectus in 59 %, and more than two major arteries in the lateral rectus in 35 % and in the medial rectus in 23 %. This showed that there was no significant difference in the mean number of major ACAS between the lateral and medial recti. Funk and Rohen [55] in human eyes reported that the perforating branches of the ACA form an intramuscular arterial circle in the posterior region of the ciliary muscle and supply the outer and posterior parts of the ciliary muscle, partly the iris, and the peripheral choroid by recurrent ACA branches.
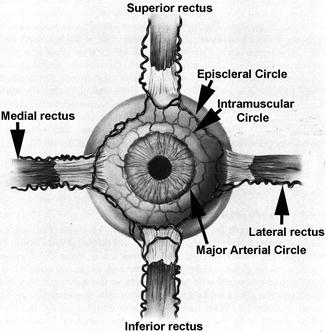
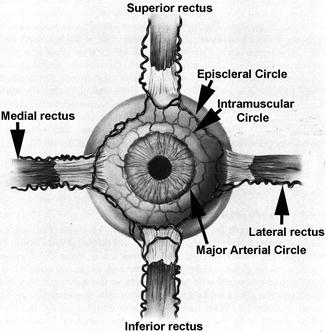
Fig. 6.2
Diagrammatic representation of anterior ciliary arteries running along the borders of the four recti muscles and also of episcleral, intramuscular, and major arterial circles (Modified from Van Buskirk [51])
There are several fluorescein angiographic studies of the ACAs. Meyer [56] on angiography of 13 subjects found the incidence of arteries the lowest over the lateral rectus muscle. Ormerod et al. [57] found marked individual variability and much larger vertical ACAs. Laatikainen [58], in a study of the temporal perilimbal area of 156 eyes, concluded that the first vessels to show fluorescein filling are the branches of the ACAs that are coming from the lateral, inferior, or superior rectus muscles. Meyer and Watson [59] reported that the positions of the ACAs are inconstant as they run radially toward the limbus within Tenon’s capsule. During their course over the anterior part of the globe, they usually branch little, if at all. Close to the limbus, they give rise to superficial (anterior episcleral) and deep (scleral) divisions. The former continue in the superficial or deeper episclera. The latter penetrate the sclera and disappear from view. They found that the scleral contributions were more prominent nasally and the episcleral divisions temporally. These anatomical findings indicate marked interindividual variation in the number and distribution of ACAs, particularly the ACA from the lateral rectus.
Direction of Blood Flow in the ACAs
The direction of blood flow in ACAs in normal eyes on fluorescein angiography is controversial. Some have reported that the blood flow in the ACAs is from inside the globe toward the site of attachment of the recti [60–64], while others have reported that it is centripetal in direction from the recti throughout their course till their perforating branches pass through the sclera [60, 61, 65–67]. Still others have found the flow to be centripetal in some eyes and from inside the eye out in other; for example, Meyer [56] found that in 25 of 40 arteries the flow was away from scleral perforations. Because of the blood flow direction from inside the globe outward, some regarded the filled vessels from inside the eye as veins [62–64]. Shimizu and Ujiie [50], based on their cast studies, reported them as definitely arteries and not veins. Talusan and Schwartz [60] on fluorescein angiography of 12 eyes found no difference in the filling of the ACAs by fluorescein by quadrant; and approximately 4 s after the initial appearance of the dye in the ACA, the anterior ciliary vein showed laminar filling. Meyer [56] on angiography of 13 subjects found veins were concentrated in the vertical meridian and were absent over the lateral rectus in 8 subjects, and all 8 veins drained away from the limbus. Ormerod et al. [57], based on scanning angiographic microscope study of 37 scleral perforating arteries, stated that reports of retrograde blood flow in the ACAs in most fluorescein angiographic studies are probably incorrect, being the result of unappreciated methodological problems.
Since the ACAs arise from the recti, one would normally expect that their blood flow should be from the muscles toward the limbus, but, as discussed above, some fluorescein angiographic studies have shown that it is the reverse. This paradoxical phenomenon is puzzling. Van Buskirk [51], based on his cast studies, postulated the following explanation. Flow direction in the cerebral circle of Willis can reverse when intraluminal occlusions alter relative pressure relationships in the cerebral vascular system. Moreover, regional shifts in cerebral blood flow have been demonstrated with localized cerebral activity [68–72]. According to him, “By the same token, it could be postulated that since at least two complete anterior anastomotic circles exist in the anterior segment, each fed by diverse arterial trunks, spontaneous changes in flow direction may occur in response to tissue demands of the extraocular muscles, the ciliary muscle, the anterior uvea, intraocular pressure, and arterial blood pressure within the individual arterial trunks.” Direct connections between the perforating branches of ACAs and the major circle of the iris “may facilitate development of ‘reverse’ flow in the anterior ciliary arteries with increased pressure within the eye or in the long posterior ciliary artery.”
Nanba and Schwartz [73] found a significant positive correlation between the diameter of the ACA and intraocular pressure. They postulated that the increase in intraocular pressure may influence the circulation of the anterior uvea, resulting in decreased pressure in the ACA and an increase in diameter of the ACA. They put forward this postulate to explain the fluorescein angiographic finding of blood flow in the ACA from the inside of the eye to the outside.
However, none of these postulates fully explain the reverse blood flow documented on fluorescein angiography in ACAs in perfectly normal eyes.
Some of the ACAs, before penetrating the sclera, give branches to the intrascleral and episcleral vascular system. Some of these vessels join the so-called aqueous veins. The relationship between aqueous outflow and blood flow in the limbal vessels also plays an important role in intraocular pressure regulation [60, 74–77]. Thus, there is a close correlation between episcleral circulation and intraocular pressure regulation [78, 79].
Long Posterior Ciliary Arteries
Terminology of PCAs and Its Implications
In the literature, there has been confusion in the nomenclature applied to the PCAs. Most investigators while describing their findings have used the term “PCA” loosely and as a generic term, covering all types of PCAs and their branches. Others have designated them the “long” PCAs right from their point of origin from the ophthalmic artery. I investigated various anatomical aspects of PCAs in 59 human orbits. The number, and mode and site of origin of PCAs, is discussed at length elsewhere [2]. My studies showed that the old nomenclature is misleading. The main PCAs arise from the ophthalmic artery (Figs. 6.3, 6.4, and 6.5). The ophthalmic artery gives out one to five main PCAs (one in 3 %, two in 48 %, three in 39 %, four in 8 %, and five in 2 %) [2]. Usually two or three main PCAs supply an eye (Fig. 6.6). The main PCAs usually lie medial and lateral to the optic nerve and hence are called medial (one in 71 % and two in 29 %) and lateral (one in 75 %, two in 20 %, three in 2 %, none in 3 %) PCAs; small and inconstant superior PCAs were seen in 9 % (one in 7 % and two in 2 %). The main PCAs divide into multiple branches before entering the eyeball (Figs. 6.6 and 6.7) and are named as follows:
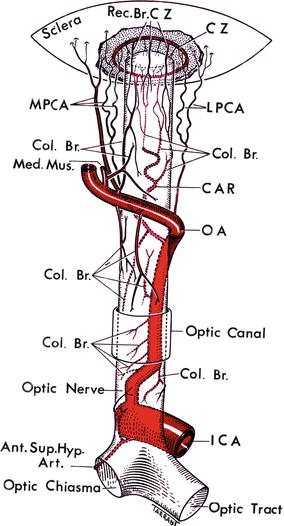
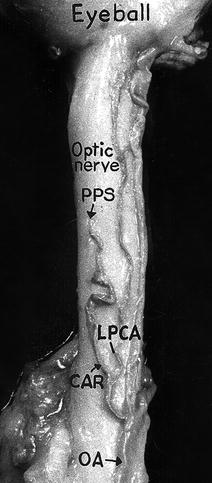
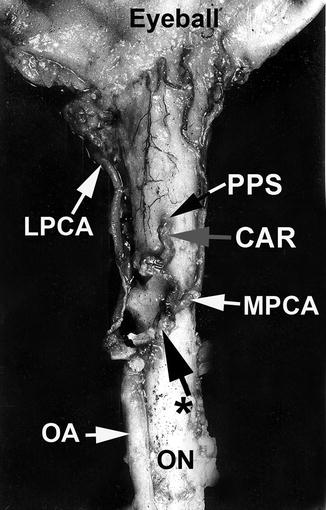
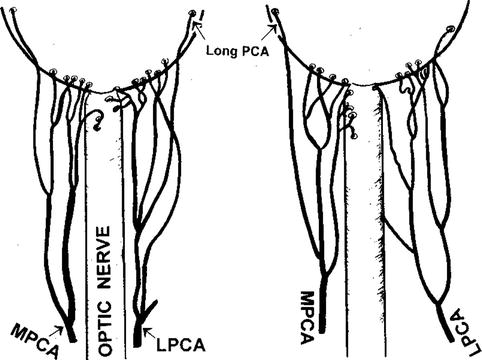
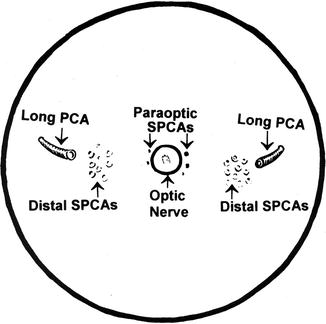
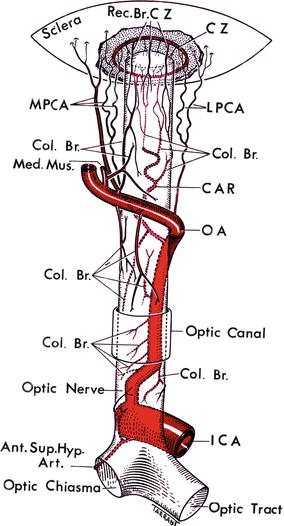
Fig. 6.3
A diagrammatic representation of the origin, course, and branches of the ophthalmic artery (reproduced from Hayreh [80]). Ant. Sup. Hyp. Art. anterior superior hypophyseal artery, CAR central artery of the retina, Col.Br. collateral branch, CZ circle of Haller and Zinn, ICA internal carotid artery, LPCA lateral posterior ciliary artery, Med. Mus. medial muscular artery, MPCA medial posterior ciliary artery, OA ophthalmic artery, Rec.Br.CZ recurrent branches of the circle of Haller and Zinn
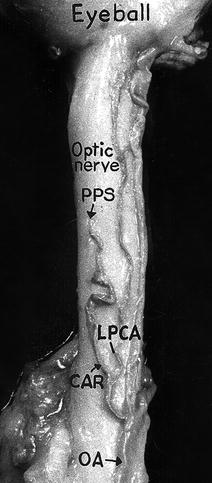
Fig. 6.4
The central artery of the retina arising by a common trunk with lateral posterior ciliary artery from the ophthalmic artery, as seen from the inferior surface of the optic nerve (Reproduced from Hayreh [81]). CAR central artery of the retina, LPCA lateral posterior ciliary artery, OA ophthalmic artery, PPS point of penetration into the sheath by the central retinal artery
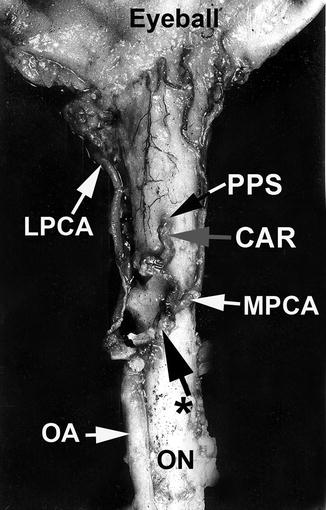
Fig. 6.5
The central artery of the retina and medial posterior ciliary artery arising by a common trunk from the ophthalmic artery, as seen from below (Reproduced from Hayreh [81]). CAR central artery of the retina, LPCA lateral posterior ciliary artery, MPCA medial posterior ciliary artery, OA ophthalmic artery, ON optic nerve, PPS point of penetration into the sheath by the central retinal artery, asterisk common trunk of origin of the central retinal artery and MPCA
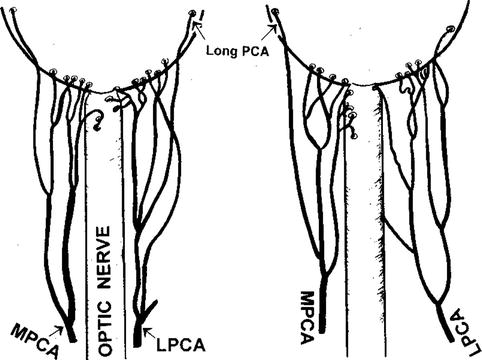
Fig. 6.6
Diagrammatic representation of actual branching pattern of medial (MPCA) and lateral (LPCA) PCAs in two eyes (Reproduced from Hayreh [84])
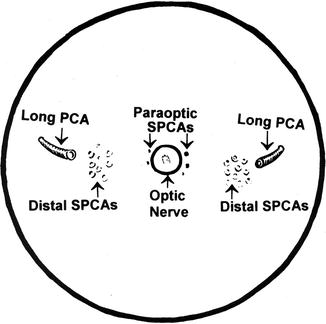
Fig. 6.7
Diagrammatic representation of the site of entry of the various long and short PCAs (SPCAs), as seen on the back of the eyeball (Reproduced from Hayreh [84])
1.
2.
Short PCAs: They may be any number, up to 20 or so (Figs. 6.6 and 6.7). They are further subdivided into two subgroups [82–84]:
(i)
(ii)
Distal Short PCAs: The majority of the short PCAs belong to this group and enter the eyeball midway between the paraoptic short PCAs and long PCAs (Figs. 6.6 and 6.7). On the temporal side, they enter the eyeball in the region of the macula (Fig. 6.8). They mainly supply the choroid.
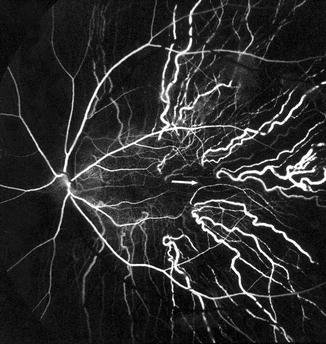
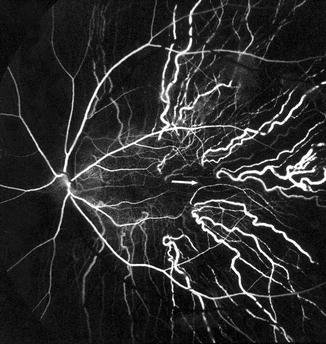
Fig. 6.8
Fluorescein fundus angiogram of a normal human eye, showing the sites of entry of the short PCAs and their course in the choroid. Arrow marks the center of the macular region. Note that no artery lies in the center of the macular region (Reproduced from Amalric [85])
From this account, it is evident that there may be 1–5 main PCAs and then three sets of branches of the PCAs supplying three different areas.
The textbook description of the long PCAs has been virtually unchanged since the early accounts of Leber [1] in 1903, Salzmann [86] in 1912, and other authors at the beginning of the twentieth century. The arteries pierce the sclera nasal and temporal to the optic nerve (the former 3.6 and the latter 3.9 mm from the optic nerve; Duke-Elder and Wybar [87]; Hogan et al. [88]) and run horizontally at first in a scleral canal (3–7 mm long, Leber [1]; 3–5 mm long, Hogan et al. [88]) and then in the suprachoroidal space, as far forward as the posterior part of the ciliary body, where each artery divides into two main divisions – superior and inferior. The superior and inferior divisions of the two long PCAs unite to form the major arterial circle of the iris in the anterior part of the ciliary body behind the root of the iris. Of the ACAs, some join the greater arterial circle of the iris and others the long PCAs or their branches [86]. The long PCA, according to all these descriptions, gives no branches throughout its entire course until it divides into its two main divisions in the ciliary muscle. The greater arterial circle of the iris, ACAs, and the terminal part of the long PCAs give recurrent choroidal branches, which supply the anterior part of the choroid up to the equator and anastomose with the SPCAs at the equator [89, 90]. No anastomoses between the SPCAs and the recurrent choroidal arteries at the equator have been found in rabbits [1, 91]. Thus, according to all the available descriptions, the long PCAs supply the choroid only in front of the equator via the recurrent choroidal arteries, with no supply to the choroid behind the equator.
I [92] investigated the distribution of the temporal long PCA in the choroid in 22 rhesus monkey eyes by fluorescein fundus angiography, after the following experimental procedures:
Group 1: The temporal long PCA was cauterized outside the eyeball near its site of penetration into the sclera in nine eyes. The accompanying ciliary nerves were carefully separated from the artery and left intact. The rest of the ocular circulation was left undisturbed. None of the rectus muscles was cut, in order to prevent interference with the anterior ciliary arterial circulation.
Group 2: All the temporal SPCAs were cauterized outside the sclera close to their site of penetration into the sclera in 13 eyes. The temporal long PCA was left intact. As in group 1, the rest of the ocular circulation was also left intact.
Temporal Long PCA Supply to the Choroid
Contrary to the prevalent classical concept, in vivo study in rhesus monkeys revealed that the long PCA invariably supplies an area of the choroid posterior to the equator. The temporal long PCA joins the choroid 5 mm temporal to the optic disc and the area of the choroid supplied by the artery starts 4.6–9 mm (average 6.2 ± 1.0 mm) from the disc. The distance of the equator and ora serrata on the temporal side from the optic disc in rhesus monkeys is 13 and 16.5 mm, respectively. This shows that the supply by the long PCA to the choroid extends well posterior to the equator. The distribution is sectoral, with the apex of the sector pointing backward (Figs. 6.9, 6.10, and 6.11). The distribution by the artery is segmental, with little direct anastomosis with the adjacent choroidal arteries. On follow-up of the eyes with occluded temporal long PCA, however, no fundus lesion was seen. These two statements may seem to contradict one another. The explanation for the nonoccurrence of fundus lesions on experimental occlusion of small branches of the PCAs is discussed elsewhere [93].
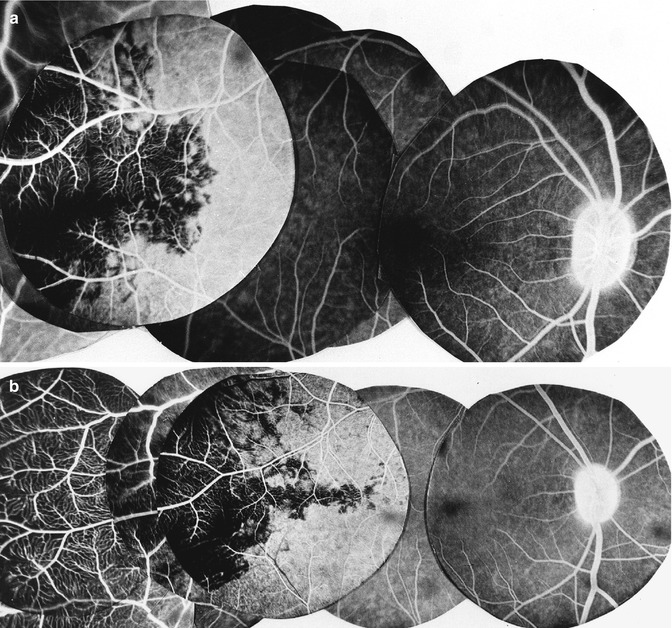
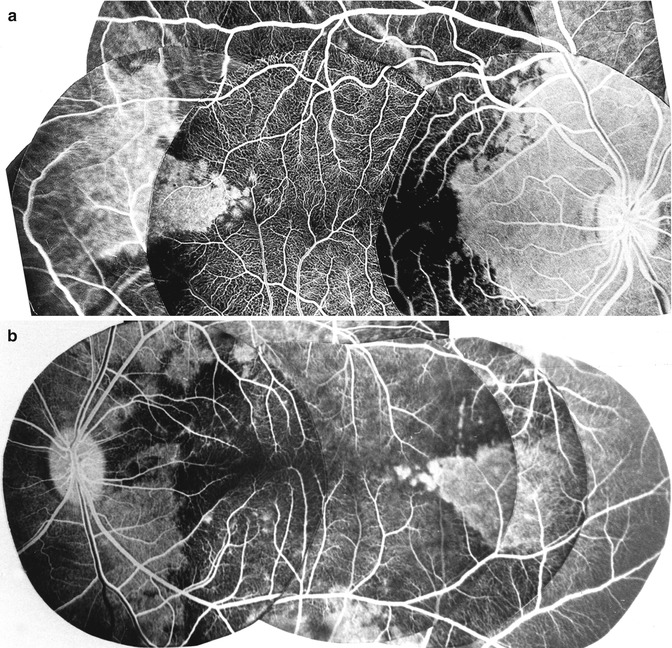
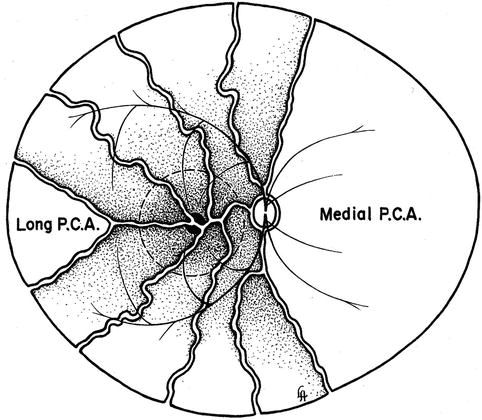
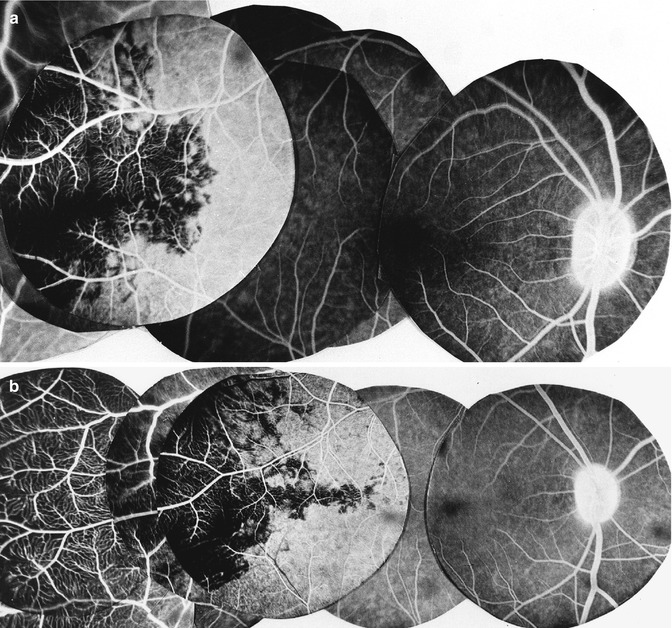
Fig. 6.9
(a, b) Two composite fluorescein fundus angiogram of rhesus monkey right eyes showing a sectoral filling defect in the posterior choroid, situated temporal to the macular region, after cutting the temporal long PCA (Reproduced from Hayreh [92])
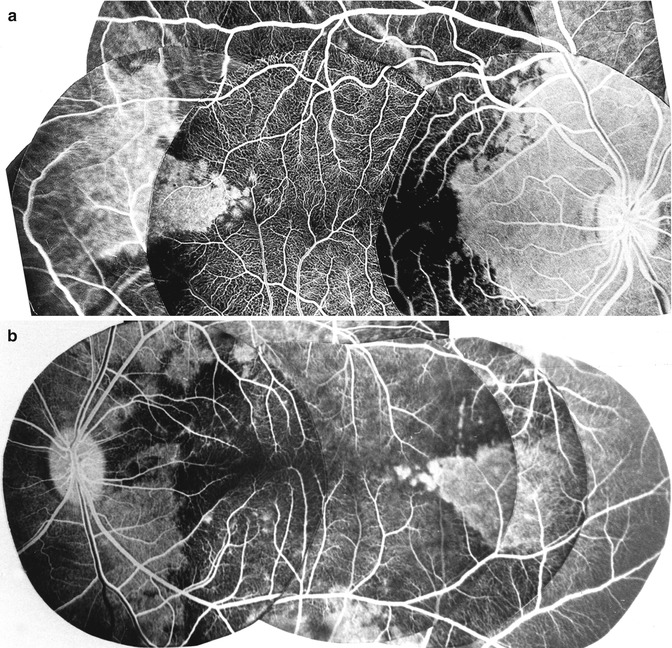
Fig. 6.10
(a, b) Two composite fluorescein fundus angiogram of rhesus monkey eyes (a of the right eye; b of the left eye) showing the filling of a sector of the posterior choroid temporal to the macular region in the area supplied by the temporal LPCA, when all the temporal short PCAs were cut. Non-fluorescence of the choroid in the extreme temporal periphery is an artifact (Reproduced from Hayreh [92])
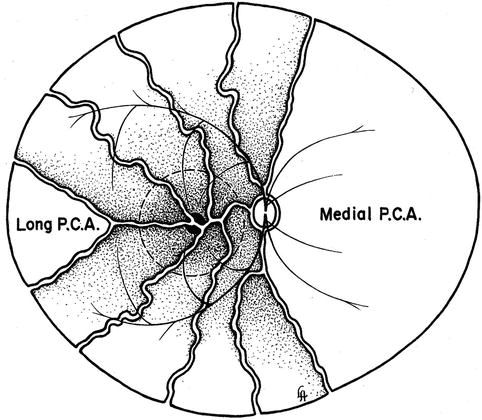
Fig. 6.11
Diagrammatic representation of distribution by various temporal short PCAs and long PCA in the posterior part of the fundus. Dotted circle in the region of distribution of temporal short PCAs represents macular region. Area of supply by medial PCA is also shown (Reproduced from Hayreh [92])
Shimizu and Ujiie [50] in their study of vascular casts of rhesus monkey eyes found that the long PCA runs a long, oblique intrascleral course till it makes a sharp bend to enter the choroid temporal to the fovea on the temporal side and that it supplies a triangular-shaped sector of the choroid by a number of local branches. In the temporal fundus beyond the equator, recurrent arteriolar branches from the long PCA supply adjacent small choroidal areas. Thus, these cast studies show the same choroid distribution by the long PCA as seen in my study. Song et al. [94] found in humans that the long PCA formed several branches before entering the iris root and those branches formed the major arterial circle of the iris with diverse diameters, in the vicinity of the iris root and the ciliary process. The watershed zone in the equatorial region shown in Fig. 6.12 was also demonstrated on wide-angle indocyanine green angiography using a scanning laser ophthalmoscope in humans [96].
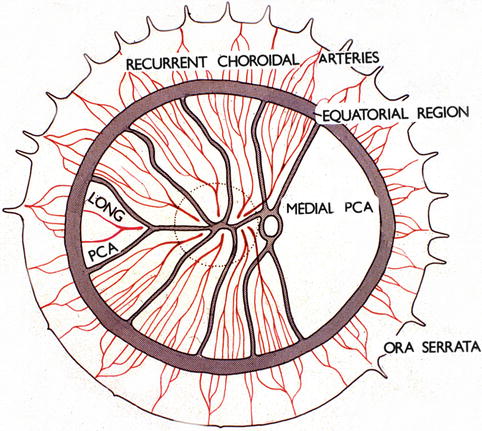
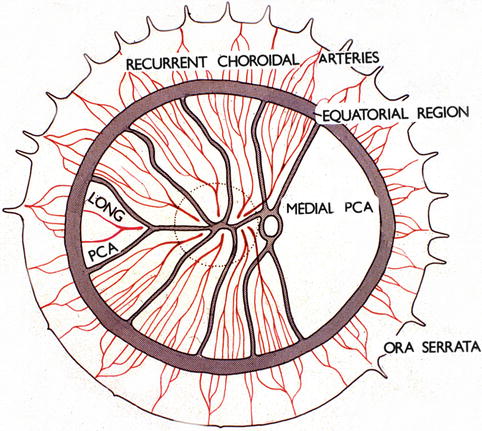
Fig. 6.12
Diagrammatic representation of the distribution by the various ciliary arteries in the choroid and their watershed zones. The choroid posterior to the equator is supplied by the medial and lateral PCAs. In the area supplied by the lateral PCA are shown the segments supplied by the various short PCAs and the one by the long PCA, with the watershed zones between them (dotted circle in this area indicates the macular region). Recurrent choroidal arteries from the anterior ciliary arteries and supposedly the greater arterial circle of the iris supply in front of the equator. The watershed zone between the anterior and posterior choroidal arteries lies in the equatorial region (Reproduced from Hayreh [95])
The occurrence of chorioretinal lesions due to occlusion of the long PCA in patients confirms the above findings in the rhesus monkeys (Figs. 6.13 and 6.14).
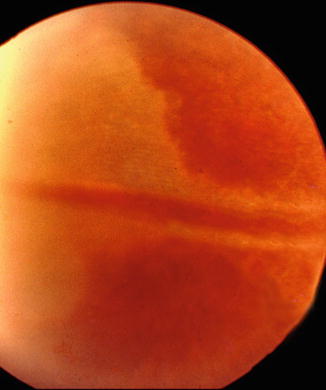
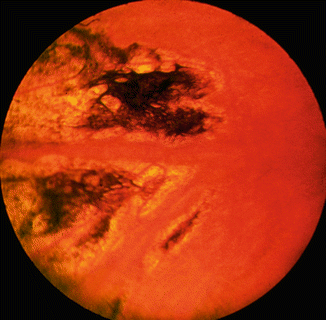
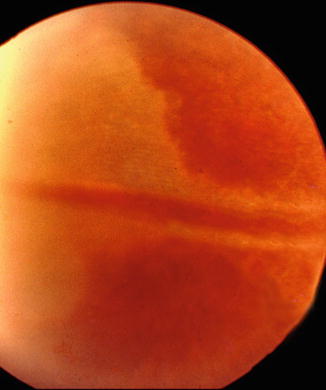
Fig. 6.13
Fundus photograph of the right eye showing triangular choroidal infarct in the temporal periphery due to occlusion of the long PCA (Reproduced by kind courtesy of Dr. Michael H. Goldbaum)
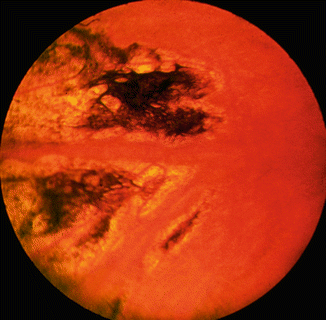
Fig. 6.14
Fundus photograph of the right eye showing triangular retinal pigment epithelial degeneration due to choroidal infarct in the temporal periphery caused by occlusion of the long PCA (Reproduced by kind courtesy of late Dr. P. Amalric)
The temporal long PCA is intimately related to the insertion of the inferior oblique muscle; the artery pierces the sclera at the nasal margin of the attachment of the tendon and the scleral canal of the artery lies along the line of attachment of the tendon. This fact may be important to bear in mind in surgical intervention on the inferior oblique muscle. It is not stressed adequately in the literature.
Arterial Blood Supply of the Anterior Segment of the Eye
Based on cast studies in monkeys and humans, the arterial supply of the anterior segment of the eye has been reported by several studies [49–52, 55, 92, 97–99]. Figures. 6.1, 6.2, and 6.15 are diagrammatic representation of its various aspects.
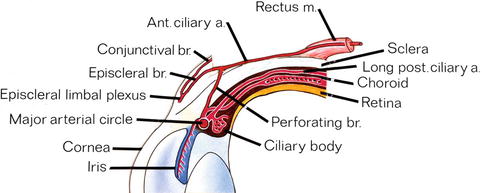
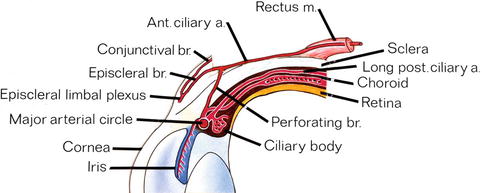
Fig. 6.15
Diagrammatic representation of arterial vascular supply of the anterior segment of the eye (Reproduced from Hayreh and Scott [100]). Ant. ciliary a anterior ciliary artery, br branch, long post. ciliary a. long posterior ciliary artery, m muscle
Arterial Circles in the Anterior Segment
The following three arterial circles in the anterior segment of the eye have been described. However, there is some controversy about different aspects of the three circles.
Episcleral Arterial Circle
This is formed by anastomoses between the adjacent ACAs (Fig. 6.2). Meyer and Watson [59], based on fluorescein angiographic studies in eight normal subjects, reported that this circle is formed by anastomoses between the adjacent ACAs and is a fragmentary circle. According to them, the anastomotic vessels may be superficial or deep; and the anterior episcleral arterial circle supplies the episclera, anterior conjunctiva, limbus, and iris. An incomplete arterial circle close to the canal of Schlemm is a part of this group (Fig. 6.1) [49].
Intramuscular Arterial Circle
It is formed by branches from the ACAs and long PCAs and is situated in the anterior part of the ciliary muscle toward the root of the iris (Fig. 6.2). It supplies the ciliary muscle and also sends recurrent branches posteriorly to the choroid. According to Funk and Rohen [55], in human eyes, this circle is formed by the perforating branches of the ACAs, and it lies in the posterior portion of the ciliary muscle.
Major Arterial Circle of the Iris
This lies at the root of the iris and in the vicinity of the ciliary processes (Fig. 6.2). It supplies the iris, ciliary muscle, and ciliary processes and also sends a few recurrent choroidal branches to the anterior part of the choroid [92]. Leber [1] wrote that the ACAs and long PCAs join at the root of the iris to form the major arterial circle. Shimizu and Ujiie [50], in their cast studies in rhesus monkeys, could not find any communication from the ACAs to the major arterial circle of the iris. According to Funk and Rohen [55], in human eyes, this circle is formed mainly by the long PCAs and supplies the inner and anterior portion of the ciliary muscle, the iris, and the ciliary processes. However, others [49, 51] have stated that perforating branches of the ACAs terminate in the anterior part of the ciliary body and anastomose with long PCAs to form the major arterial circle of the iris. According to Van Buskirk [51], this is the least continuous of the three arterial circles and consists of segments.
Blood Supply of the Sclera, Episclera, and Conjunctiva
These are supplied by the ACAs. Anastomoses between the adjacent ACAs form the episcleral vascular circle. Shimizu and Ujiie [50] studied the blood supply to this region in rhesus monkeys. They showed that the episcleral vascular plexus consists of at least three layers, each with different characteristics. (1) The deepest episcleral layer consists of a coarse, widely variable meshwork of small-sized venules and arterioles. (2) The middle episcleral layer comprises ACAs and their very few branches; anterior to the ACAs lie two to four tortuous vessels which frequently form vascular anastomoses. (3) The most superficial episcleral layer consists of a vascular network which occasionally communicates with the above vascular anastomoses. They felt that all these layers possibly represent the vascular system of Tenon’s capsule. The inner vascular layer of the palpebral conjunctiva is made up of a network of venules and capillaries. The arterioles are located in an outer layer. The venules run vertically toward the lid margin. The intermediate zone, along the lid margin between the palpebral conjunctiva and the skin, has venules converging toward the site of the external outlet of the Meibomian glands. Funk and Rohen [58], in a cast study of primates, found a capillary network in the equatorial region in the episclera, conjunctiva, and subconjunctival layer. In a zone 1–3 mm posterior to the limbus, they found a large number of short typical arteriovenous anastomoses fed by arterioles from the ACAs, but such arteriovenous anastomoses were rare in a zone posterior to the limbal region. The presence of arteriovenous anastomoses in primate casts was also reported by Selbach et al. [101], who also found capillary loops in the limbal arcade but not in the episclera itself.
Angiographic studies also have investigated the episcleral and conjunctival vasculature. Meyer and Watson [59], on fluorescein angiography of the conjunctiva and episclera in eight normal subjects, found that the episcleral arterial circle supplies the anterior conjunctival and episcleral circulations and the limbal arcades. According to them, the episcleral circulation is supplied by the episcleral arterial circle. Arteries of the episcleral circle give rise to fine loops that run forward into the limbal reflection of the conjunctiva before curving back radially to feed the lacework of the anterior conjunctival capillary plexus. Ormerod et al. [67], on 360° angiographic study of the episcleral and conjunctival vasculature in 11 normal human subjects, found an extensive episcleral venous plexus draining into a venous plexus around the rectus muscles, and small branches of the episcleral circle passing forward to the limbus where they looped backward in a radial direction to form the anterior conjunctival veins.
Blood Supply of the Limbus
The limbus is the region between the transparent cornea and opaque sclera. The blood supply to this part is entirely by the ACAs [49, 52]. Perforating branches of the ACAs, before penetrating the sclera, give branches to supply the limbus. Many arterioles from the limbal region supply the peripheral cornea. The peripheral conjunctiva is supplied by recurrent arterioles which loop posteriorly from the corneal arcade. Venous drainage from the peripheral cornea and conjunctiva, along with those from the episclera, drains posteriorly into the orbital venous system.
This region also contains a variable number of efferent vessels from the outer aspect of the canal of Schlemm, and they vary in size from capillary size to large trunks. Most of them, after leaving the canal, anastomose with one another to form the deep scleral plexus. The deep part of the intrascleral venous plexus of the anterior ciliary veins communicates with the canal of Schlemm through its efferent collector channels. Finally, they empty into the episcleral venous plexus.
Blood Supply of the Iris
It is well established that the blood supply of the iris comes from the major arterial circle of the iris. There are several cast studies dealing with the blood supply to the iris. Shimizu and Ujiie [50] in rhesus monkeys found the iris vessels arise from the greater arterial circle. The vessels in the iris have a radial course toward the pupil. Vessels along the collarette and the innermost pupillary border have a circular course and are few in number. The minor circle of the iris is not a complete circle. There are frequent interarterial communications in the iris. The venous blood from the iris flows through the ciliary process and finally into the vortex vein. Van Buskirk [51] in his cast studies in primates reported that iris arterioles usually arise from the major iris circle and occasionally from the intramuscular circle. The arterioles run centripetally toward the border of the pupil. The capillary network of the iris drains into iris venules, which join the veins that run toward the root of the iris and the bases of the ciliary processes and join the choroidal veins. Zhang and Gao [102], in casts of human subjects, found that the iris has about 180–200 radial vessels. The calibers of the iris arteries are homogeneous and measure about 50–120 μm. Song et al. [94], in flat preparation of the human iris, found that in the pupillary margin the iris vasculature network formed the minor arterial circle of the iris – an arcade, by connecting to adjacent vessels. In the pupillary margin, the capillaries were somewhat thick and connected to the irregular traveling iris vein.
Ojima and Matsuo [103] and Funk and Rohen [99], in their cast studies, reported that the iris has corkscrew-like arteries to allow iris movement. According to them, the iris vessels are arranged in the following three layers:
(i)
Anterior Capillary Layer: This consists of a large number of very dense, small, tortuous capillaries.
(ii)
Arterio–venular Layer: This lies within the iris stroma. It contains arteries and veins. The arteries have a bent spiral course, and the veins are straighter. Near the pupillary margin, there is an incomplete minor arterial circle of the iris. The veins run toward the root of the iris and drain into the tributaries of the vortex veins.
(iii)
Posterior Capillary Layer: This lies near the dilator muscle.
The iris capillaries are lined by an endothelial cell layer which has tight cell junctions, which results in the iris capillaries having a blood-aqueous barrier [104–106]. The iris capillaries also have pericytes.
The iris is a highly vascular structure. Rohen and Funk [107] postulated that that is meant to supply the surrounding avascular tissues of the posterior lamellae of the cornea and the trabecular meshwork with oxygen. Castenholz [108] postulated that this could be a thermoregulatory mechanism in this region. However, so far we have no proof of either assumption.
Parodi et al. [109] reported a rare congenital anomaly of iris arteriovenous communication on iris fluorescein angiography and indocyanine green videoangiography in eight patients. These iris arteriovenous communications consist of abnormal vascular connection bypassing the iris capillary bed.
Raviola and Butler [110] in rhesus monkeys found unidirectional movement of horseradish peroxidase in the iris blood vessels. That is, when it is injected into the blood stream, it remains in the lumen of the iris vessels. However, when it is injected into the anterior chamber, it permeates the iris tissues and penetrates the lumen of vessels of the iris by transcellular vesicular transport. Bill [111] reviewed the permeability of the blood-aqueous barrier to substances that are not transported by cellular mechanisms. The iris vessels seem to constitute a more efficient barrier in primates than in rabbits and cats, most probably due to the presence of tighter junctions between the endothelial cells in primates.
Normal Iris Vascular Pattern on Fluorescein Angiography
While cast studies give useful information about the iris vessels, they do not provide information about their in vivo circulatory pattern and properties. Fluorescein angiography of the iris vessels, however, does provide that essential information. I investigated the normal fluorescein iris angiographic vascular pattern in normal human eyes [112] and normal eyes of cynomolgus and rhesus monkeys [113].
Normal Human Iris Vascular Pattern on Fluorescein Angiography
In my human study [112], during the early stages, I tried fluorescein angiography in a number of patients with dark to light brown eyes, but the iris vessels of these subjects could not be outlined on angiography because of the brown pigment in the stroma masking the iris vessels. However, it has been shown that iris angiography using indocyanine green dye can show vessels in normal pigmented iris [114, 115]. On fluorescein iris angiography, the iris vessels could be outlined only in blue or green eyes, as has also been reported by other workers [65, 116–118]. In my study [112], fluorescein iris angiography was done in 42 blue or green eyes of subjects between 10 and 64 years.
The arteries in the iris started to fill radially from the root of the iris (Figs. 6.16, 6.17, 6.18, 6.19, 6.20, 6.21, and 6.22). The sequence of filling in the various segments of the iris was highly variable. Frequently, the entire iris started to fill simultaneously, but more often the various segments filled in different sequences; often the nasal part tended to fill first (Fig. 6.16) and the temporal part last. Delayed filling might involve the superior temporal, inferior temporal (Figs. 6.16 and 6.17), or temporal sectors only (Figs. 6.18 and 6.19). In that case, the upper and lower parts of the iris filled in the intermediate phase between the nasal and temporal filling, sometimes simultaneously and sometimes not. It was notable that, in a few eyes, the vessels in one small segment of the pupillary region (usually the temporal part) showed a substantial delay in filling, so that it was the last part of the iris to fill (Figs. 6.16, 6.17, 6.18, and 6.20). The filling of the entire iris usually took 5–10 s or even longer. In view of this marked diversity, no one filling pattern of the iris can be described as typical.
