Applied Laser Optics: Techniques For Retinal Laser Surgery
Nelson R. Sabates
Felix N. Sabates
Laser photocoagulation is the transfer of light energy into heat energy, which then denatures proteins and produces tissue coagulation. Laser radiation is directional and essentially coherent, allowing the beam to be focused to a very small spot of high irradiance. In addition, its monochromatic light allows the choice of different wavelengths to produce desired effects in specific tissue layers. These wavelengths extend from 400 nm, which is blue light, to 800 nm, which is the infrared wavelength.
Laser application can affect tissue according to different interaction modes: thermal, photochemical, and ionizing. These applications require high irradiance in a small area to produce their main effects. Nearly all of the laser procedures used in the treatment of retinal disease are thermal processes with the exception of photodynamic therapy (PDT), which is a photochemical process. (Fig. 1).
LASER INTERACTIONS
THERMAL EFFECT
Photocoagulation
The photocoagulation effect is the most commonly used in retinal laser surgery. Ocular photocoagulation uses heat produced through the absorption of light by ocular pigments. A rise in temperature of about 10°C to 20°C will cause coagulation of the ocular target tissue. The amount and rate of increase in temperature depend on the location of the target tissue as well as the degree of absorption of the specific wavelength that is used.
If the power is increased beyond the point of producing a minimal effect, the lesion size in the treated area will increase beyond the spot size. Associated with this is the important fact that it requires only a relatively small increase in temperature to change from a minimal tissue response to a heavy one. Obviously, it is necessary to make power increases in small increments in photocoagulation.
Laser energy applied to the retina has the highest absorption variance per unit in the pigment epithelium, owing to the high density of melanin granules in that tissue. In a small spot, the temperature rises in the pigment epithelium rapidly, reaching a constant value and equilibrium when the heat being introduced is equal to the heat being removed by conduction from the tissues. In larger spots, a higher temperature is obtained in the center of the burn, since heat removal from the center is less effective than from the edges. If the spot size is changed, an adjustment must be made in the power level. Decreasing a spot size requires a decrease in power level, whereas increasing a spot size requires a power increase.
Photocoagulation can be used to obliterate abnormal neovascular membranes, produce chorioretinal scars in retinal reattachment surgery, and treat single blood vessels. Photocoagulation is used in the treatment of many retinal diseases, including diabetic retinopathy, subretinal neovascularization, retinal vascular disease, sickle cell retinopathy, and peripheral retinal tears. It is also used in laser trabeculoplasty for the treatment of glaucoma. The most common laser used for these procedures is the argon green light source.
PHOTOCOAGULATION PARAMETERS
It is important to understand the concept of power density delivery of the coagulating beam applied to retinal tissue. Spot size, power settings, and exposure times are determined prior to treatment but can be, and often are, adjusted during the treatment. Different protocols have been established for different applications and interactions; however, these are only general guidelines. Flexibility is important and is based on training and experience with different settings and parameters.
The relationship between spot size, exposure time, and power, which are used to produce the correct power density, need to be closely monitored. If the spot size does not change, the power and exposure intervals should be kept constant until a good power density has been found. Any decrease or increase in spot size should be accompanied by a decrease or increase in input power until an appropriate response is obtained. Careful titration of the laser response is warranted. In practice, there are many factors that affect the size of the spot and power density, such as wavelength, media opacity, and absorption quality of the tissue to be treated.
Photovaporization
Photovaporization develops when laser energy increases the temperature of the cellular and extracellular water to 100°C, producing steam and vaporization of the tissue. Usually, a wavelength with low penetration is used, so most of the laser energy is absorbed superficially and creates tissue incision by tissue vaporization. After the tissue is vaporized, further application of laser energy results in even higher temperatures, producing photocarbonization of tissue.
The carbon dioxide laser has been used in ophthalmology primarily for photovaporization and photocautery. A great advantage of photovaporization is that it produces an almost bloodless incision by sealing blood vessels and lymphatic tissue in its path.1 Intravitreal photocautery has been used in treating fibrovascular fronds.2
Hyperthermia
Hyperthermia is the process whereby the laser causes an elevated tissue temperature above the normal 37°C but still avoids coagulation or vaporization effects. This temperature generally ranges between 42°C and 52°C. At these temperatures, biomolecules undergo changes that may result in significant membrane alteration. If this type of hyperthermia is maintained for a period of time (20 seconds to 3 to 4 minutes), irreversible effects occur.
Hyperthermia procedures on the retina are represented by transpupillary thermotherapy (TTT). TTT has been used in the treatment of choroidal melanoma and is being investigated as a treatment for occult choroidal neovascular membranes.3 Indocyanine green may be injected prior to treatment of a choroidal neovascular membrane to enhance specific absorption at the irradiation wavelength. The usefulness of these treatments is still under investigation.
IONIZING EFFECT
Extremely high irradiance delivered with a very short exposure in a small spot will result in electrons being energized from molecules in target tissue, producing a collection of free electrons and ions called plasma. Rapid expansion of the plasma creates acoustic and shock waves that, combined with latent tissue stress, incise the target tissue, producing photodisruption independent of tissue pigmentation or laser absorption. Transparent tissue can be incised in this way, obviating the need for conventional surgery. The most common laser source used is the Nd:YAG operating at the 1064-nm wavelength used in the anterior segment of the eye for discission of the posterior lens capsule, peripheral iridectomy, and synechotomy. In the posterior segment, it has been used successfully in the cutting of vitreous membranes and vitreoretinal bands. Because of the high amount of energy delivered at the focal point of disruption, it is important that the focus of energy be as far away from the retina as possible and at least 6 mm from the crystalline lens.4 Although there is intense heat at the point of plasma formation, the spot is so small that the heat dissipates rapidly.
PHOTOCHEMICAL EFFECT
A chemical reaction can be made to occur by intense laser energy if the energy of the photons is high enough. Energizing of photons increases with shorter wavelengths; therefore, ultraviolet light is more effective in producing photochemical reaction than is visible or infrared light. The photochemical effect of laser radiation has been used experimentally in photoradiation by red (630 nm) wavelength to produce cytotoxic free radicals in a tumor previously sensitized by a hematoporphyrin-derived uptake.5
The most common photochemical effect in use today is PDT, an example of laser treatment with a photosensitizing agent. This new treatment modality combines low-power diode lasers (689 nm) with an infusion of verteporfin to ablate subretinal neovascular membranes. The absorbed energy of the laser activates a series of photochemical events, beginning with the verteporfin and ending with the occlusion of vascular tissue in the subretinal membrane.6 The advantage of this therapy over conventional photocoagulation is that the lower energy levels result in much less damage to adjacent normal tissue.
Photoablation is produced by a photochemical reaction using an intense excimer (ultraviolet) laser. The most common ophthalmologic use of this therapy is in excimer lasers used in refractive surgery. At present, there are no uses for this therapy in the treatment of retinal diseases.
FACTORS INFLUENCING RETINAL PHOTOCOAGULATION
Optical characteristics of ocular tissues play an important role in the effectiveness of photocoagulation in the retina. Laser light traveling through the eye toward the target tissue is affected by the degree of reflection, scattering, and absorption of laser energy by ocular tissues and by media opacities. Other important factors affecting laser photocoagulation are the degree of absorption by ocular pigments, specific wavelengths used, spot size, power applied, and the exposure time used to produce the desired reaction in the retina (Fig. 2).
REFLECTION, SCATTERING, AND MEDIA ABSORPTION
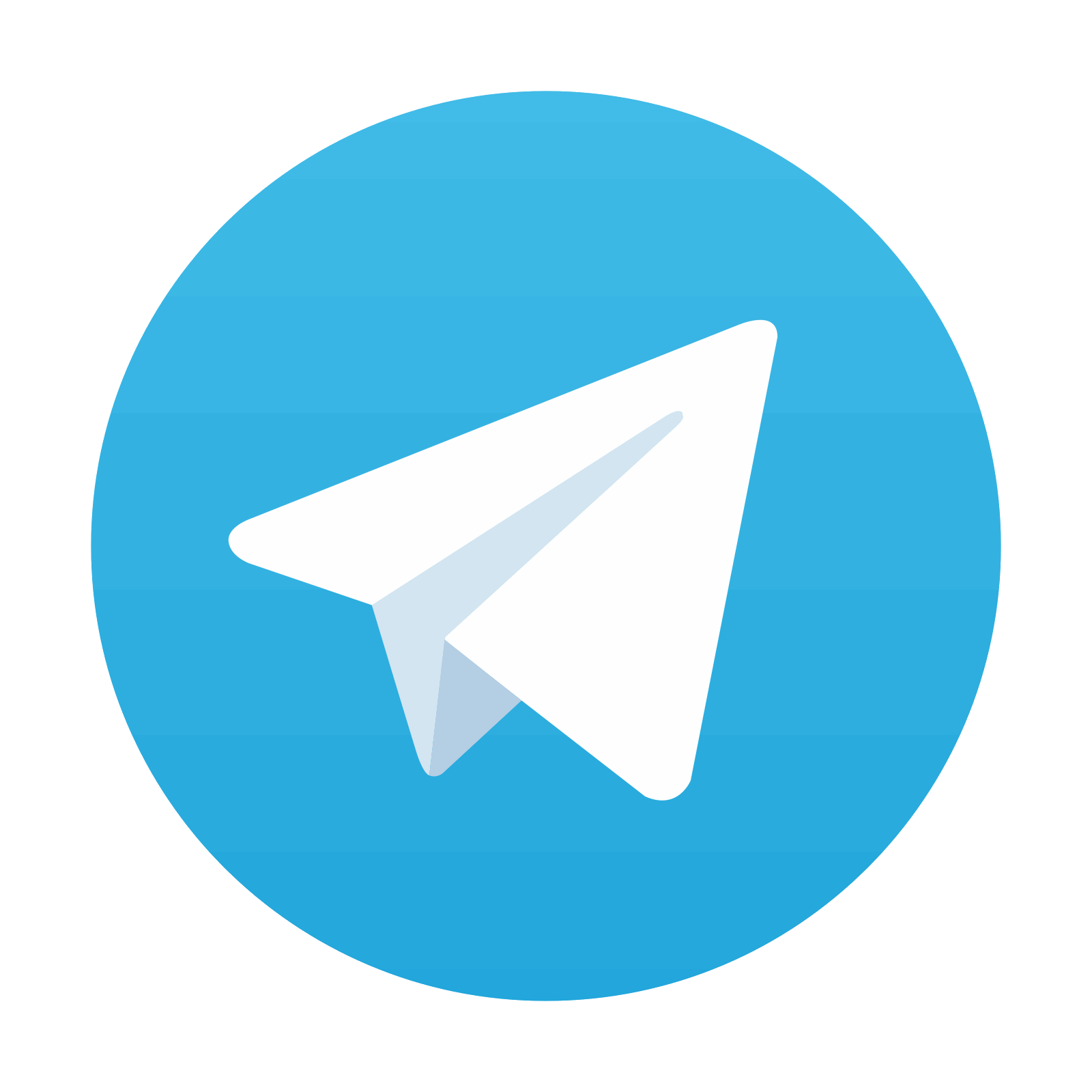
Stay updated, free articles. Join our Telegram channel

Full access? Get Clinical Tree
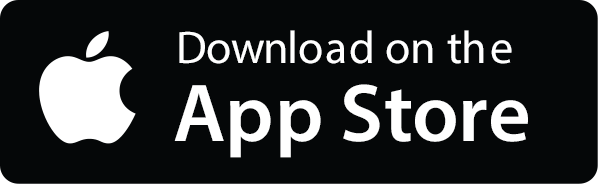
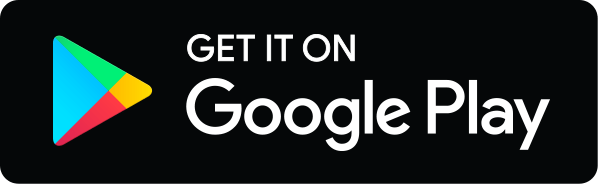