Antimicrobial Prophylaxis in Ophthalmology
Judy Ou MD1
Clinical Instructor
Bruce D. Gaynor MD2
Assistant Clinical Professor
Michael Starr MD3
Associate Clinical Professor
1 FI Proctor Foundation and Department of Ophthalmology, University of California at San Francisco, San Francisco, CA
2 FI Proctor Foundation and Department of Ophthalmology, University of California at San Francisco, San Francisco, CA
3 Department of Ophthalmology, Mount Sinai School of Medicine, New York, NY
Prophylaxis of ocular infections dates from at least the 19th century, with development of the Credé technique of silver nitrate instillation to prevent gonococcal ophthalmia in the newborn.1 Today, opportunities abound for ocular infection prevention, using antibiotics, antiviral agents, antiseptics, antitoxins, and immunization. The clinical settings include not only the prevention of postoperative infections but also posttraumatic intraocular and eye wall infections and tetanus; infections after removal of suture material and implantation of human donor tissues such as cornea, sclera, and fascia lata; and recurrent herpes simplex keratitis and cytomegalovirus (CMV) retinitis.
Rational prophylaxis of ocular infections is a challenging task because it requires consideration of the pharmacokinetics of drug delivery, optimal in vitro activity against the most likely offending pathogens, and demonstrated clinical efficacy in in vivo applications. It also encompasses more thorny aspects, such as the problems of promoting antibiotic resistance to potent antibiotics that are effective in treating the most antibiotic-resistant bacteria, and the ethics of preventing infection in an individual patient, possibly at the expense of the future welfare of the community of all patients. Indiscriminate and unthinking use of prophylaxis is potentially harmful to all and must be counteracted by well-founded and deliberate prophylaxis. This is all the more challenging in ophthalmology, in which there are fewer clinical studies to support and direct prophylactic regimens than in other medical and surgical specialties. This chapter discusses the scientific basis and specific recommendations for various prophylactic regimens in ophthalmology.
Prophylaxis of Postoperative Infections
Nonophthalmic Literature
Perhaps the two most important factors responsible for the success of modern surgery are the use of aseptic technique and prophylactic antibiotics. The effectiveness of antibiotics during surgery was first established in an experimental animal model in the early 1960s.2 Many clinical trials have proved the effectiveness of prophylaxis; perioperative antibiotic use has become a standard of care for most major surgical procedures. Despite this, there are at least 920,000 postoperative wound infections in the 23 million patients who undergo surgery annually in the United States.3 The exact timing, choice of antibiotic, and duration of therapy are constantly being updated. Although there are no definitive studies in the ophthalmic literature, the nonophthalmic surgical experience provides a framework for the development of a treatment regimen for ocular surgery. The use of antibiotics in contaminated or emergent settings, such as perforated bowel or infected biliary or urinary tracts, is considered to be therapeutic and therefore is not included in this discussion of prophylaxis.
The indications for prophylaxis include procedures wherein the risk of infection is high or the consequences of infection are particularly deleterious, even if the risk of infection is low. For about two decades, it has been firmly established that prophylaxis is beneficial in “clean-contaminated” procedures such as bowel surgery, in “clean” procedures involving the insertion of a prosthetic device such as total hip replacement, and in major “clean” procedures such as coronary bypass.5,6,7 More recently, indications for prophylaxis have extended to include relatively minor “clean” procedures such as mastectomy or herniorrhaphy.8
The success of antibiotic prophylaxis in surgery correlates directly with the susceptibility of the infecting organism in vitro to the antibiotic used.9 Because of their excellent spectrum of activity, the low incidence of allergy, and proved efficacy in clinical trials, the cephalosporins, especially cefazolin, are used most widely in the world for prophylaxis.10 Emerging pathogens with greater resistance may require rethinking of this approach in the future.
Historically, one of the most controversial questions regarding prophylaxis is the timing and duration of therapy. It is generally thought that high blood levels of antibiotic should be maintained throughout the surgical procedure and that a long course is no more beneficial than a short course in the postoperative period.11,12,13 In a study of 2,847 patients undergoing elective clean or clean-contaminated surgical procedures, it was found that preoperative (during the 2 hours before incision) antibiotic administration was responsible for a significant reduction in wound infection, compared with early (2 to 24 hours before incision); perioperative (during the 3 hours after the incision); or postoperative (between 3 and 24 hours after the incision) administration.14 In this study, there were 346 different types of surgery performed; the three most common were total abdominal hysterectomy (10%), cholecystectomy with intraoperative cholangiography (9%), and bowel resection (8%). Four intravenous antibiotics constituted 84% of all the antibiotics used: cefazolin (56%), cefonicid (12%), cefoxitin (10%), and cefamandole (6%).
Emergence of Resistant Organisms
Although enterobacteria collected before the advent of modern antimicrobial agents had conjugative plasmids at about the same frequency as those isolated today, they rarely contained antibiotic-resistance genes, whereas plasmids isolated today often encode resistance to antibiotics.15 Bacteria are continuously developing resistance to antimicrobial agents; there are more than 100 described antimicrobe-resistance genes.16 Acquired resistance occurs by several mechanisms: alteration of the drug target, production of a detoxifying enzyme, decreased antibiotic uptake, or multidrug-resistance pumps.17 Important examples of resistance include penicillin-resistant Streptococcus pneumoniae,18 vancomycin-resistant enterococci (VRE),19 the presence of extended-spectrum β-lactamases (ESBL) in several species of Gram-negative bacilli,20 and imipenem-resistant Pseudomonas aeruginosa.21 Also, multidrug-resistant Staphylococcus aureus remains a continued threat.22,23
An important example of antibiotic resistance by target alteration is penicillin-resistant Streptococcus pneumoniae. Penicillin-binding proteins (PBPs) are enzymes important in creating and maintaining the peptidoglycan component of the bacterial cell wall. Penicillins bind these enzymes and thereby interfere with cell wall synthesis. Highly resistant pneumococcal strains have PBPs with markedly decreased affinity for penicillin. Although this phenomenon was first described in 1967, widespread presence of these isolates did not occur until the 1980s.18 Roughly 25% of U.S. isolates are penicillin resistant.24
Another example of target alteration is seen in vancomycin-resistant enterococci. Vancomycin, a glycopeptide, inhibits cell wall synthesis by forming complexes with the peptidyl-D-alanyl-D-alanine termini of peptidoglycan precursors at the cell surface. The presence of these complexes hinders prolongation of the growing peptidoglycan chain and blocks formation of interpeptide bonds. Resistant enterococci synthesize peptidoglycan precursors with the depsipeptide D-alanyl-D-lactate, which has decreased affinity for vancomycin. This resistance mechanism was first documented in France in 1988 and presents a significant clinical problem.19
Production of β-lactamases represents the second mechanism of acquired bacterial resistance, production of a detoxifying enzyme. β-Lactamases are a heterogeneous group of enzymes that hydrolyze the cyclic amide bond of the β-lactam-ring antibiotics. In the early 1980s, a new group of enzymes arose, designated the extended-spectrum β-lactamases, conferring resistance to third-generation cephalosporins in strains of Klebsiella pneumoniae, P. aeruginosa, and Escherichia coli.20 Surveys of nosocomial isolates in Europe show a rising prevalence of these organisms, and multiple outbreaks in the United States have been documented.25
Imipenem-resistant P. aeruginosa represents the third mechanism of resistance, decreased uptake of antibiotic. These bacteria lack an outer membrane protein, D2, through which imipenem normally traverses. The prevalence of these strains is also rising.21 Multidrug-resistance pumps are the fourth mechanism of bacterial resistance. These efflux pumps are thought to be widespread in bacteria. especially in E. coli and Pseudomonas species and function by antibiotic extrusion.26,27,28,29
Some organisms display multiple types of resistance mechanisms. The development of penicillin-, methicillin-, and fluoroquinolone-resistance in S. aureus is a classic example. It is notable how quickly resistance developed after introduction of these antibiotics. In the early 1940s, penicillin G was almost universally effective against S. aureus. By the mid-1940s, the rapid appearance of β-lactamase provided S. aureus resistance to penicillin G; by the 1990s, more than 95% of S. aureus was resistant to penicillin G, ampicillin, and the anti-Pseudomonas penicillins.22 The emergence of methicillin-resistant S. aureus (MRSA) in the 1980s owing to altered PBPs spurred an increase in the use of vancomycin. Widespread use of vancomycin may have created the selective pressure favoring vancomycin resistance in other bacterial species (e.g., enterococci). In 1996, the first documented case of S. aureus with intermediate levels of resistance to vancomycin (VISA) was reported from Japan (30), and isolates have been reported from the United States.31,32 Four years after the introduction of fluoroquinolones, most nosocomial MRSA was resistant to fluoroquinolones, and such resistance has been reported in almost all countries.23 Fluoroquinolone resistance is mediated by the alteration of DNA gyrase.33 Most multidrug-resistant infections are nosocomial, but these resistant organisms inevitably will spread to the community.
Although resistance genes were rarely present before the use of antimicrobial agents,34 the use or overuse of these agents provided the requisite selective pressures for greater expression of these genes.35 There is evidence that subinhibitory levels of antibiotics (e.g., during tapering) provide a condition highly favorable for the selection of resistant organisms.36 Current practices by physicians include the increasing use of wider-spectrum antimicrobial agents to treat minor infections.37 Such practices must be resisted and discouraged to avoid the catastrophic potential of ever-widening antimicrobial resistance.21,38 In Finland, a decrease in the use of macrolide antibiotics has led to a decrease in the frequency of erythromycin resistance in group A streptococci.39 Widespread changes to more conservative prescription patterns would certainly slow the emergence of resistant strains but may also decrease the frequency of resistant strains that have already appeared.40
Pharmacokinetics
General Considerations
The pharmacokinetics of antimicrobial delivery to the ocular anterior and posterior segments is fundamental to devising rational strategies to treat and prevent intraocular and surface infections. Antibiotic selection, dosing, timing, and route of administration are influenced by pharmacokinetic considerations. For example, the anatomic and physiologic barriers to delivery of antimicrobials to the posterior segment has usually necessitated direct intravitreal antimicrobial instillation to deliver adequate amounts of medication. As another important example, the pharmacokinetics of antimicrobial elimination from the eye are especially significant with single or infrequent dose administration regimens, such as anterior chamber or intravitreal antibiotic injections, or infusion fluid irrigation with antibiotics, where rapid elimination may rapidly reduce antimicrobials to less than inhibitory concentrations. Subinhibitory concentrations are not only ineffective but may also play a role in the development of drug-resistant bacteria, a factor that impacts on adequate dosing of all prophylactic regimens, including topical drop administration. Pharmacokinetic considerations are also important in the interpretation of in vitro antimicrobial sensitivity testing results. For both prevention and treatment plans with antimicrobials, pharmacokinetic factors are critical.
Sensitivity Testing
In vitro sensitivity testing may be performed on almost any ocular microbial isolate, usually in instances of conjunctivitis, keratitis, and endophthalmitis; however, the results of sensitivity testing reported simply as “resistant” or “sensitive” to a given antibiotic are predicated on the serum levels of the antibiotic that are achievable with systemic administration, rather than the levels achievable in ocular tissues by local administration. The in vitro data, unrefined by local pharmacokinetic considerations, may have limited clinical relevance. The commonly achievable serum levels after systemic administration may be lower or higher than those achieved in various intraocular or surface ocular tissues with nonsystemic routes of administration, such as topical drops and collagen shield soaked delivery systems, subconjunctival injections, and direct installation routes into the anterior chamber or vitreous. One can better employ quantitative in vitro sensitivity parameters, expressed as minimal inhibitory or cidal concentrations of antimicrobial (usually expressed as micrograms of antibiotic per mL of fluid), if one also knows the usual range of antimicrobial tissue concentrations achievable in the relevant ocular tissue (expressed in the same units, or as μg/mg of tissue) by the more commonly used local routes. One might thereby make a reasonable determination whether the isolate will receive effective concentrations of antimicrobial in ocular tissue and will be likely to respond clinically to a degree that the in vitro data would suggest. Such consideration of the clinically achievable antimicrobial tissue concentration is reflected by the inhibitory quotient (IQ), a ratio of the achievable peak antibiotic level in a given tissue to the in vitro minimal inhibitory concentration (MIC) for a given antibiotic-bacterial combination.41 This measure provides a multiple or fraction of the in vitro antimicrobial level necessary to inhibit the growth of 50% (mic50) or 90% (mic90) of strains of the organism tested. An inhibitory quotient of 5 to 10 or more is generally accepted as indicative of adequate antimicrobial tissue levels and has been correlated with better clinical response to both ocular and nonocular infections.42,43 Beyond pharmacokinetic factors and in vitro sensitivity testing, in vivo antibiotic effects are related to the “time and concentration dependence” pharmacodynamic indices of a given antimicrobial.44 The efficacy of some antimicrobials is dependent on exposure time to the pathogen when the antimicrobial is above a minimally effective in vitro concentration (T>mic), but with less significant correlation to antimicrobial efficacy with increases of concentration beyond this minimum (usually two to four times the MIC). Time-dependent antibiotics, such as vancomycin, the cephalosporins, and the macrolides (e.g., erythromycin), exert less than their full antimicrobial potential with shortened exposure times. When such antibiotics are introduced into the anterior chamber after intraocular surgery and are rapidly cleared with aqueous turnover, they may have insufficient time to exert a significant antimicrobial effect. Concentration-dependent antibiotics such as the fluoroquinolones and aminoglycosides, are more dependent for their efficacy on the peak concentration achieved in tissue than on the duration of exposure to the pathogen and demonstrate a more direct correlation between peak concentration and antibacterial effect.45 Plotting antimicrobial concentration in tissue versus time produces a curve that reflects the duration of minimal concentrations necessary for clinical efficacy (T>mic), as well as peak concentrations (Cmax), relevant to both types of dependency classes.46,47,48 Of critical importance, one must consider that in vitro sensitivity testing, even with allowance made for achievable ocular tissue levels and the duration of those levels, does not factor in the in vivo variables that make in vitro testing and pharmacokinetic considerations incomplete in predicting actual clinical response. Drug interactions with infectious and inflammatory exudates, tissue and serum binding of antimicrobials, and normal function of host defenses against infection are only a few factors that may impact on the ultimate microbial response to prophylaxis or treatment that is not reflected by in vitro sensitivity testing results and pharmacokinetic considerations.
Specific Antibiotics
Fluoroquinolones
The fluoroquinolones have become the predominant antibiotic class for prophylactic use in ophthalmology since their introduction as ophthalmic drops in the early 1990s. Their increasingly excellent in vitro spectrum of coverage and pharmacokinetic profile and low ocular toxicity have lead to their predominance over previously favored classes, such as the aminoglycosides.49 The pharmacokinetic profile of these agents is sufficient to provide therapeutic aqueous humor levels after topical instillation to a degree that, for the first time, provides a sound empirical basis for their use as prophylactic preoperative and perhaps postoperative topical agents, despite the long-standing use of the postoperative prophylaxis route with antimicrobial agents that in past decades possessed less impressive intraocular penetration.50 Fourth-generation agents demonstrate impressive ocular pharmacokinetics, and their administration by systemic routes have started to overcome the barriers to achieving effective intravitreal concentrations of antimicrobials that are confronted with previous antibiotic types. This has lead to some rethinking about potential routes of antimicrobial administration for the treatment of endophthalmitis, beyond intravitreal injection, with potential impact as well on prophylactic regimens.51,52 The second-generation fluoroquinolones, norfloxacin, ciprofloxacin, and ofloxacin, were generally effective against most ocular aerobic bacteria with some deficiencies covering streptococcal species as well as anaerobes. At the time second-generation fluoroquinolones were introduced, methicillin-resistant staphylococci were not as prevalent and problematic as at present, and the lack of efficacy against these organisms was not as significant. Today, multiply resistant staphylococcal species are increasingly prevalent in community-acquired infections and represent a significant threat for ocular infection that is still not adequately addressed even by the newer fourth-generation fluoroquinolones.53,54,55,56
In most other respects, the fourth-generation fluoroquinolones have been shown more effective in vitro than second-generation fluoroquinolones and earlier classes of antibiotics, such as the aminoglycosides, against ocular bacterial isolates, and in general they achieve higher intraocular penetration, although the significance of these differences is untested or lacking in some clinical settings.57,58 The fourth-generation fluoroquinolones target two bacterial enzymes, a basis for claims of their inherent superiority over earlier fluoroquinolones, which target only one enzyme, enabling less development of bacterial resistance by requiring two instead of one genetic mutation. Such resistance to fourth-generation fluoroquinolones will need to be closely monitored since thus far, the race between pharmacologic research and development on the one hand, and bacterial adaptation to newer antibiotics on the other, has been running for over five decades without a clear winner.
Aminoglycosides
Topical aminoglycosides, such as gentamicin and tobramycin, less so neomycin, have been used extensively in ophthalmology for many years, particularly prior to the advent of the fluoroquinolones. A broad spectrum of both Gram-positive and -negative coverage and good cidal activity in the presence of adequate concentration, however, was offset by local tissue toxicity.59 Surface epitheliopathy and retinotoxicity were of concern, especially the latter with gentamicin.60,61,62,63 Although currently superseded by the fluoroquinolones in many clinical scenarios, the development of significant numbers of fluoroquinolone- and methicillin- resistant staphylococcal infections, and their current frequent in vitro susceptibility to aminoglycosides such as gentamicin, suggest that the aminoglycosides may continue to have a useful role, such as in posttraumatic endophthalmitis prophylaxis and the treatment of fluoroquinolone-resistant postoperative endophthalmitis.64 For most surface infections, including keratitis, aminoglycosides have had adequate pharmacokinetics to treat susceptible organisms. Concentrations many times in excess of the usual commercial preparations of these antibiotics have been used to enhance tissue penetration, especially in treating Pseudomonas species ulcerative keratitis. Aminoglycosides often serve as general Gram-negative coverage in conjunction with a concentrated formulation of topical Gram-positive coverage, currently usually vancomycin, as part of a broad-spectrum coverage for serious ocular surface infections of unspecified but presumed to be bacterial origin. A study in rabbit and human eyes65 showed that with sufficiently frequent dosage, topically applied 0.3% gentamicin can achieve bacteriostatic or even bactericidal levels in the aqueous and other ocular tissues, and penetration is greater in an inflamed eye.66 Another study showed the levels attained intravitreally after topical administration in rabbit eyes to be variable (between 0 and 2.9 μg/mL).67 There is evidence that gentamicin penetration into ocular tissues is enhanced by aphakia but intravitreal levels remain less than inhibitory at best (and often unmeasurably low) (68).68 Tobramycin has been used topically in ophthalmology for more than 20 years; there is in vitro and clinical evidence that it is more effective and is associated with fewer adverse reactions than gentamicin.69,70,71 When 0.3% tobramycin was applied topically four times daily for 2 days, however, and at 90 and 60 minutes before cataract surgery, clinically significant aqueous humor levels (13 ng/mL) were unobtainable at the start of surgery.72 Tobramycin levels in tear film are proportional to the concentration of antibiotic instilled, but as with gentamicin, at commercially available, as well as at higher “fortified” concentrations, significant decreases in rates of re-epithelialization are seen compared to nonaminoglycoside antibiotics.73
Other Antibiotics
Bacitracin is a polypeptide antibiotic produced by Bacillus species and is often used topically in ophthalmology. Its ocular penetration is poor but is enhanced when a corneal epithelial defect is present.63 Despite increasing resistance among endophthalmitis isolates to commonly used antibiotics, many remain susceptible to bacitracin, making it a useful choice in the reduction of ocular surface bacterial isolates in a prophylactic setting, notwithstanding its poor ocular penetration (74). Trimethoprim has potentially wide antibacterial coverage, except for Pseudomonas species, and therefore is often combined with the antipseudomonal agent, polymyxin B, with which it may be synergistic.75,76 When used for systemic infections, trimethoprim is susceptible to the rapid development of bacterial resistance and may be paired with a sulfonamide to mitigate this effect. Although topically applied trimethoprim/polymyxin B sulfate fails to reach significant aqueous humor levels, there is evidence that it is clinically efficacious in nonserious surface ocular infections.72,77,78,79,80
Administration Routes
Topical Drops
Topical antibiotic drop administration has the longest track record for ophthalmic use and is recommended for its simplicity and low cost as well as high safety profile. This is countered by the inconvenience and sometimes uncertain compliance of patient self-administration. Depending on the pharmacokinetics of the specific antibiotic, antibacterial effect may be exclusively extraocular and serve to decrease only the bacterial population of the ocular surface, or may have significant intraocular penetration that impacts on aqueous or vitreous microbial contamination. Contrary to oft-cited references to sterilization of the ocular surface, the eradication of ocular surface flora for any significant period is not practicable, even with the concomitant use of disinfectants,81 possibly due to sequestration of flora in conjunctival crypts where antimicrobial action is minimized. Pharmacokinetic considerations are much simpler when considering surface action than when preoperative or postoperative administration is intended to achieve some level of intraocular penetration, but dosing issues remain important in either instance. Consideration needs to be paid to the potential encouragement of drug resistant organisms when subinhibitory levels of antibiotic are present owing to insufficient dosing, for either prophylactic use or treatment of established infection.82,83 Prophylactic short-term use has been shown in one study to not encourage emergence of resistant flora when an accepted dosing regimen is used.84 Long-term topical use has been shown to induce resistance at extraocular sites.85 Care should be exercised in this regard by limiting the duration of prophylactic regimens as well as avoiding infrequent dosing. With intraocular instillation of antibiotics, encouraging the development of resistance has been considered less of an issue by some authors, but as noted later, the duration of adequate intraocular antimicrobial levels still raises the issue of efficacy for this route of prophylaxis.86
Collagen Shields
Collagen shields have been advocated as a drug delivery device for topical antimicrobial administration because of their ability to rapidly provide sustained drug levels without the inconvenience of frequent drops or the potential side effects or complications of subconjunctival injections.70 Studies on various antibiotics, including tobramycin, gentamicin, vancomycin, ofloxacin, gatifloxacin, moxifloxacin, and amphotericin B, have been done on rabbit and human eyes.87,88,89,90,91,92,93 Data from these studies show that generally, when presoaked with antimicrobial agents, collagen shields are able to attain anterior segment antibiotic levels comparable to or greater than those with topical drops or subconjunctival injection, but the kinetics of drug delivery vary with the antibiotic in question and in some instances may be less than levels achievable with topical drops.94 This variability, without consistent results for all antimicrobial preparations, along with toxicity issues relating to concentration of the antimicrobial, must be considered when shields are used for clinical applications. In one clinical series, application of gentamicin and dexamethasone by subconjunctival injection and by presoaked collagen shield was compared in patients undergoing extracapsular cataract extraction.95 No adverse effects were reported and the collagen-shield patients had less postoperative pain, less conjunctival injection, less conjunctival hemorrhage, fewer Descemet folds, and less aqueous flare than the subconjunctival injection patients. In another series of patients undergoing cataract surgery, ofloxacin-soaked collagen shields provided considerably higher aqueous humor antibiotic levels than after topical administration.96 Another potential advantage of drug delivery using collagen shields is that the shield may be used to deliver two or more medications simultaneously. Various drug combinations, such as cefazolin and tobramycin, and gentamicin and methylprednisolone, can form precipitates under certain conditions, however.97 The effect of these precipitated medications on bioavailability to ocular tissues and the potential mechanical and biochemical toxicity to ocular tissue are for the most part unknown. More clinical data are needed for antimicrobial delivery by collagen shields, especially in humans, before recommendations regarding their use for infection prophylaxis can be made.
Subconjunctival Injections
Subconjunctival injections of antibiotics are used routinely by many surgeons for prophylaxis. Studies with various antibiotics given subconjunctivally show postinjection differences in the regional concentration of the drug, consistent with penetration of these drugs by direct diffusion through the underlying ocular coats at the injection site, rather than by leaking through a conjunctival perforation site and then penetrating diffusely through the cornea via the tear film.98,99,100 Animal and human studies show adequate penetration of subconjunctival gentamicin into anterior segment tissues but poor penetration into the vitreous.101,102,103 Aqueous levels of tobramycin delivered by subconjunctival injection have been shown to be therapeutic.104,105,106 Subconjunctivally administered cefazolin also reaches therapeutic concentrations in aqueous but as with most antibiotics given by subconjunctival injection, vitreous penetration is poor.107,108 Third-generation cephalosporins (ceftizoxime, ceftriaxone, and ceftazidime) show poor penetration into the vitreous of rabbit eyes when given 100 mg subconjunctivally; an inflamed eye facilitates drug penetration.109 Subconjunctivally injected cefotaxime reaches therapeutic levels in human vitreous only in eyes that have had previous surgery.110 A randomized trial showed that patients given subconjunctival cefuroxime had less pain and hyperemia than those given gentamicin,111 and use of this second-generation cephalosporin for prophylaxis by subconjunctival injection has been popular in some European countries (see later). Subconjunctivally injected cefuroxime attains clinically significant aqueous levels, whereas topically applied drops do not.112 There are few data regarding the subconjunctival injection kinetics of fluoroquinolones. Ciprofloxacin (1 mg) injected perilimbally in rabbits reached aqueous levels of 0.887 μg/mL and 0.094 μg/mL at 1 and 10 hours, respectively. Data on fourth-generation fluoroquinolone penetration by subconjunctival injection are not available at present.
Intraocular Administration
Intracameral administration of antimicrobials, either by direct instillation or by inclusion in intraocular irrigation fluids, has been advocated for the prophylaxis of postoperative endophthalmitis, based predominantly on anecdotal reports and uncontrolled studies of their efficacy.114,115 Although this route would seem to be advantageous by overcoming barriers to penetration with topical and subconjunctival routes, pharmacokinetic considerations of antimicrobial elimination with aqueous humor turnover make questionable the likely efficacy of this route for prophylaxis.116,117 Several antibiotics commonly used by this route, such as vancomycin and the aminoglycosides, have been shown to have a short half-life in the anterior chamber so that the duration of supra-inhibitory levels may be too short to be effective against intraocular contamination at the time of intraocular surgery.118,119 Current studies on fourth-generation fluoroquinolones are preliminary but might show more promising results owing in large part to their generally low MICs and non–time dependency. Their anterior chamber levels, however, are unlikely to remain sufficiently prolonged to prevent intraocular infection that could occur through incompetent incisions a day or more after surgery.120 If shown to be safe, a combination of intraoperative intracameral and postoperative topical fourth-generation fluoroquinolones might provide an effective regimen to provide sufficiently prolonged postoperative prophylaxis, even for microbial contamination of the intraocular compartments associated with incompetence of the surgical incision. As has been previously noted, all such pharmacologic prophylaxis should be adjunctive to meticulous aseptic surgical technique, including careful incision architecture and, when indicated, use of sutures to minimize the risk of incision incompetence.121
Systemic Administration
In general, use of systemic antimicrobials for prophylaxis of postoperative infection has long been discouraged, in part because of pharmacokinetic considerations and in part because of their low risk/benefit ratio. Penetration of antibiotics into the vitreous cavity is impeded by the blood–retina barrier, although there is evidence that disruption of this barrier, such as during inflammatory conditions or trauma, facilitates penetration.66,122,123,124,125,126,127,128 Intravenous aminoglycosides have thus been advocated for trauma-associated endophthalmitis prophylaxis despite evidence of poor vitreous penetration.67,129,130 Because of increasing cephalosporin resistance to coagulase-negative staphylococci, some authors have advocated empirical intravitreal and in some cases, intravenous, vancomycin for the treatment of established endophthalmitis.131,132,133,134 Ciprofloxacin has been advocated as an adjunct in endophthalmitis prophylaxis because of its broad spectrum of activity and good intravitreal penetration when given orally or intravenously.135,136,137 El-Baba et al135 showed human intravitreal ciprofloxacin levels above the minimal inhibitory concentration for 90% of strains for Staphylococcus epidermidis, Bacillus species, and Enterobacteriaceae after a single oral dose of 750 mg; additional oral doses show even higher intravitreal levels.138 Similar therapeutic intravitreal levels were obtained in rabbits and pigs after intravenous ciprofloxacin administration.126 Three doses of 400 mg of oral ofloxacin also attained clinically significant intravitreal levels.139 The Endophthalmitis Vitrectomy Study140 showed no visual benefit to intravenous ceftazidime and amikacin in treating established postoperative (after cataract surgery or secondary lens implantation) bacterial endophthalmitis; based on this, the study concluded that intravenous antibiotics have no place in the treatment of postoperative endophthalmitis. This may be too broad an extrapolation of the results however, for all clinical applications and antibiotics. Vitreous penetration of both intravenous cephalosporins and aminoglycosides (e.g., ceftazidime and amikacin) is known to be generally poor and should not be extrapolated to the use of other antibiotic classes (e.g., fluoroquinolones or vancomycin) in this setting. Furthermore, prophylaxis against infection in a traumatized eye may be very different than in a postoperative eye because of issues of ocular pharmacokinetics; finally, the requirements for successfully preventing intraocular infection may be significantly less demanding than those for treating established intraocular infection, so that parenteral antibiotics for prophylactic indications might be more reasonable from an efficacy standpoint.141 As noted previously, the fourth-generation fluoroquinolones show the most impressive intravitreal penetration after systemic administration with some basis for reconsideration of the systemic route for prophylaxis. The potential, however, for significant adverse effects of systemically administered antibiotics, to prevent a rarely occurring infection in the millions of patients who undergo elective intraocular surgery annually, may continue to argue strongly against routine antimicrobial prophylaxis by this route.
Sources of Infection and the Role of Preoperative Culture Testing
Sources of infection in postoperative endophthalmitis include both endogenous (patients’ normal resident flora on lids and conjunctiva) and exogenous (airborne contaminants, contaminated intraocular solutions, intraocular lenses, surgical instruments and equipment, and operating room personnel) sources. There are essentially four types of evidence that establish preoperative, endogenous normal surface flora as the most common source of infectious organisms in postoperative endophthalmitis: similarity regarding species incidence (e.g., S. epidermidis is the most common isolate in both ocular surface normal flora and intravitreal cultures of postoperative endophthalmitis)142,143,144,145,146; phage-typing identities of isolates from routine preoperative surface cultures and postoperative endophthalmitis vitreous specimens147,148,149; similar or identical antibiotic sensitivity profiles; and molecular epidemiology profiles using DNA probes, restriction fragment length polymorphism, and pulsed-field gel electrophoresis.150,151 The preponderance of evidence implicating endogenous flora as causative of endophthalmitis led some decades ago to preoperative culturing and attempts to reduce or eliminate ocular flora with prophylactic antibiotics and antiseptics. Obtaining reliably reproducible culture results has proved elusive, however. For example, daily conjunctival and lid margin cultures in asymptomatic adolescents were highly variable in one study, and in another study conjunctival cultures taken preoperatively in asymptomatic patients were reproducible 1 day later in only 65% of 65 patients not using antibiotics.152,153 Therefore, the reliability and significance of cultures in asymptomatic patients remains unclear, and culture results may be misleading in any given patient. Even if culture results were consistently reproducible, however, their usefulness would still remain doubtful. More than 95% of preoperative normal cataract patients harbor organisms that are capable of causing infection, whereas the incidence of postoperative endophthalmitis remains much lower, by a factor of 1,000 or less.154 Routine preoperative cultures are therefore an inefficient way to predict and prevent postoperative endophthalmitis and are no longer routinely performed in asymptomatic patients. Cultures in patients who demonstrate signs of ocular surface infection, however, are essential in reducing infection risk, and steps should be taken to attempt eradication of any ocular surface or lacrimal drainage system infection before performing elective surgery. Patients with any extraocular infections probably should also be treated before proceeding with elective ocular surgery because of possible cross-contamination to the ocular surgical site or because of potential host defense impairment resulting from fighting an ongoing extraocular infection.155 Finally, the clinical usefulness of standard in vitro sensitivity testing on ocular isolates is unproved and where possible, should be evaluated in the context of the pharmacokinetic considerations noted previously.
Antibiotics: Effects on Normal Flora and Endophthalmitis Incidence
Determining the impact of antibiotics on postoperative endophthalmitis incidence has always been a challenge, in part because of the difficulty controlling for the many factors that may influence the development of infection, and in part because of the fortunate infrequency of these infections. To control for a multitude of potential confounding variables, an adequately sized prospective clinical trial is best, with random assignment of patients and appropriate masking. Often a retrospective case control study is undertaken with considerably less complexity and expense, and commensurately less compelling results. In both study designs, the many thousands of surgical patients necessary to result in sufficient infections in the treatment arms, however, generally requires long periods of time and/or multiple surgical centers, both of which complicate the control of all variables. Adding to this the development of new antimicrobials, which may make dated, or obsolete, the results of formidable and expensive clinical trials, one can readily appreciate the many issues that complicate any attempt to assess the impact of antimicrobial prophylaxis for ophthalmic surgery. The results of such trials, especially those dating from the middle decades of the last century, are open to question because of methodological shortcomings. The clinical trials of more recent years, although sometimes more methodologically rigorous, have proven too expensive to be conducted often and still are wanting in convincingly demonstrating any unequivocal therapeutic effect.156 Some might argue that this only reflects the lack of effective antibiotic prophylaxis, but the beneficial effects of antibiotics on surrogate variables like cultures of the ocular surface flora and the aqueous humor, as well as the more voluminous and convincing data on the value of antibiotic prophylaxis in nonophthalmologic surgery, collectively suggest the likelihood of prophylactic efficacy in ophthalmic surgery and support the wide use of antimicrobials for prophylaxis of infection in this clinical setting.
Although the consistency of results of external eye cultures is variable, as noted previously, there is evidence that the use of preoperative topical antibiotics decreases the amount of lid and conjunctival flora in asymptomatic patients as well as postoperative bacterial counts in patients wearing patches.157,158,159 As discussed later, this has also been demonstrated for antibiotics administered by other routes. Fahmy160 showed that aminoglycoside drops given the night before surgery were effective in eliminating 95% of pretreatment strains of bacteria by the next morning. In this study, gentamicin sulfate solution 0.3% was more effective than several other antibiotics including chloramphenicol 0.5%, sulphamethizole 4%, bacitracin 1%-neomycin 0.5%, and ristocetin 0.5%-polymyxin B 0.25% solutions, and oxytetracycline 3%-polymyxin B 0.1% ointment. Whitney et al161 demonstrated a significant reduction in the incidence of Staphylococcus species on the eyelids preoperatively with the use of antibiotics, especially gentamicin 1.0% solution. (Note that this gentamicin concentration was more than three times that of the currently commercially available concentration.) Gentamicin was again superior, in this study, to chloramphenicol 0.5% solution, polymyxin B-neomycin-gramicidin solution, and polymyxin B-neomycin-bacitracin ointment. Six doses given hourly of topical ciprofloxacin 0.3% drops significantly lowered counts of coagulase-negative staphylococci specifically, and total bacteria overall, preoperatively in normal eyes.162 Osher et al72 showed that one drop of either trimethoprim 0.1%/polymyxin B sulfate 10,000 U/mL, or tobramycin 0.3% given 4 times daily for 2 days and 90 minutes and 60 minutes before cataract extraction, eradicated all organisms identified in baseline conjunctival cultures except S. epidermidis. Similar results have been observed with other topical antibiotics, including the fourth-generation fluoroquinolones.163,164
Studies that demonstrated the ability to decrease preoperative ocular surface flora encouraged many surgeons to apply antibiotics topically preoperatively and postoperatively for endophthalmitis prophylaxis, and in subsequent years by other routes as well. The data supporting the actual benefit of antibiotic usage for decreasing postoperative ophthalmic infections, however, are somewhat limited and imperfect, especially in comparison to the body of clinical research on antibiotic prophylaxis for general surgery. Dunnington and Locatcher-Khorazo (144) performed an early retrospective analysis of postoperative endophthalmitis in cataract surgery and found no cases of endophthalmitis in 663 patients treated with either topical penicillin or sodium sulfathiazole ointments preoperatively. A nonconcurrent historical control group treated with silver-protein solution had an endophthalmitis incidence of 1.8%. Another early study described 7,095 cataract patients who received various topical antibiotics preoperatively.145 An incidence of endophthalmitis of 0.08% in treated patients was thought to be an improvement over historical controls. In a nonrandomized trial spanning 21 years, patients given preoperative topical antibiotics for cataract surgery had a lower incidence of endophthalmitis than patients not given antibiotics.164,165 Since these earlier studies, newer and more powerful antibiotics, specifically the fluoroquinolones, have enjoyed increasing popularity for prophylactic topical use in ophthalmology. Their safety is accepted, as is their pharmacokinetic and in vitro efficacy, but clinical evidence of their in vivo ability to effectively reduce the incidence of postoperative endophthalmitis is limited to date.166,167,168,169
Furthermore, as with the earlier generations of fluoroquinolones, the increasing prevalence of resistance among the bacteria that commonly cause postoperative endophthalmitis threatens the future utility of the fourth-generation class of these antimicrobials170 and there continues a debate as to what extent the therapeutic and prophylactic use of these antimicrobials in ophthalmology contributes to the development of resistant organisms, in any given patient, as well as in the population at large.
The effect of subconjunctival antibiotics on endophthalmitis has been studied in large groups of patients over many years, yet there continues a debate on their efficacy with widespread geographic variation in the frequency of using this route of prophylaxis.171,172,173 A study of 54,000 patients in the Indian subcontinent showed no decrease in endophthalmitis in those patients given antibiotics subconjunctivally, compared with those who were not.174 A subsequent study on 23,900 patients showed a reduction in endophthalmitis when antibiotics were given subconjunctivally and topically, compared with topically only.175 In a more recent retrospective case control study, subconjunctival cefuroxime, a second-generation cephalosporin, demonstrated a statistically significant reduction in the incidence of presumed postoperative endophthalmitis176, confirming the results of an earlier smaller case-control study using the same antibiotic.177 A protective effect against infection was also found in a retrospective study using subconjunctival gentamicin, or gentamicin and cefazolin, a first-generation cephalosporin.178 Other studies have confirmed a beneficial effect of subconjunctivally administered antibiotics179 despite earlier studies that failed to demonstrate a benefit, perhaps because of methodologic issues.180 A recent literature review that preceded the more recent studies failed to find convincing evidence for the benefit of subconjunctival antibiotic injections, however.156 Because of geographic variations in antimicrobial susceptibilities as well as changes over time as bacteria spread resistance mechanisms, favorable results must be taken with some circumspection as advocating for specific antimicrobial choices. For example, increased resistance to aminoglycosides has been noted among Staphylococcus isolates causing postoperative endophthalmitis in recent years.133 In addition to questions of efficacy, there are significant potential complications of administering antibiotics subconjunctivally, including inadvertent needle penetration of the globe or entrance of leaking subconjunctival antibiotic through unsutured corneal or scleral incisions, potentially causing intraocular inflammation or retinal toxicity. Indeed, episodes of sterile endophthalmitis have been suspected secondary to various different causes including toxic levels of antibiotics entering the eye by direct intracameral instillation at the end of surgery or through unsutured incisions after postoperative subconjunctival injection.181
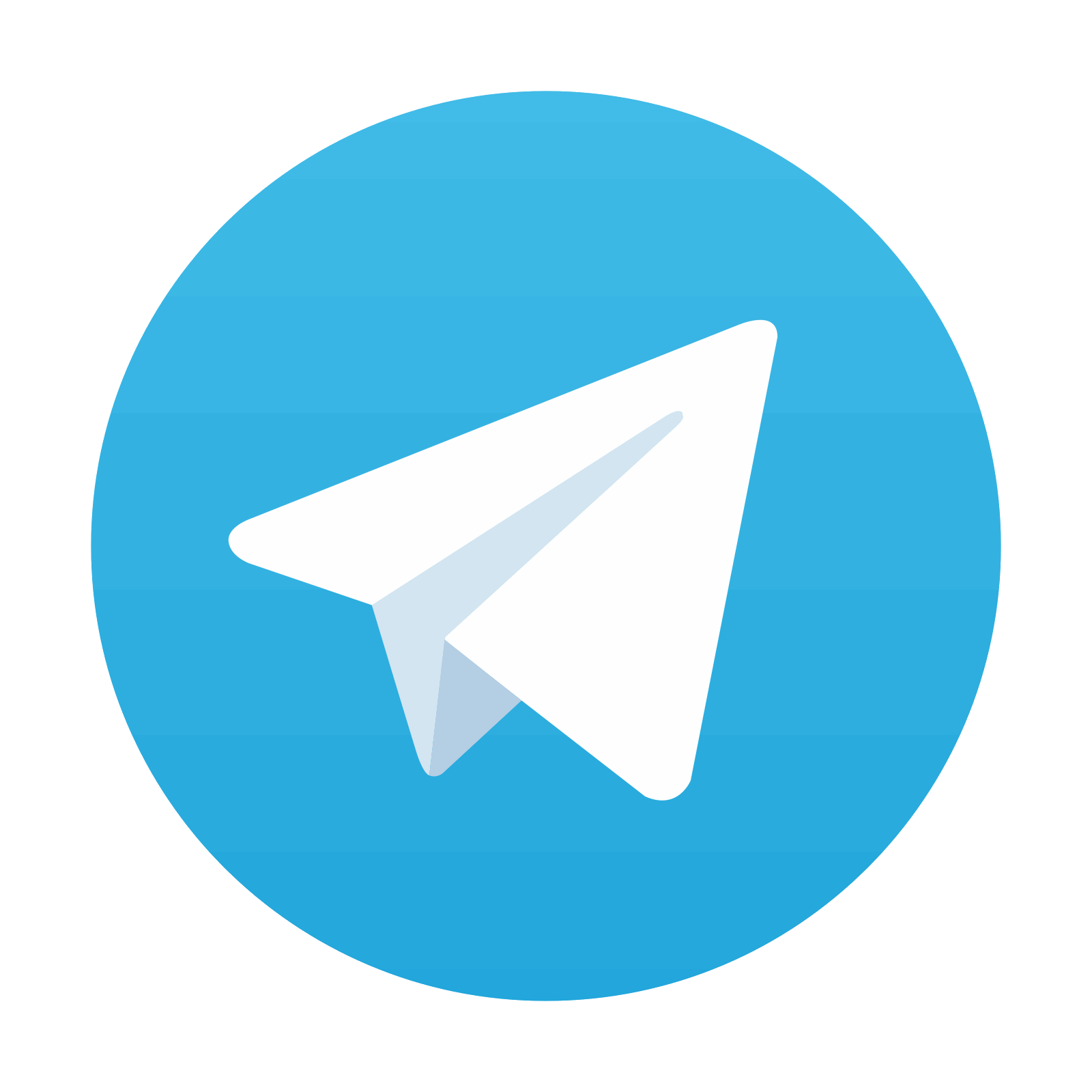
Stay updated, free articles. Join our Telegram channel

Full access? Get Clinical Tree
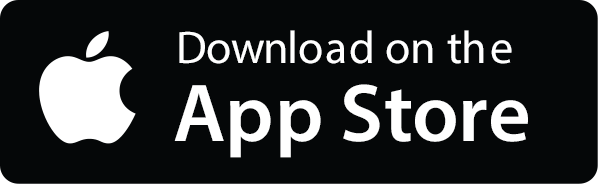
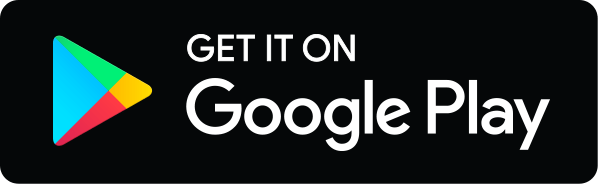