Anatomy and Embryology of the Optic Nerve
Cristiano Oliveira
Christopher A. Girkin
Russell W. Read
Supported in part by unrestricted departmental grants from Research to Prevent Blindness, Inc., New York, NY, USA, and the Eyesight Foundation of Alabama, Birmingham, AL, USA. Drs. Girkin and Read are both recipients of Physician-Scientist Awards from Research to Prevent Blindness, New York, NY, USA.
Anatomical Overview
The second cranial, or optic, nerve is an evagination of the central nervous system and is unique among the cranial nerves in this aspect. The optic nerve is composed of the myelinated axons of the retinal ganglion cells (RGC) and is interspersed with connective tissue septae. The number of axons within the human optic nerve exhibits individual variability with counts ranging from approximately 800,000 to 1.5 million.1 Similarly, the glial and myelin components are present in variable amounts, relative to the axons and the surrounding microenvironment.
Measured from the posterior sclera to the optic chiasm, the human optic nerve is approximately 50 mm long and can be divided into four portions: intraocular; intraorbital; canalicular; and intracranial. The intraocular segment can be further subdivided into three regions: the surface nerve fiber layer; prelaminar; and laminar regions. The intraocular segment is approximately 1 to 1.5 mm long. The intraorbital portion, extending from the posterior sclera to the optic canal, allows considerable excursion as the globe moves due to tortuosity along its 40 mm length (Fig. 1). The intracanalicular portion, approximately 6 mm long, passes through the optic canal along with the ophthalmic artery and branches of the sympathetic plexus.2
Within the intraorbital and intracanalicular portions, the optic nerve is surrounded by extensions of the arachnoid and dural sheaths, which corresponds to the intracranial structures. These represent a potential pathway for the spreading of blood, infectious agents, and tumor cells between the eye and the central nervous system. At the posterior globe, the dural sheath fuses with the sclera and the sheaths of the superior and medial rectus muscles. This latter anatomical fact explains the pain with eye movement frequently found in retrobulbar optic neuritis. Posteriorly, the dura is fused to the periosteum of the optic canal. This results in relative immobility at this segment of the nerve and makes it more susceptible to injury. The pia mater that envelops the nerve fuses to its outer surface and covers the central retinal vessels.2
The intracranial prechiasmatic optic nerve is approximately 10 mm long. The flat and X-shaped chiasm may lie within the chiasmatic sulcus or more posteriorly near the diaphragm sellae (prefixed or postfixed chiasm, respectively). This variability in the position of the chiasm accounts for some of the variation in visual field defects found with pituitary tumors. The optic tracts terminate in the lateral geniculate nuclei.2
Several important structures are situated in close proximity to the intracranial portion of the optic nerve. The frontal lobes of the brain lie above the nerves; the anterior cerebral and anterior communicating arteries separate the optic nerve from the olfactory tract; the lateral aspect of the optic nerve is adjacent to the internal carotid artery as the vessel emerges from the cavernous sinus; and the posterior ethmoid and sphenoid sinuses are adjacent to the nerves posteriorly.2 These close anatomic relationships form the basis for the visual manifestations of space-occupying lesions occurring in these areas.
Nerve Fiber Layer and Optic Nerve Head
The axons of the RGC are the major component of the optic nerve, with astrocytes as its major glial cell type. At each level of the optic nerve head, glial cells are organized to support the axons in their passage from the eye to the optic nerve. Immunostaining techniques have been used to characterize astrocytes. All express glial fibrillary acidic protein (GFAP) (Fig. 2) and can be divided into type 1 and type 2 astrocytes based on the absence or presence, respectively, of the neural cell marker A2B5. Type 1 astrocytes are further subdivided into type 1A and type 1B according to the expression of neural cell adhesion molecule (N-CAM), which is present only in type 1B astrocytes.3,4 The nonmyelinated portion of the RGC axons initially extend from their somal origin, travel along the vitreal surface of the neural retina, following a centripetal route toward the neural canal, forming the nerve fiber layer of the retina. The axons are organized into bundles by astrocytes, which is a process that begins at the point of axon emergence from the RGC cell body. These bundles are the basis of the striations seen clinically in the retinal nerve fiber layer. Type 1A cells (GFAP+/N-CAM–) provide direct structural support for the axons, whereas type 1B cells (GFAP+/N-CAM+) provide a physiological interface between axons and vitreous, connective tissue and vascular tissue.3 After reaching the optic disc, the axons take a sharp turn to pass though the neural canal, which is encased by the supportive connective tissue of the lamina cribrosa. The optic nerve head extends from the vitreal surface of the optic disc to the posterior scleral surface (Fig. 3).
The prelaminar portion of the optic nerve head contains very little collagenous connective tissue, other than that surrounding the central retinal vessels and capillaries of the disc.
The lamina cribrosa consists of a reticular network of connective tissue beams that serves to support the nerve. These beams generally have a vascular core surrounded by an extracellular matrix and covered by glial tissue.5 The RGC axons pass through pores formed by spaces between the beams. The lamina functions to protect the axons from biomechanical stresses as they pass from the relatively higher pressure intraocular space to the lower pressure space of the central nervous system. Elastic fibers are a major component of the extracellular matrix of the lamina cribrosa.6 and provide it with elasticity and resiliency to adapt to mechanical distortions. Laminar type 1B astrocytes line the cribriform plates, extend their processes inside those plates and the nerve bundles,3 and are responsible for the synthesis of ECM macromolecules, such as elastin and collagen.7,8,9 Type 1A cells’ processes are associated with the axonal bundles passing through the laminar openings.3 Beginning in the postlaminar region, the axons become myelinated (Fig. 3) and type 2 astrocytes (GFAP+/N-CAM–/A2B5+) are first seen within the bundles.3
Retrobulbar Optic Nerve Cell Types
Axons
After the axons cross the lamina cribrosa they become sheathed by myelin produced by oligodendrocytes (Fig. 3). Hematoxylin- and eosin-stained cross sections of the optic nerve demonstrate that the principal myelinated optic nerve fibers appear as small, faintly stained, eosinophilic dots surrounded by relatively clear halos. These clear halos, representing the myelin sheaths, are the result of lipid dissolution during processing. Special stains can be used to enhance the appearance of axons (e.g., Bodian’s method and Luxol fast blue staining). Using electron microscopy, the nerve fibers are identified as cytoplasm with mitochondria, enveloped by multilaminar myelin sheaths formed by oligodendrocytes (Fig. 4).
![]() FIGURE 4. Ultrastructure of the optic nerve. A. Axons are surrounded by myelinated sheath. B. Oligodendritic cell. (M) Microglial cell. 18,750×. (Courtesy of Dr. John R. Guy.) |
There is a certain degree of topographic representation within the optic nerve head corresponding to the retinal surface. RGC axons originating in the peripheral retina are located in the peripheral portion of the nerve, whereas those originating more posteriorly enter the optic nerve head closer to the optic cup. Fibers from the macula form the papillomacular bundle and enter the disc temporally.10 At the level of the chiasm, nerve fibers originating in the nasal retina decussate, whereas temporal axons project ipsilaterally. The relative proportion of uncrossed fibers determines the size of the binocular visual field and varies among different species.11 The axons project to the lateral geniculate nucleus, the superior colliculus, and several other subcortical nuclei in a highly ordered manner, maintaining the spatial relationship originating in the retina12,13 and reflecting their embryologic development (discussed later).14,15,16,17,18,19
The unmyelinated prelaminar and laminar regions of the optic nerve demonstrate high mitochondrial enzyme activity when compared with the postlaminar myelinated regions. The high levels seen in unmyelinated regions of the human optic nerve reflect the dynamic functional requirements of this part of the nerve. Myelinated fibers conduct by saltatory conduction, whereas unmyelinated fibers must repolarize more extensive areas of plasma membrane, which requires more energy. Given that the unmyelinated optic nerve has a relatively higher demand for mitochondrial enzyme activity, this region may be more sensitive to mitochondrial deficits, which may explain why optic neuropathies can occur in those with mitochondrially inherited diseases.20
Glial Elements
As in the central nervous system, the glial elements of the optic nerve are astrocytes, oligodendrocytes, microglia, and newly described NG2-glia. These cells interact in a dynamic fashion with axons by establishing functional interdependency. The physiology and pathology of these cell types are integrated by extracellular signals including glutamate, potassium (K+), and ATP.21,22
Astrocytes
Astrocytes exhibit large cell bodies with many long, coarse processes. With electron microscopy, cytoplasmic glycogen granules, extensively lobulated nuclei, numerous intermediate glial filaments, and a wide type of granular endoplasmic reticulum characterize these cells. Processes extending from astrocytes—not seen with light microscopy—are seen as numerous thin structures almost devoid of cytoplasmic organelles, but are densely packed with microfibrils. They form end-feet over the basal lamina of both the pia mater and vasculature to create the glia limitans and extend minute collaterals that engage the nodes of Ranvier, which are the only areas of myelinated axolemma not covered by myelin.22
Glutamate is the primary axon-to-glial signaling molecule and is released either from axons at the level of the nodes of Ranvier or from astrocytes in response to activation by axonal action potential propagation at the nodes.23,24 A single node of Ranvier is contacted by the perinodal microprocesses of different astrocytes. Activation of the glutamate receptors raises astrocyte intracellular calcium (Ca2+), and because these cells are functionally coupled via gap junctions, Ca2+ waves are propagated through the syncytium following initial stimulation.25,26
ATP is the primary signaling molecule between astrocytes in the optic nerve,27 and it further couples axonal activity and astrocyte functions.22 Astroglial Ca2+ waves not only signal between astrocytes, but also modulate the behavior of oligodendrocytes and microglia and potentially induce reactive changes distant from the site of central nervous system damage.28,29
Astrocytes primarily regulate extracellular K+ released by the axons at the nodes of Ranvier,30 but also form a conduit from blood vessels to axons, through which glucose can be rapidly transported. Astrocytes also store glycogen, the single largest energy reserve in the central nervous system, that can be broken down and transferred to axons.31
After any type of insult to the central nervous system, astrocytes become reactive and exhibit a vigorous response specific to the region or to the nature of the insult involved.32 Reactive astrocytes have a characteristic phenotype and actively synthesize a variety of molecules in response to stress.33 They adopt some properties of immature cells, such as motility and production of elastin.9 Expression of elastin mRNA is not detectable in the normal adult optic nerve,34 but reappears in pathological situations, such as glaucomatous optic neuropathy.35 In the lamina cribrosa, synthesis of elastin by astrocytes most likely represents a specialization of these cells to the mechanical function of the tissue, and when resumed, represents a specific response to mechanical stress.36 Elastosis may alter laminar tissue compliance and may contribute to the progression of damage seen in glaucoma.36
Oligodendrocytes
Oligodendrocytes are normally absent in the retina and optic nerve head, first appearing posterior to the lamina cribrosa. The primary function of these glial cells is to provide the myelin sheath to RGC axons, which facilitate the rapid conduction of nerve impulses. Mature myelin-producing oligodendrocytes are process-bearing cells with round or oval nuclei and granular cytoplasm. Each oligodendrocyte terminal process sheathes an axon to form one internodal myelin segment of variable thickness and length.39 Oligodendrocytes are located in groups between axons, usually near the center of the axonal bundles, with their processes running parallel to the axons.
When viewed by electron microscopy, oligodendrocytes appear moderately electron dense compared with astrocytes. Their nucleus is round or oval and is usually eccentrically located, which leaves a large mass of cytoplasm at one pole of the cell. The nuclear content exhibits a slight clumping of chromatin that appears as a rim beneath the nuclear envelope (Fig. 4). The cytoplasm is rich in ribosomes that are either free or associated with the endoplasmic reticulum. Their Golgi complexes are well developed. Oligodendrocytes have neither glycogen granules nor bundles of intermediate filaments. When analyzed by immunohistochemistry, oligodendrocytes do not stain for GFAP, but are positive for galactocerebroside, myelin basic protein, and N-CAM.3
It is now clear that, in addition to insulating axons, oligodendrocytes are important for axonal integrity and nodal function. Oligodendrocytes induce segregation and clustering of sodium (Na+) and K+ channels along RGC axons and at the developing nodes of Ranvier.40,41,42 Oligodendrocyte disruption and demyelination result in the dispersion of axolemmal ion channels and impairment of conduction.43
Similar to astrocytes, differentiated oligodendrocytes express glutamate receptors that raise intracellular Ca2+ when activated.44 Glutamate activity is normally terminated by uptake into oligodendrocytes, astrocytes, and axons,45 but under pathological conditions, such as ischemia, membrane transporters operate in reverse and the enhanced glutamate signaling becomes excitotoxic.46,47
Oligodendrocytes are exceptionally sensitive to any insults to the neural tissue, such as injury, ischemia, or inflammation. This results in the loss of oligodendrocytes and myelin and eventually secondary axon degeneration.48 Demyelination is marked by the attenuation of and, in extreme cases, complete loss of internodal myelin sheaths in individual oligodendrocytes and manifests as slowed nerve conduction velocities and conduction block.49
Microglia
Microglia are resident phagocytic mononuclear cells adapted to the specialized microenvironment of the neural tissue, performing the function of immune surveillance. In normal tissue, microglia are quiescent with a stellate shape (due to multiple ramified processes), a small nucleus, and are distributed in a regularly spaced array throughout the optic nerve.50,51 Viewed with electron microscopy, microglial cells have small, heterochromatic nuclei (Fig. 4). Their cytoplasm contains vacuoles, a granular endoplasmic reticulum arranged in long narrow strands and various inclusions, such as large dense bodies, lamellar bodies, myelin bodies, and other forms of cellular debris. Consistent with their immune origin and similar to hematogenous monocytes, microglia express cells markers, such as HLA-DR (major histocompatibility class II), CD45 (leukocyte common antigen),51 and CD68 (Fig. 5).
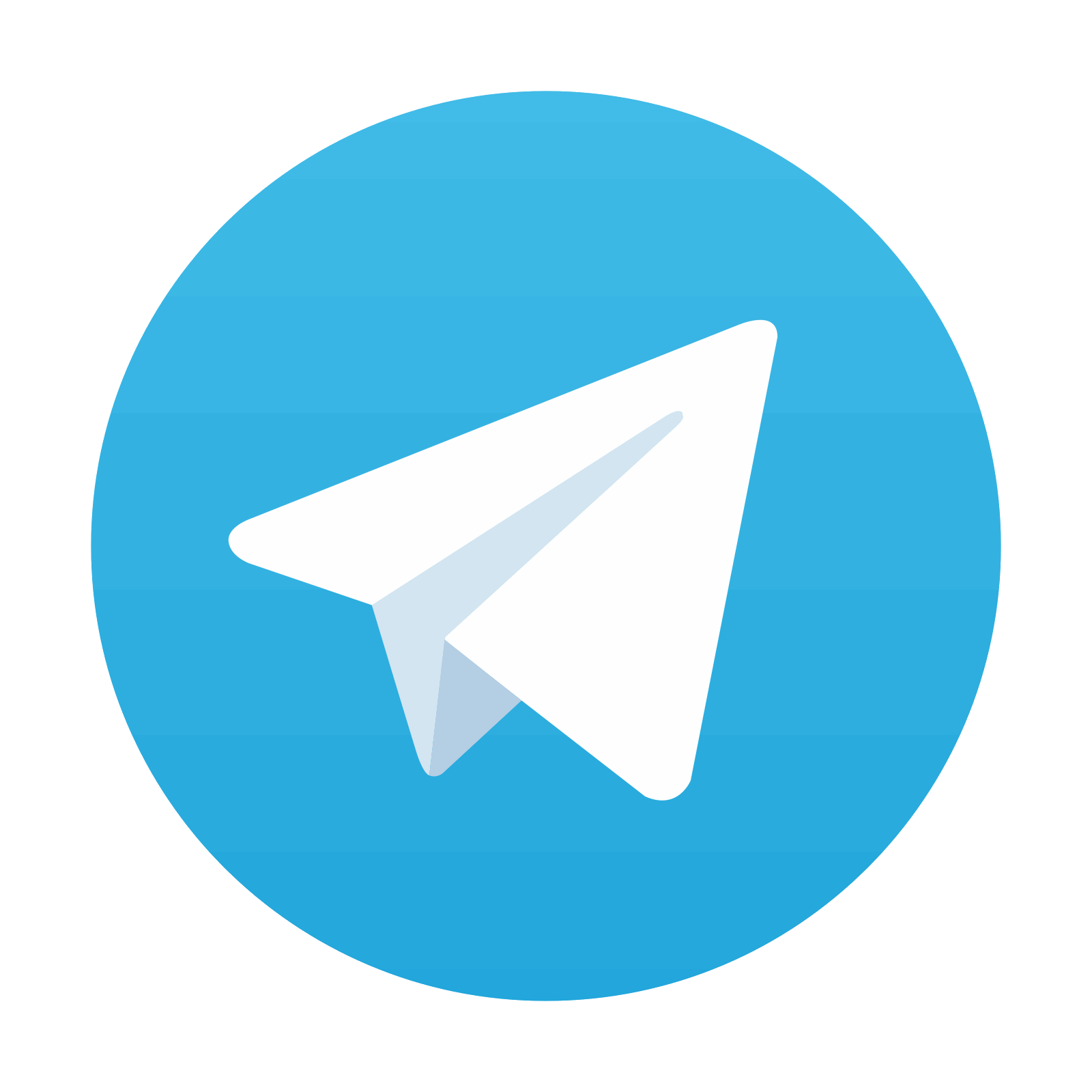
Stay updated, free articles. Join our Telegram channel

Full access? Get Clinical Tree
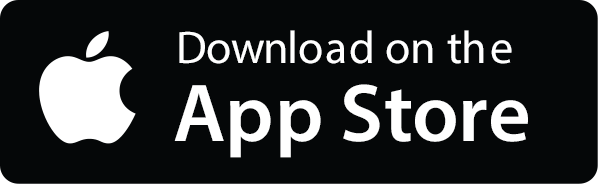
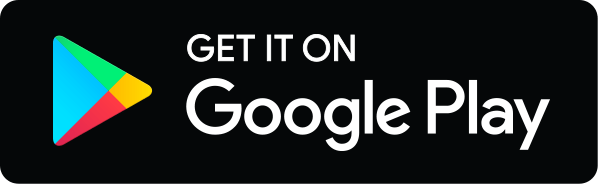
