Anatomy and Cell Biology of the Human Retina
Ann H. Milam
Julie E. Smith
Sinoj K. John
The term retina derives from the Latin word rete, meaning “net.” This name was originally used by ancient Greeks, who observed that the retina had the appearance of a fishing net.1 The retina has two functions. The first, performed by the rod and cone photoreceptors, is to transduce information from an optical image into electrical signals. The second, performed by the neural circuits of the retina, is to process certain features of the visual world from the photoreceptor signals and relay this information to the brain via the optic nerve.2 Microscopic features of the retina have been studied since the late 1600s, when Antony Van Leeuwenhoek described globular cells and blood vessels in a bovine retina.1 By the late 1800s, Ramón y Cajal and Camillo Golgi had developed silver staining techniques and elucidated the basic cellular organization of the retina. In his 1892 monograph, La Rétine des Vertébrés, Cajal2 wrote:
Furthermore it is recognized that the retina is a genuine neural center, a sort of peripheral cerebral segment whose thinness, transparency and other qualities render it particularly favorable to histological analysis. In fact, although its cells and fibers are essentially similar to those of other centers, they are arranged in a more regular fashion, different types of cells being distributed in distinctly separate layers. Furthermore, the limited size of their dendritic fields, the orientation of the axon (always well defined and descending) and the presence of layers arranged expressly for intercellular connections (outer and inner plexiform layers) are fortunate circumstances which help to clarify the morphology and interrelations of neurons.
Later investigators, notably Stephen Polyak in the 1930s, used the classical Golgi methods to provide detailed information about the various cells in the primate retina, including that of the human.1 Since that time, application of newer techniques, including electron microscopy, radioautography, immunocytochemistry, and molecular biology, has expanded our understanding of human retinal organization. However, many questions about the anatomy and cell biology of the human retina, some outlined below, remain to be answered by application of modern research methods.
DEVELOPMENT OF THE RETINA
The retina comprises tissues derived from the optic vesicle, an outgrowth from the diencephalon at approximately 25 days in fetal development (Fig. 1). The optic vesicle invaginates during the fourth fetal week to form a cup, the inner wall of which differentiates into the multilayered neurosensory retina (Fig. 2). The outer wall of the cup becomes the pigment epithelium.
RETINAL PIGMENT EPITHELIUM
The retinal pigment epithelium (RPE) is a monolayer of hexagonal cells extending from the margin of the optic disc to the ora serrata, where it is continuous with the pigmented epithelium of the pars plana of the ciliary body.3 The RPE cells are cuboidal in cross section and joined near their apical margins by junctional complexes that include maculae and zonulae adherentes (intermediate spot and belt junctions, respectively), as well as zonulae occludentes (tight belt junctions). In the zonulae occludentes between adjacent RPE cells, the plasma membranes are fused and are impermeable to water and ions (Fig. 3). These continuous belts of tight junctions constitute the outer blood—retinal barrier, which blocks extracellular movement of water, ions, and macromolecules between the choriocapillaris and the subretinal space.
The RPE cells are essential for photoreceptor health and have several other functions, including vitamin A metabolism and regeneration in the visual cycle,4 phagocytosis and degradation of shed outer segment tips, light absorption by melaningranules, heat exchange, secretion of the matrix surrounding the photoreceptor inner and outer segments, and active transport of materials between the choriocapillaris and the subretinal space.
The RPE cells are smaller (approximately 10 to 14 μm diameter) and contain more melanin in the macula, and are flatter and wider (up to 60 μmdiameter) with less pigment in the periphery. Photoreceptor density also varies across the retina, but a relatively constant ratio of 45 photoreceptors per RPE cell is found in all retinal regions.5
Each RPE cell is polarized with an apical part adjacent to the subretinal space and a basal portion facing Bruch’s membrane. The apical surface has numerous long microvilli that envelop the photoreceptor outer segments and are involved in phagocytosis of shed outer segment tips. The apical portion also contains numerous cytoplasmic filaments, microtubules, and football-shaped melanin granules (see Fig. 3). The melanin is thought to absorb stray light, decreasing light scatter within the eye.
The midportion of the RPE cell contains the nucleus, cytoplasmic organelles for protein synthesis (ribosomes, rough endoplasmic reticulum, and Golgi apparatus), smooth endoplasmic reticulum, phagosomes containing outer segment tips undergoing digestion by lysosomal enzymes, and lipofuscin granules. The lipofuscin granules, which increase in number with normal aging, represent residual outer segment lipids incompletely digested by lysosomal activity. The lipofuscin granules are autofluorescent (Fig. 4A) and are relatively sparse in the RPE of children. RPE cells in older eyes are engorged with lipofuscin granules, which also contain incompletely digested melanin granules. The highest density of RPE lipofuscin is found in the macula; this high concentration of lipofuscin granules may compromise RPE functions and has been suggested to cause macular degeneration.6
The lateral and basal surfaces of the RPE cells are highly specialized, containing a variety of different receptors and ion channels. The RPE basal surfaces are infolded, increasing the area of the RPE surface membrane. The basal RPE surface rests on a basal lamina that forms the innermost layer of Bruch’s membrane. The other layers of Bruch’s membrane are the inner collagenous, elastin, outer collagenous, and the basal lamina of the choriocapillaris (see Fig. 3).
The RPE cells have electrogenic sodium-potassium pumps in the apical membrane and chloride-bicarbonate exchange transporters in the basal membrane. The net effect of the pumps, transport systems, and passive ion channels is movement of water across the RPE in a retina-to-choroid direction, promoting adhesion of the neurosensory retina to the RPE.5
INTERPHOTORECEPTOR MATRIX
The RPE secretes some of the molecules that fill the subretinal (interphotoreceptor) space. This space, the remnant of the ventricular lumen present during fetal development, is delineated by the apical surfaces of the photoreceptor, Müller, and RPE cells. The outer margin of the space is established by the zonulae occludentes (tight junctions) between the RPE cells, and the inner margin is defined by the external limiting membrane, formed by intermediate junctions (zonulae adherentes) between photoreceptor inner segments and Müller cell apical processes.7 This space is filled with the interphotoreceptor matrix, a heterogeneous collection of proteins/glycoproteins, enzymes, and glycosaminoglycans/proteoglycans that surround the rod and cone inner and outer segments. Notable components of the interphotoreceptor matrix are interphotoreceptorretinoid binding protein (see Fig. 4B), though to function in transport of vitamin A between photoreceptors and the RPE,4 and the matrix sheaths that surround individual rod and cone outer segments. The matrix sheaths of rods and cones differ in composition: those of the rods are stained by certain plant lectins, notably wheat germ agglutinin, while the cone sheaths are positive with other lectins, including peanut agglutinin (see Fig. 4C). The sheaths of the specialized foveal cones are labeled with wheat germ agglutinin and elderberry bark lectins. These matrix sheaths are thought to isolate individual photoreceptor outer segments, maintain outer segment orientation, and contribute to attachment of the photoreceptors to the apical surfaces of the RPE cells.8
PHOTORECEPTORS
Human retinas, like those of other vertebrates, contain two types of photoreceptor cells, rods and cones. Rod cells mediate dim light vision, while cones function in bright light and are responsible for color vision. The names of these cells are derived from the shapes of their outer segments, which contain the visual pigments. Additional differences are present in the size and shape of the rod and cone inner segments, the location of the cell bodies, and the size and location of their synapses. These features of human cones are revealed by immunocytochemistry using an antibody against the cone molecule, 7G6 (Fig. 5A). Examination of the same microscopic field by Nomarski differential interference contrast microscopy reveals other features of the outer retina, including the external limiting membrane, outer nuclear layer, and layer of photoreceptor axons and synapses (see Fig. 5B).
Humans have a single type of rod with a long, thin outer segment containing the visual pigment, rhodopsin, which absorbs maximally at 500 nm. The cone outer segments are shorter and wider and somewhat conical. Each of the three cone types contains a different cone opsin that absorbs maximally in the red (564 nm; L cones), green (533 nm; M cones), or blue (437 nm; S cones) region of the spectrum. The visual pigments can be demonstrated by immunocytochemistry using antibodies specific for the different proteins. Rod outer segments are strongly labeled with anti-rhodopsin (Fig. 6). The composition of the red cone pigment is so similar to that of the green cone pigment that they cannot be differentiated by immunocytochemistry, but the majority of cone outer segments are labeled with an antibody that recognizes both red- and green-sensitive cones (Fig. 7A). A minority of the cones are blue-sensitive (see Fig. 7B).
![]() Fig. 6. Immunocytochemical demonstration of rhodopsin (red) in human rod outer segments (O). R, retinal pigment epithelium. Cell nuclei (n) are counterstained blue with DAPI. Bar = 20 μm. |
The normal human retina contains approximately 4.6 million cones, with a peak foveal cone density of approximately 199,000 cones/mm2 (Fig. 8). There are approximately 92 million rods, with highest rod density (more than 150,000 rods/mm2) in a ring at the eccentricity of the optic disc.9 Blue-sensitive cones are absent in the foveal center, most numerous (over 2000 cells/mm2) in a ring at 0.1 to 0.3 mm from the foveal center, and constitute approximately 7% of the cones in the periphery.10
The photoreceptor inner segments contain a cluster of mitochondria forming the ellipsoid, and organelles for protein synthesis (ribosomes, rough endoplasmic reticulum, and Golgi apparatus) in the myoid between the ellipsoid and cell nucleus. The rod and cone outer segments are formed by stacks of membranes containing the visual pigments, rhodopsin and the cone opsins, respectively. In rods, the membranes pinch off at the base of the outer segment to form multiple discs resembling a stack of coins, surrounded by the plasma membrane (Fig. 9). There is ongoing addition of newly synthesized rhodopsin into these membrane discs as they form at the base of the rod outer segment. The newly synthesized protein can be traced by light microscopic radioautography after pulse labeling an animal with radioactive amino acids that are incorporated into rhodopsin being synthesized in the inner segment. The radioactive rhodopsin is then inserted into newly forming basal discs and appears as a dark band across the outer segment in radioautographs. These labeled discs are displaced outward by continuous addition of new, unlabeled discs at the outer segment base (Fig. 10). The process of disc addition is balanced by periodic shedding of the outer segment tip, which is phagocytosed and degraded by RPE lysosomal activity. In primates, complete renewal of a rod outer segment occurs in a about 2 weeks. In cones, the outer segment membranes remain continuous with the surface membrane (see Fig. 9), such that newly synthesized photopigment is inserted diffusely into the outer segment, rather than as a band as found in rods. The time course of cone outer segment renewal is not known.
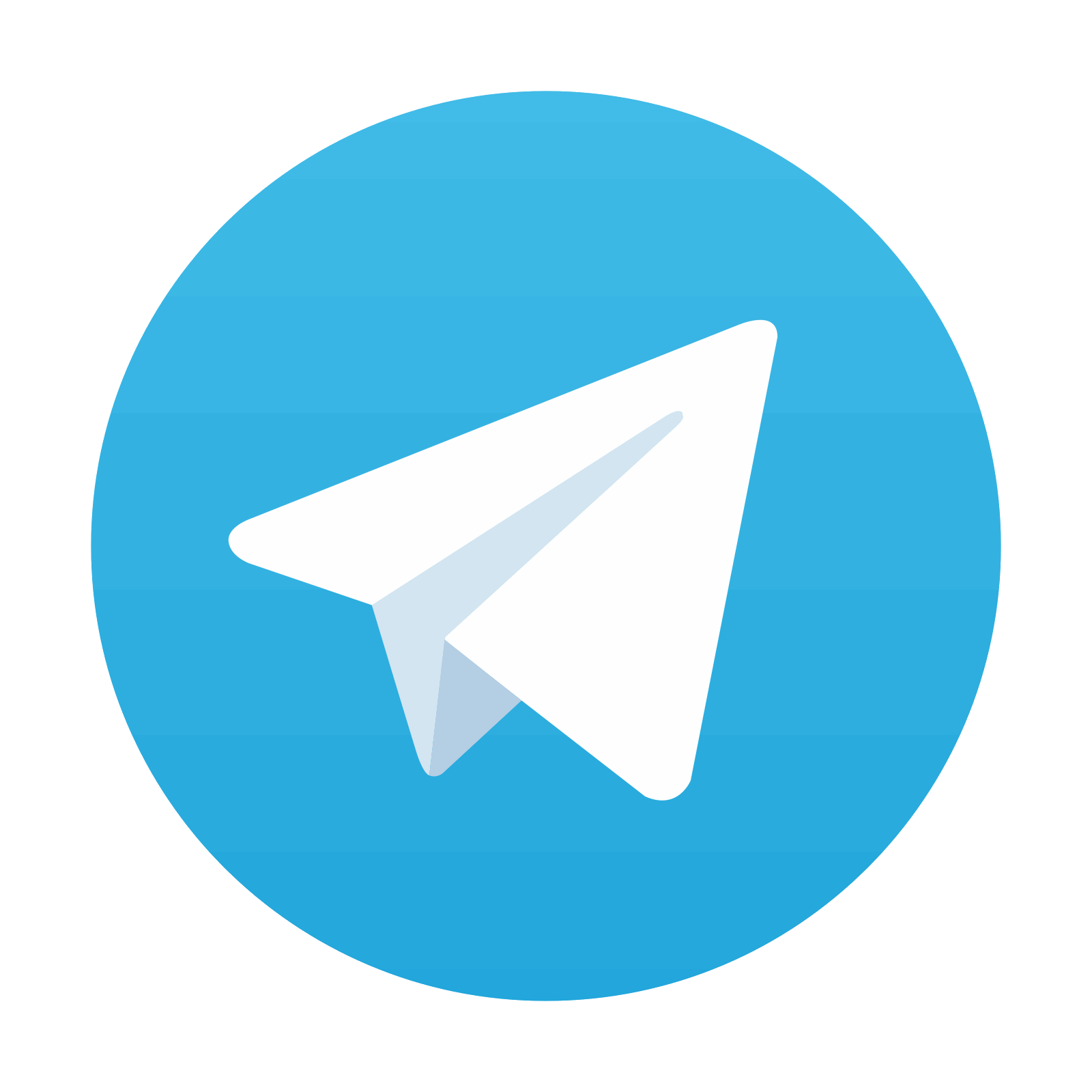
Stay updated, free articles. Join our Telegram channel

Full access? Get Clinical Tree
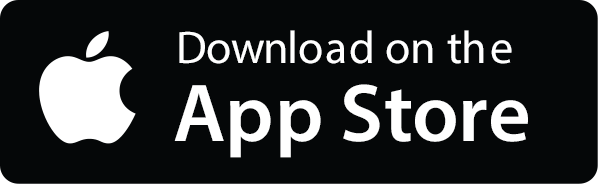
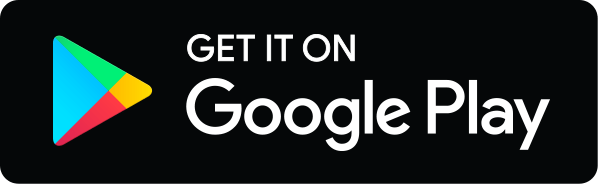