Alpha-Adrenergic Drugs
Joel S. Mindel
CATECHOLAMINE-CONTAINING NEURONS OF THE EYE
The eye is innervated by catecholamine-containing axons originating from the superior cervical ganglion, a sympathetic nervous system structure. These postganglionic axons innervate multiple tissues including the smooth muscle fibers in the iris dilator muscle, conjunctival blood vessels, and lids. The axon terminals release the transmitter, norepinephrine1 resulting in dilation of the pupil, blanching of the conjunctiva, and widening of the palpebral fissure. Catecholamine-containing nerve endings have also been demonstrated in human cornea.2 In the fetus, these are subepithelial; in the adult, they are found only in the stroma. The physiologic role of these corneal fibers is not known but they may mediate epithelial chloride transport; this theory is based on experiments demonstrating that exogenous catecholamines stimulate rabbit corneal epithelium transport by a cAMP related mechanism.3 Catecholamine-containing nerve endings have been demonstrated in the areas of the trabecular meshwork and corneal limbus of mammals, including humans.4,5
Catecholamine-containing nerve fibers are found in their greatest concentrations in the iris dilator muscle and ciliary processes.6 Their numbers are much reduced in the rat iris after superior cervical ganglionectomy, but some fibers remain.7 A limited number of adrenergic fibers have been found in the iris sphincter muscle.8 In chickens and rabbits, the retinal blood vessels have a sympathetic innervation from the superior cervical ganglion.9,10 This innervation is much more extensive in arterioles than venules and falls off rapidly with increasing distance from the optic nerve head. Some, but not all, investigators have shown catecholamine-containing fibers in the retinal blood vessels of human eyes.11,12 Electrical stimulation of the superior cervical ganglion causes a 58% to 70% reduction in the blood flow to the choroid of the rabbit,13 suggesting that this structure, too, has a sympathetic nervous system innervation.
TRANSMITTER SYNTHESIS, RELEASE, AND INACTIVATION
Norepinephrine is synthesized from tyrosine by the sequential actions of tyrosine hydroxylase, dopa decarboxylase and dopamine-beta-hydroxylase. Tyrosine hydroxylase is the rate limiting enzyme. Norepinephrine is stored in granular vesicles located in varicosities in the terminals of sympathetic neurons. Release of norepinephrine may not occur with each action potential. Rather, release is partially a function of the frequency of action potentials.14 Increasing this frequency facilitates norepinephrine release. Dopamine-beta-hydroxylase is released along with norepinephrine. Both have been demonstrated in iris neurons15 and in the aqueous humor.16 Cervical sympathetic stimulation increases their levels. Rat iris contains phenylethanolamine N-methyltransferase and can, therefore, synthesize epinephrine; this synthesis is reduced but not prevented by superior cervical ganglionectomy or by prior treatment with the sympathetic neurotoxic agent, 6-hydroxydopamine.17 Rat retina is also capable of synthesizing epinephrine.18
Released norepinephrine is inactivated primarily by reuptake into the nerve terminals that release it. Reuptake results either in return to a storage vesicle or to degradation by intraneuronal monamine oxidase (MAO). There are at least two forms of MAO, types A and B, both of which have been identified in the iris-ciliary body of rabbits.19 Neuronal reuptake sites exhibit a feedback mechanism. As more norepinephrine is released, the number of reuptake sites increases and as less norepinephrine is released, the number of reuptake sites decreases.20 Smaller quantities of released norepinephrine are inactivated by being absorbed into the postsynatic neuron or smooth muscle fiber where they are destroyed by MAO or catechol-O-methyl-transferase (COMT). The remainder of released norepinephrine diffuses away into the circulation.
Close synaptic contact with a histologically identifiable area of specialized muscle membrane, as occurs in cholinergic striated muscle fibers, does not occur in the adrenergic system.21,22 However, the separation between neuron terminal and muscle fiber found in the iris, 15 to 20 nm, is less than that found at most sympathetic innervations. Released norepinephrine stimulates a muscle bundle, rather than a single muscle fiber, not only because it can diffuse over a larger area but also because there are low-resistance interconnections between adjacent muscle fiber membranes, called gap junctions, that are approximately 1 μm in size.23
RECEPTORS
At pharmacologic doses, drugs may act at nonphysiologic sites as well as at physiologic receptors. Multiple types of adrenergic receptors have been identified. Initially, these were classified as alpha and beta.24 Subsequently, these have been subdivided.25 There are at least two types of alpha-adrenergic receptors, alpha-1 and alpha-2; norepinephrine stimulates both types. Alpha-1 receptors were believed to be purely postjunctional (e.g., on smooth muscle membranes) and alpha-2 receptors were believed to be purely prejunctional (e.g., on norepinephrine-releasing neuron terminals). However, this distinction has not held up. Current terminology refers to receptors by type (e.g., alpha-1 or alpha-2) and does not assume location. Other receptors that may be found on norepinephrine-releasing neurons include beta-adrenergic, dopaminergic, nicotinic, muscarinic, histaminergic, serotinergic, angiotensinergic, opiatergic, prostaglandinergic, GABAergic and adenosinergic. Receptor subtype does not determine function. For example, stimulation of beta-2 adrenergic prejunctional receptors may enhance or inhibit norepinephrine release.26 There is no a priori way of knowing which.27
Adrenergic receptors are polypeptides of molecular weights between 64,000 and 80,000 daltons. They are glycoproteins and have structural similarities to rhodopsin.28 The receptors are coupled to guanine nucleotide regulatory proteins which, in turn, are linked to various cytoplasmic effector enzymes. The guanine nucleotide regulatory protein is a tripeptide, consisting of alpha, beta and gamma subunits, activated when the adrenergic receptor is stimulated by the binding of a GTP molecule. The activated protein then dissociates into subunits of one or two of its peptide chains. One of these subunits activates the effector enzyme while the other subunit(s) activates or inhibits other enzymes,29 resulting in an enhanced or reduced response. Alpha receptors do not seem to elevate adenyl cyclase activity but alter calcium levels and may cause hydrolysis of polyphosphoinositides. Polyphosphoinositides, in turn, may generate one or two secondary messengers, diglycerol and inositol triphosphate. Alpha-2 receptors often reduce adenyl cyclase activity.30 Both alpha-1 and alpha-2 receptors are believed to act primarily through alterations in calcium ion fluxes.31 Two subtypes of alpha-1 receptors can be identified pharmacologically, alpha-1A and alpha-1B, and a third and fourth subtype, alpha-1C and alpha-1D, may exist.32,33,34,35 Alpha-1A does not stimulate inositol triphosphate formation but causes influx of extracellular calcium. Alpha-1B stimulates inositol triphosphate and releases intracellular calcium. Thus both subtypes of alpha-1 receptor would raise intracellular levels of calcium but only the alpha-1A subtype would be dependent on extracellular calcium. In contrast to alpha receptors, beta-1 and beta-2 adrenergic receptors activate a guanine nucleotide regulatory protein that, in turn, activates membrane-bound adenyl cyclase. The activated adenyl cyclase catalyzes the formation of the intracellular second messenger, cyclic AMP, from ATP.36
Before prejunctional receptors were identified, norepinephrine release was believed to be dependent only on the rate of axon stimulation. However, when stimulation of prejunctional alpha-2 receptors was found to inhibit norepinephrine release, the importance of this feedback mechanism was quickly appreciated. Alpha-2 receptors were subsequently identified in postjunctional neurons in the central nervous system. In general, drugs do not distinguish between pre- and postjunctional alpha-2 receptors. However, drugs do distinguish between different alpha-2 receptors and a further subdivision has been made (e.g., alpha-2A, alpha-2B,37,38 alpha-2C and alpha-2D).39,40 Alpha-2 receptor subtypes have been cloned and sequenced. The alpha-2A receptor resides on human chromosome 10, so an alternative name was given, alpha 2-C10; for similar reasons alpha-2B is also known as alpha 2-C2 and alpha-2C as alpha 2-C4. Alpha-2C receptors have been found in human retinoblastoma cells.41 Prazosin, imiloxan, and chlorpromazine bind preferentially to alpha-2B receptors rather than to alpha-2A receptors; prazosin and chlorpromazine, but not imiloxan, bind readily with alpha-1 receptors as well. Rauwolscine binds equally well to alpha-2A and -2B receptors, but not to alpha-1 receptors. Oxymetazoline binds preferentially to alpha-2A receptors but not to alpha-2B or alpha-1 receptors.42 To further confuse a complex situation, clonidine, which has been considered an alpha-2 agonist, has been found to stimulate another type of receptor as well. This second type of receptor is activated by imidazoline but not by catecholamine.43
SUBSENSITIVITY AND SUPERSENSITIVITY
The number of adrenergic receptor binding sites changes, with time, inversely to the amount of released neurotransmitter.44 A prolonged increase in norepinephrine release results in receptor sites becoming desensitized by a number of mechanisms (e.g., by becoming phosphorylated), which changes their structure, or by becoming sequestered (i.e., removed from the cell membrane surface and placed into vesicles).45 For example, the agonist-occupied beta-2 receptor can be phosphorylated by an enzyme called beta-adrenergic receptor kinase; phosphorylation results in decreased coupling to the guanine regulatory protein.46 Sensitivity to an agonist can also be altered by changes in other receptors. For example, long-term activation of CNS beta receptors will increase the number of alpha-2 receptors.47 Different subtypes of alpha-2 receptors undergo different desensitizations to agonists that may explain their evolutionary significance (i.e., to meet differing needs for down-regulation).48
Supersensitivity to an adrenergic transmitter can be produced by its absence. Removal of the rat superior cervical ganglion results in decreased levels of released catecholamines.49 Simultaneously, the degenerating iris nerve terminals, unable to release norepinephrine, gradually increase their content of catecholamines to a maximum of 25% above baseline at 12 hours, followed by a decline to complete disappearance at 24 hours. Iris supersensitivity to alpha-adrenergic agonists occurs within 2 weeks.50 Supersensitivity means that a lower agonist concentration is needed to produce a given submaximal or maximal response, a so-called “shift to the left” of the dose-responsecurve. The magnitude of the maximum response produced may or may not also be increased in the supersensitive state depending on the tissue and the response being measured.
Supersensitivity of skeletal muscle is associated with a marked increase in the number of nicotinic cholinergic receptors. However, supersensitivity in adrenergically innervated smooth muscle is not associated with a dramatic increase in the number of alpha receptors. Several weeks after superior cervical ganglionectomy the rabbit iris exhibits a small decrease in the total number of alpha receptors, presumably due to loss of the prejunctional receptors on the nerve endings.51 However, there may be small increases in the numbers of postjunctional alpha-2 and beta receptors.52 The binding affinities of the alpha and beta receptors remain unchanged. Other mechanisms are needed to explain alpha-adrenergic supersensitivity.53 One is the absence of a neuronal reuptake mechanism that can inactivate the agonist. While this has been claimed to be the primary, or exclusive, cause of iris supersensitivity in rats,54 it does not explain the iris supersensitivity that occurs after interruption of the neuronal pathway proximal to the superior cervical ganglion. Such a preganglionic interruption leaves the postganglionic neurons, and their reuptake mechanisms, intact. A second cause of supersensitivity may be an alteration of the muscle fiber’s cell membrane not involving the number of receptors. The transmembrane resting potential may be reduced or enzyme kinetics may be enhanced. For example, an increase in norepinephrine-stimulated phosphatidylinositol 4, 5-diphosphate breakdown occurs in the supersensitive rabbit iris dilator muscle.
DRUG EFFECTS ON ADRENERGIC FUNCTION
The discovery of multiple subclasses of alpha-adrenergic receptors has altered the classification of drugs. A given drug may be an agonist at one type of alpha receptor but not at another. Binding does not necessarily correlate with agonist activity. For example, clonidine and apraclonidine bind relatively indiscriminately to the various alpha-2 subtypes but both preferentially activate alpha-2A receptors. Structurally, alpha-adrenergic agonists tend to be either catecholamine derivatives or imidazoline derivatives. Catecholamine contains a 6-carbon unsaturated (benzene) ring. Imidazolines have a 5-membered ring consisting of 3 carbon and 2 nitrogen atoms. Imidazolines usually have a longer duration of action. Examples of catecholamine derivatives are norepinephrine, epinephrine, phenylephrine, tyramine, hydroxyamphetamine, and ephedrine. Examples of imidazoline derivatives are clonidine, phentolamine, naphazoline, tetrahydrozoline, antazoline, tolazoline, yohimbine, oxymetazoline, and xylometazolone.
TABLE 1. Most Prominent Activities of Alpha-Adrenergic Drugs | ||||||||||||||||||||||||||||||||||||||
---|---|---|---|---|---|---|---|---|---|---|---|---|---|---|---|---|---|---|---|---|---|---|---|---|---|---|---|---|---|---|---|---|---|---|---|---|---|---|
|
DIRECT AGONISTS
Norepinephrine is approximately equipotent as an alpha-1A, alpha-1B, and alpha-2 agonist. Phenylephrine is primarily an alpha-1 agonist57 with both alpha-1A and alpha-1B activity. At eqimolar concentrations phenylephrine has 15% of norepinephrine’s alpha-1 potency but less than 1% of norepinephrine’s alpha-2 potency.58 At high concentrations phenylephrine can stimulate beta receptors and produce a rise in cAMP.59 The neuronal reuptake mechanism is less sensitive to phenylephrine than to naturally occurring catecholamines,60 but once phenylephrine enters a neuron, it can reduce catecholamine synthesis by up to 50%.61 Phenylephrine can be converted to epinephrine by hydroxylation in the liver.62 Epinephrine is more than 600 times as potent as norepinephrine at alpha-2 receptors and is also a potent alpha-1 agonist.58 Methoxamine is a selective alpha-1A agonist not metabolized by monoamine oxidase. Apraclonidine and clonidine are preferential alpha-2A agonists; however, at higher concentrations, alpha-1 effects begin to appear. Clonidine is also an adenosine antagonist.63 Naphazoline is a preferential alpha-2 agonist. Oxymetazoline, like apraclonidine, binds relatively indiscriminately toalpha-2 receptor subtypes but, especially at low concentrations, is a relatively specific alpha-2A agonist.
INDIRECT AGONISTS
Amphetamines act by causing release of stored monoamines (e.g., norepinephrine, dopamine and serotinin) from synaptic vesicles and by inhibiting their reuptake as well.64,65 They may also be direct alpha-2 antagonists.66
Cocaine inhibits the reuptake of monoamines.67,68,69 Cocaine is benzoylmethylecgonine, a naturally occurring alkaloid accounting for 0.7% to 1.5% of the total weight of coca leaves. Cocaine is inactivated in many strains of rabbits by the serum enzyme, cocainesterase.70 In humans, cocaine is hydrolized by serum cholinesterase71 and hepatic esterases72; smaller amounts are N-demethylated in the liver.73 Cocaine is not metabolized in the plasma of patients with cholinesterase deficiency who have prolonged apnea to succinylcholine.74 When 1.5 mg/kg is applied intranasally in subjects with normal cholinesterase activity, peak plasma levels occur in 15 to 60 minutes and cocaine is cleared from the serum with a half-life of 3.8 hours.75 Cocaine is relatively stable at acidic pH’s but undergoes spontaneous hydrolysis as the pH is raised.76 Solutions below pH 4 appeared relatively stable when stored at 25°C for 45 days, while increasingly rapid hydrolysis occurred as the pH was raised from 5.5: phosphate buffer accelerates hydrolysis while carbonate buffer does not.77,78
Cocaine is both a local anesthetic and an indirect adrenergic agonist. The local anesthetic activity correlates well with cocaine’s ability to stabilize neuronal cell membranes by inhibiting sodium movement through ion channels.79 The indirect adrenergic effect results from cocaine’s competing with monoamines (e.g., norepinephrine, dopamine and serotonin) for the reuptake mechanism of peripheral sympathetic neurons.68,69
Tyramine is actively taken up by nerve endings and causes release of stored norepinephrine.
DIRECT ANTAGONISTS
Dapiprazole, hydralazine, prazosin, and thymoxamine are primarily alpha-1 antagonists. For example, thymoxamine is 100 times as potent at alpha-1 receptors than it is at alpha-2 receptors. Phenoxybenzamine and phentolamine are relatively nonselective alpha antagonists. Rauwolsine and yohimbine are primarily alpha-2 antagonists.
INDIRECT ANTAGONISTS
Guanethidine both produces release of stored norepinephrine into the cytoplasm, where it is destroyed, and prevents norepinephrine reuptake. Reserpine destroys neuronal storage vesicles. 6-Hydroxydopamine destroys the adrenergic nerve endings that take it up.
OCULAR PHARMACOLOGY–ANIMAL STUDIES
CORNEA
Alpha-1 receptors have been identified in cell cultures of rabbit, bovine, and human corneal endothelium.80 Their physiologic role, and the effects of their pharmacologic stimulation, are not known.
LACRIMAL SECRETION
Alpha-1 agonists, such as phenylephrine and norepinephrine, stimulate protein secretion by lacrimal gland acini in the rat; alpha-1 antagonists inhibit this effect.81 Drugs that are primarily alpha-2 or beta agonists have no stimulatory effect.
INTRAOCULAR PRESSURE
Unilateral sympathectomy proximal to the cervical ganglion reduces the ipsilateral aqueous humor norepinephrine level and prevents the dark-induced elevation in intraocular pressure found in many mammalian species.82,83,84,85 Melatonin and cAMP levels in the aqueous humor do not appear related to the phenomenon.86 The circadian elevation in intraocular pressure can be blocked by an alpha-1 antagonist, prazosin, but not by the alpha-2 antagonist, rauwolscine, or by the nonselective beta blocker, timolol.87 Treatment with apraclonidine, an alpha-2 agonist, reduces aqueous humor norepinephrine. This has led to speculation that stimulation of prejunctional alpha-2 receptors might reduce norepinephrine release and blunt the circadian elevation in intraocular pressure.
Oxymetazoline, a relatively selectively alpha-2A agonist is used in low concentrations (e.g., 0.001% to 0.05%) as a conjunctival vasoconstrictor; at these concentrations it does not lower the intraocular pressure in man.88 However, at concentrations of 0.1% to 1.5%, it minimumly but significantly lowers the intraocular pressure of normal rabbits and monkeys and, markedly, of glaucomatous monkeys.89 This reduction in pressure is associated with a decreased aqueous humor flow rate and an increased uveoscleral outflow.
Topical norepinephrine causes an initial intraocular pressure elevation that is more marked in rabbits with prior superior cervical ganglionectomy.90 The intraocular pressure returns to baseline in about 1 hour and then continues to fall, reaching a minimum about 3 to 6 hours after administration.91,92 The early hypertensive effect appears to be the result of extraocular muscle stimulation and co-contraction, because disinserting the extraocular muscles prevents it.93 This hypertensive response can be prevented by prior administration of the alpha-1 antagonist, phenoxybenzamine. Alpha-2 agonists prolong the rabbit hypotensive phase while alpha-2 antagonists (e.g., yohimbine) prevent it.94 The increase in trabecular outflow facility found in monkeys and rabbits after topical, intracameral, or intravitreal injection of norepinephrine or epinephrine appears to be mediated by beta-2 receptors.95,96 Phenylephrine will not produce increased outflow facility. Pretreatment with timolol prevents the norepinephrine induced increase in outflow facility while pretreatment with the relatively specific beta-1 antagonist, betaxolol, does not. Norepinephrine reduces aqueous humor formation while epinephrine has little effect and phenylephrine has none; none of the three drugs affects episcleral venous pressure.97
Clonidine, an alpha-2 agonist and also an adenosine antagonist,63 produces variable transient effects on intraocular pressure in cats, depending on the route of administration.98 Unilateral topical application of clonidine produces a slight decrease in blood pressure but a marked bilateral intraocular pressure reduction; clonidine levels in the contralateral eye are insufficient to explain this reduction.99 When injected into the external carotid artery, clonidine produces a transient decrease in intraocular pressure followed by an increase associated with contraction of the eyelids and extraocular muscles.100 This elevation lasts 5 to 10 minutes, followed by a prolonged hypotension. When injected into the vertebral artery or intravenously, the intraocular pressure is reduced. Chronic superior cervical ganglionectomy in cats produces an enhanced bilateral hypotensive response to intravenous clonidine.101 These results demonstrate that clonidine produces ocular hypotension through central nervous system mechanisms as well as ocular mechanisms, such as anterior segment vasocontriction and aqueous humor secretion reduction.102 In rabbits, ventriculocisternal brain perfusion with clonidine results in reduced intraocular pressure. However, a simultaneous lowering of systemic systolic and diastolic blood pressure occurs, suggesting that, in this circumstance, ocular hypotension is largely a secondary drug effect.103
In rabbits, apraclonidine 0.5% given locally reduces intraocular pressure significantly 4 to 24 hours after treatment. When drops are given unilaterally 1 hour before and immediately after bilateral iris laser treatment, an intraocular pressure elevation fails to occur in treated eyes.104 Aqueous humor protein concentrations, but not prostaglandin E2 concentrations, are reduced in apraclonidine treated eyes; phenylephrine 5% topically does not reduce aqueous humor protein elevations, which suggests that this apraclonidine effect is alpha-2 receptor mediated.
IRIS
The rabbit iris dilator muscle is stimulated by alpha-1 adrenergic agonists. The result is mydriasis. In vitro, strips of rabbit dilator muscle contract to norepinephrine, an alpha-1A and alpha-1B agonist, but not to methoxamine, a selective alpha-1A agonist.105 This suggests that alpha-1B receptors predominate. However, in vivo, methoxamine produces mydriasis. Norepinephrine increases the turnover of iris phosphatidic acid and phosphatidylinositol.106 Inhibition of rabbit monoamine oxidase causes an increased iris dilation from topical norepinephrine. Eight days after a single unilateral injection of pargyline, the two pupils have equal diameters but the treated iris will have a nearly three-fold greater response to a topical 1.5% norepinephrine solution.107 However, the pupil response to methoxamine, a direct-acting selective alpha-1A agonist not metabolized by monoamine oxidase, remains unchanged. Alpha, but not beta, antagonists prevent mydriasis.108
Reserpine, a sympatholylic agent that destroys storage vesicles, injected intraperitoneally, 3 mg/kg, is as effective as superior cervical ganglionectomy in producing miosis and markedly reducing rat iris catecholamines.50 By 6 hours, all iris catecholamines have completely disappeared.
Amphetamine or cocaine, adminstered systemically, produces pupil dilation. Amphetamine acts both by causing release of stored monoamines from synaptic terminals and by inhibiting their reuptake as well.65 Amphetamine, 10 mg/kg, causes a 65% increase in extraneuronal catecholamines within 30 minutes, followed by a gradual reduction in intraneural catecholamines to 30% of baseline levels by 2 hours. Cocaine inhibits the reuptake of a number of monoamines.67 Cocaine, 10 mg/kg, causes a significant (100%) increase in extraneuronal iris catecholamines within 30 minutes but has no effect on intraneuronal levels. By 2 hours postinjection, cocaine’s effect on extraneuronal catecholamines is gone. Both amphetamine and cocaine induced elevations in extraneuronal catecholamines correlate well with the pupil dilation. Neither cocaine nor amphetamine produces pupil dilation in surgically denervated irides. However, imipramine, a drug that inhibits norepinephrine reuptake, does produce dilation of the denervated rat iris due to its potent anticholinergic (antimuscarinic) sphincter effect.109,110
Rabbits kept in continuous light for 1 week maintain a miosis that is, presumably, in part due to a sustained reduced sympathetic stimulation of the dilator muscle. There is a resultant supersensitivity to the mydriatic effects of epinephrine and norepinephrine.111 Interestingly, however, constant dark, with resultant mydriasis, does not result in subsensitivity to these agonists. The supersensitive surgically denervated rabbit iris responds to norepinephrine with an exaggerated increase in phosphatidic acid and phosphatidylinositol production.106 Repeated topical application of epinephrine results in reduced dilation and a reduction in induced inositol triphosphate and diacylglycerol formation.112
Amphibian responses may differ from those of mammals. In frogs, topical application of beta-1 or beta-2 agonists produces prolonged mydriasis. Beta blockers produce miosis. Alpha agonists (e.g., phenylephrine) and alpha antagonists (e.g., phenoxybenzamine) have some effect on pupil diameter but much less so than beta-adrenergic drugs.113
Alpha-2 agonists inhibit the release of norepinephrine from sympathetic neurons innervating the iris dilator muscle.114 The result should be miosis. In rats and cats, alpha-2 agonists may induce a partial mydriasis by a central nervous system effect.115,116,117 Alpha-2 stimulation of the dorsal midbrain area (Edinger-Westphal nucleus) inhibits the parasympathetic innervation of the iris sphincter muscle. In cats, a dose related mydriasis could be achieved by direct injection of small amounts, 0.5 mg, of amphetamine into the third ventricle; this too was associated with inhibition of ciliary nerve activity.118
Iris stromal pigmentation occurs in early life and is dependent on an intact adrenergic innervation. However, melanin formation in the iris pigment epithelium is not dependent on the sympathetic nervous system. The hypopigmentation of iris stromal melanocytes, produced by denervation, can be mimicked pharmacologically in newborn rabbits by topically applying the alpha-adrenergic antagonist, thymoxamine, 0.5%, three times a day for 12 weeks.119 Similar application of the beta blocker, timolol 0.5%, is ineffective.
Phenylephrine oxazolidine is a more lypophilic prodrug of phenylephrine and better penetrates the corneal epithelium.%119a Topical application of phenylephrine oxazolidine to rabbits will produce as much mydriasis as a phenylephrine HCl solution 10 times as concentrated. Unfortunately, the prodrug is unstable in water and must be formulated as to suspension in sesame oil. A pivalic acid prodrug of phenylephrine is more stable and also about 10 times as active as phenylephrine; the unmetabolized prodrug itself may have significant intrinsic adrenergic activity.120
CILIARY BODY
Phenylephrine causes an acute localized constriction in the arterioles supplying the ciliary processes%120a and a transient increase in prostaglandin synthesis and release.121,122 However, after 7 weeks of treatment, rabbit arterioles constrict only 20% compared to that initially. Epinephrine also reduces blood flow to the rabbit iris and ciliary processes, but isoproterenol, a beta agonist without alpha-adrenergic activity has no effect.123 These results suggest that blood flow to the ciliary processes is controlled by alpha-adrenergic receptors. Binding studies show that iris-ciliary body alpha receptors have characteristics typical of alpha-1 receptors (i.e., their order of binding potency is epinephrine < norepinephrine < isoproterenol).124 In addition, rabbit ciliary body contains many alpha-2A receptors.125 Competitive binding studies with iodoclonidine are consistent with those shown by other alpha-2A receptors (i.e., the sequence of binding affinities is oxymetazoline < chlorpromaÜbk 4Ý Üol 0Ý zine < Ümh- 15Ý< prazosine).
RETINA
Alpha-1 and alpha-2 adrenergic binding sites and norepinephrine have been identified in bovine retinal blood vessels.126 The norepinephrine is assumedto be in sympathetic axon terminals because its level becomes almost undetectable following superior cervical ganglionectomy.127,128,129,130,131 There are norepinephrine accumulating cells in the neuroretina, but these cells do not synthesize the transmitter.132 However, the enzyme for epinephrine synthesis, phenylethanolamine-N-methyltransferase, has been identified in a subpopulation of rat amacrine cells.133 Neuroretina contains alpha-2 receptors almost to the exclusion of alpha-1 receptors.134,135
OCULAR PHARMACOLOGY–HUMAN STUDIES
LIDS
Stimulation of the sympathetically innervated smooth muscle fibers causes lid elevation. Ten normal subjects had unilateral application of 2.5% phenylephrine, 10% cocaine, 1% hydroxyamphetamine and 1% apraclonidine.136 At least 24 hours passed between testing each drug. Two drops of each medication were placed in the eye, separated by 10 seconds. Photographs were taken at 1, 3, 5, 10, 15, 30, and 60 minutes. The onset of palpebral fissure widening began soonest with phenylephrine but its duration was the shortest. Hydroxyamphetamine took the longest to reach its maximum effect, 20 to 25 minutes. The mean maximum effect was approximately 1.5 mm and occurred between 10 to 15 minutes after application. The effect began to decline by the 20th minute. By 1 hour, approximately 0.7 mm of widening remained. There was no significant difference in the maximum effect of any of the four drugs.
Naphazoline, 0.1%, a preferential alpha-2 agonist with some alpha-1 activity, given topically, elevates the upper lid, presumably due to the alpha-1 stimulatory effect on Müller’s muscle.137 However, continued use several times a day for several weeks results in tolerance and a reduced effect. The acute lid effect lasts 2 hours and begins as soon as 5 minutes after instillation. Naphazoline drops also produce conjunctival vasoconstriction and a slight, but statistically significant, pupil dilation (median pupil diameter predrop is 4 mm, and 30 minutes postdrop is 4.5 mm); there is no significant change in intraocular pressure. When a single drop of apraclonidine, 1% is applied, lid retraction is maximum between 1 and 3 hours after instillation.138
Thymoxamine, an alpha-1 antagonist, topically139 and systemically,140 can cause paresis of the adrenergically innervated Müller’s muscle of the upper lid; this effect could be valuable in treating lid retraction. Guanethidine depletes adrenergic stores and has been used to treat thyroid disease-related lid retraction.141 Unfortunately, chronic use results in conjunctival hyperemia, irritation, and even scarring.142
CORNEA
Alpha receptors have been demonstrated in the cultures of epithelium of intact corneas of humans.143 The binding of H3-prazosin, an alpha-1 antagonist, was competitively inhibited by the agonists, phenylephrine and norepinephrine; the alpha agonist, methoxamine, stimulated phosphatidylinositol 4, 5-bisphosphate hydrolysis. The physiologic role of these receptors is not known.
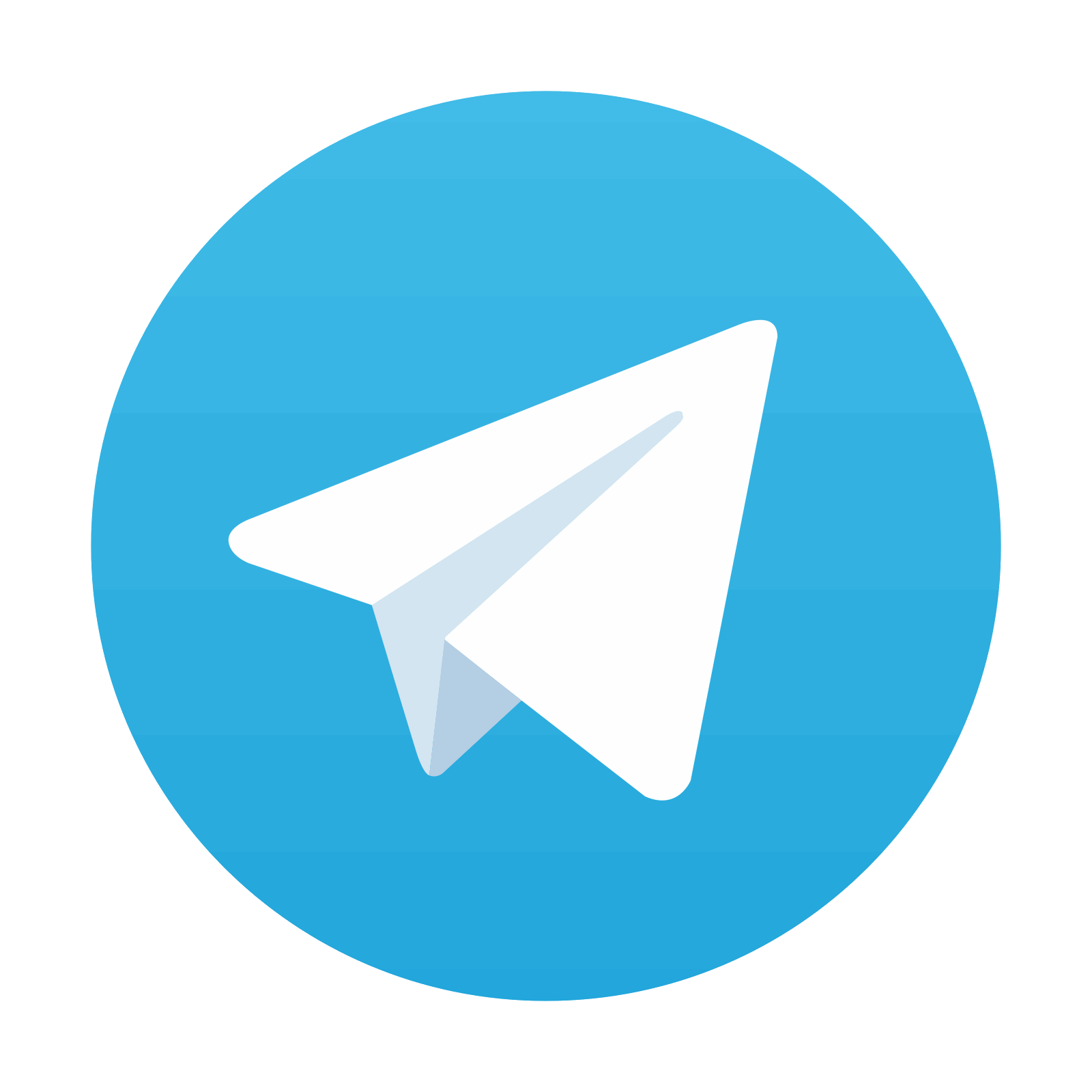
Stay updated, free articles. Join our Telegram channel

Full access? Get Clinical Tree
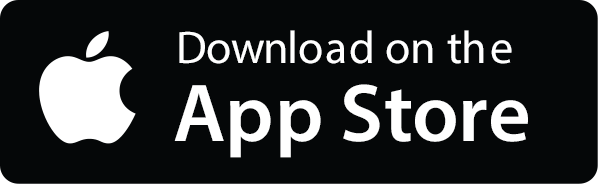
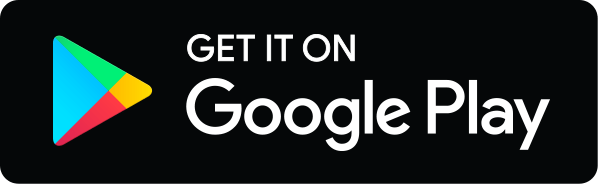