Ultrashort Pulsed Lasers In Corneal Applications
Ronald M. Kurtz
Melvin A. Sarayba
Tibor Juhasz
CORNEAL PHOTODISRUPTION
The mechanism of photodisruption in the cornea is similar to that in other materials, beginning with a process termed laser-induced optical breakdown (LIOB). In LIOB, a strongly focused, short-duration laser pulse generates a high-intensity electric field, leading to the formation of a mixture of free electrons and ions called a plasma (1). The plasma expands with supersonic velocity, rapidly cooling and displacing surrounding tissue (2, 3, 4, 5). As the plasma expansion slows, the supersonic displacement front propagates through the tissue as a shock wave. The shock wave loses energy and velocity as it propagates, ultimately relaxing to an ordinary acoustic wave (6). Because adiabatic expansion of the plasma occurs on a time scale that is short in comparison to the time required for heat transfer, thermal effects are minimized or avoided. The cooling plasma vaporizes a small volume of corneal tissue, forming a cavitation bubble that consists mainly of CO2, N2, and H2O (7).
The specific characteristics of photodisruption depend primarily on the pulse duration, pulse energy, and focusing geometry of a particular laser system. Until recently, ophthalmic photodisruption was limited to a few intraocular procedures, such as iridotomy and capsulotomy, due to the relatively large energies needed to initiate LIOB with available nanosecond pulse-duration neodymium:yttrium-aluminum-garnet (Nd:YAG) lasers (8). The resulting large shock waves and cavitation bubbles produce significant collateral tissue effects. Reducing either the focal spot size or the pulse duration of the laser decreases the threshold energy required for LIOB (2, 3, 4, 5, 6,9). Although a smaller spot size can be achieved with a large focusing angle, this makes scanning systems for delivery of pulses over large areas (such as the cornea) impractical. Alternatively, the laser pulse duration can be decreased from the nanosecond (10−9 sec) to the femtosecond regime (10−15 sec).
In contrast to the nanosecond systems, femtosecond laser photodisruption requires lower laser energy, minimizing collateral tissue effects that scale with energy. Photodisruptive shock wave size (radial propagation distance to decay to an acoustic wave) and cavitation size (maximum bubble radius) for pulses in the few hundred femtoseconds range are significantly smaller than those generated by picosecond (10−12 sec) and nanosecond pulses. The small amount of energy deposited in tissue and the rapid (faster than 1 μs) adiabatic plasma expansion prevent significant heat transfer to surrounding tissue. Narrow collateral tissue damage zones have been demonstrated in cadaver corneal tissue with femtosecond photodisruption (10,11).
The cornea presents an attractive target for femtosecond laser surgical applications because its superficial location and transparency make it easily accessible to femtosecond pulses with negligible nonlinear focusing effects. To be used for high-precision cutting applications, multiple laser pulses can be placed contiguously to create incision planes within the cornea in nearly any orientation (horizontal, vertical, or oblique; Fig. 83-1). The only limitation to creating arbitrary incision planes is that pulses must be delivered in a deep to superficial order, because the cavitations bubbles created can block the optical path to deeper tissue. Intersecting these resection planes can create complex shapes. The lack of vascular structures in the cornea is another benefit of working in this tissue, because photodisruptive lasers do not coagulate blood vessels.
COMMERCIAL SYSTEMS
The IntraLase FS (IntraLase Corp., Irvine, CA) is currently the only clinically available femtosecond laser surgical system. It consists of a high repetition rate (10-15 kilohertz), femtosecond laser source, and precision scanning delivery system that allows creation of uniform lamellar resections within a diameter of approximately 10 mm and to a depth of 500 μm (Fig. 83-2). To attain depth reproducibility in the 10 μm range, the system utilizes an applanation lens to temporarily flatten the anterior surface of the cornea. The surgeon first fixes the eye’s position with a limbal suction
ring and then places the applanating lens, securing it via an internal clamp. This process mechanically couples the eye to the beam delivery system, thereby maintaining a fixed reference distance from the laser’s focusing objective (Fig. 83-3).
ring and then places the applanating lens, securing it via an internal clamp. This process mechanically couples the eye to the beam delivery system, thereby maintaining a fixed reference distance from the laser’s focusing objective (Fig. 83-3).
![]() FIGURE 83-1. Laser pulses are delivered contiguously to create a resection. Orientation can be horizontal, vertical, or oblique. |
The IntraLase FS has received Food and Drug Administration (FDA) clearance for four surgical indications: creation of a hinged LASIK flap, anterior lamellar keratoplasty, channel formation for intracorneal ring segments, and in-situ keratomileusis for the correction of myopia. Several additional applications are under development, including posterior lamellar keratoplasty and so-called intrastromal refractive procedures.
CREATION OF LASIK FLAP
Rationale
LASIK’s dominance in refractive surgery results from its rapid visual rehabilitation, minimal postoperative discomfort, and high predictability, all of which can be linked to creation of a corneal flap that avoids significant disturbance of the epithelial surface (11, 12, 13). Although primarily responsible for these advantages, the flap is also the main source of vision-threatening LASIK complications such as free caps; globe perforation; incomplete or irregular flaps; and thin, thick, and buttonhole flaps. Flap-related LASIK complications occur in as many as 8.8% of cases (14, 15, 16, 17). Even when performed without complication, mechanical flap creation has been associated with significantly nonuniform resections, which may influence refractive outcomes (18).
![]() FIGURE 83-2. A uniform planar cut (arrows) with sharp edges is demonstrated on a cadaver eye using optical coherence tomography. |
Femtosecond Laser Surgical Technique
A LASIK flap is created by scanning a pattern of laser pulses (approximately 500,000 to 800,000) at a desired depth parallel to the applanated corneal surface. After the resection plane is created, a side cut is made by advancing the laser toward the surface in a circular pattern. A hinge can be placed at any meridian by blocking the beam for a short time during each circle pass. When the laser reaches the surface, gas bubbles escape from the interface and the suction is released. The flap can then be elevated for excimer laser ablation, similar to traditional LASIK with the mechanical microkeratome. Both spiral (circular) and raster (X-Y) scan patterns (Figs. 83-4 and 83-5) can be used to create the planar cut. In addition, a reservoir (termed a “pocket”) can be formed outside of the planar cut to create a drainage channel for cavitation bubbles, thereby reducing the spread of gas into the stroma. The most common method now used for flap creation utilizes the raster scan pattern and reservoir depicted in Fig. 83-6.
Early Clinical Studies
In cadaver eye studies (19), excellent reproducibility was identified for key flap parameters such as thickness (standard deviation 12 μm) and diameter (standard deviation 200 μm). Initial human flap resections performed in 46 eyes in 1999 and 2000 (19,20) demonstrated no significant intraoperative complications. Suction and/or applanation were lost during the procedure in several eyes; however, all such cases underwent a subsequent successful procedure on the same or next surgical day (within 24 hours). No postoperative inflammation, diffuse lamellar
keratitis, or flap dislocations were observed and none of the eyes experienced a loss of more than one line of best spectacle-corrected visual acuity (BSCVA).
keratitis, or flap dislocations were observed and none of the eyes experienced a loss of more than one line of best spectacle-corrected visual acuity (BSCVA).
Initial U.S. Clinical Series
The initial U.S. clinical series was completed between June and October 2000 (21), with follow-up through 2001. A total of 208 procedures were performed by two surgeons using a first-generation femtosecond laser model to create the corneal flap and either a VISX S2 or Technolas 217 for excimer ablation. Complications included loss of suction in 4 eyes (1.9%), all of which were successfully completed after a delay of 5 to 45 minutes. Postoperative flap complications such as striae, dislocation/slippage, diffuse lamellar keratitis, and epithelial downgrowth were not seen in this series, and no change in the standard LASIK nomograms of each surgeon was required. All eyes in the low myopia group (less than −3 D) were within one diopter of emetropia, whereas 95% were within 0.5 D. All of the low myopia eyes achieved uncorrected visual acuity (UCVA) of 20/30 or better at 6 months, without benefit of any retreatments. For the moderate myopia group (−3 to −6 D), 96% were within one diopter of emetropia, whereas 96% achieved UCVA of 20/40 or better. In the high myopia group (greater than −6 D), 95% of eyes were within
one diopter of emetropia, whereas 92% achieved UCVA of 20/40 or better.
one diopter of emetropia, whereas 92% achieved UCVA of 20/40 or better.
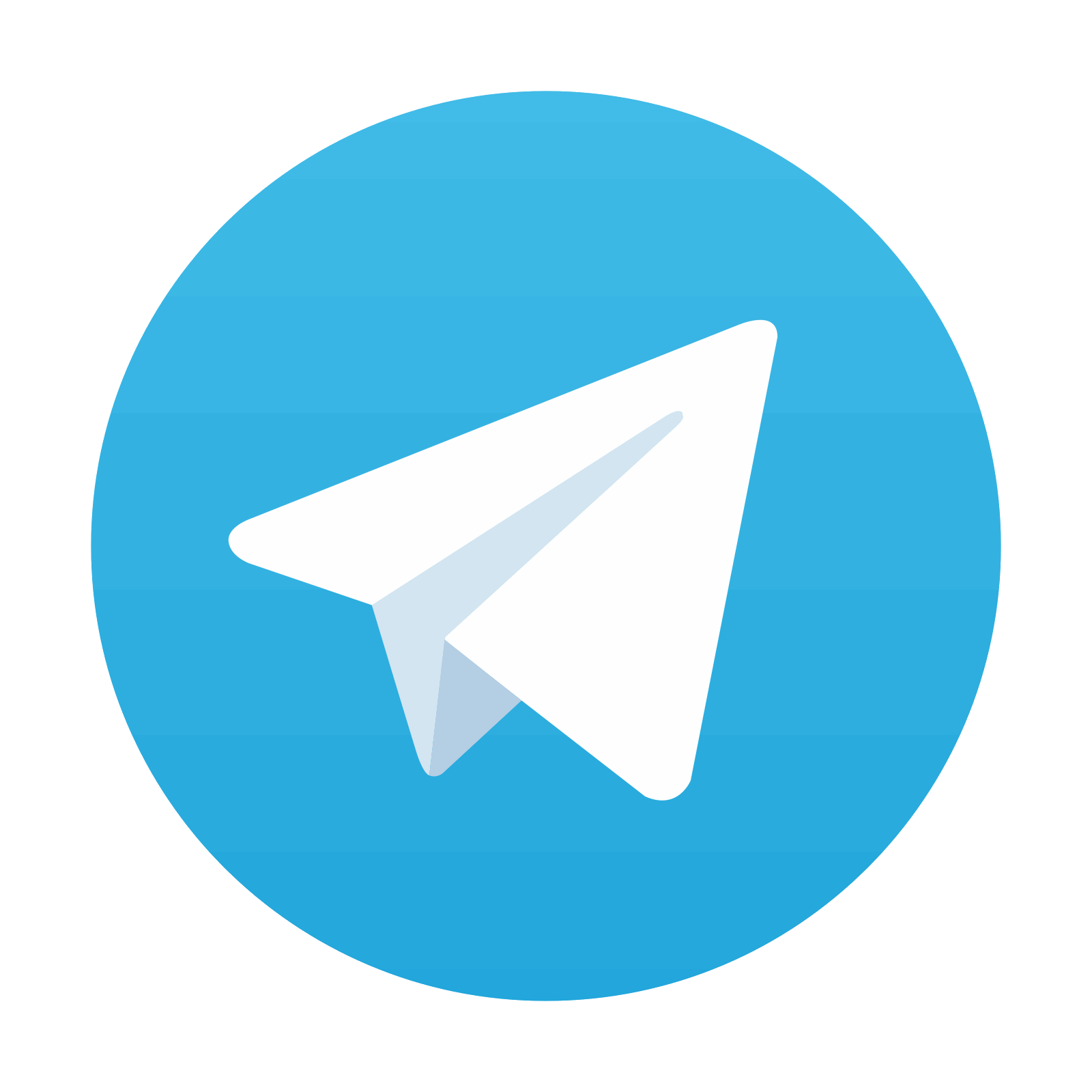
Stay updated, free articles. Join our Telegram channel

Full access? Get Clinical Tree
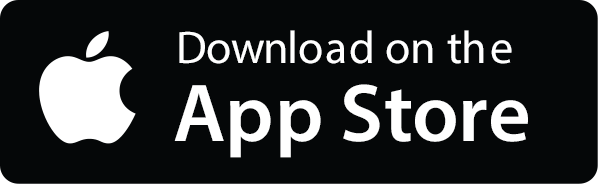
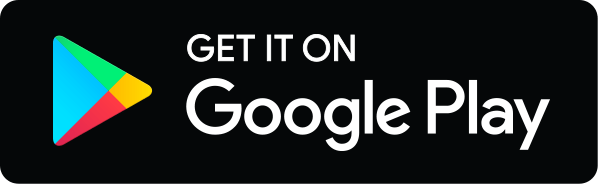