Fig. 2.1
Schematic drawing of the strategies for hair cell regeneration
2.2.1 Self-Repair
Before hair cells or spiral ganglion neurons disappear, the induction of self-repair may be a pragmatic strategy. For this purpose, there are two possible strategies. One is the promotion of survival of hair cells and subsequent reconstruction of cellular components in hair cells by spontaneous activity. This can be expressed as the protection from cell death. Several agents for promotion of survival or protection from cell death have been reported. Some of such candidates have been examined for their efficacy and safety in clinical trials [7, 8]. On the other hand, there is no specific report describing the induction of reconstruction of cellular components in damaged hair cells. In spiral ganglion neurons, reconstruction of synaptic contacts with the inner hair cells or cochlear nucleus neurons is a key issue in regeneration of cellular components. For this purpose, further investigations should be required to reveal mechanisms for maturation of functional hair cells and spiral ganglion neurons.
2.2.2 Transdifferentiation
After hair cells have gone, three different possible strategies can be applied depending on the condition of the remaining supporting cells. If sufficient numbers of healthy supporting cells still remain in the sensory epithelium, the induction of transdifferentiation of supporting cells to hair cells can be a strategy for hair cell regeneration. Hair cells and supporting cells share a common progenitor in the development. In fate determination of progenitor cells in the sensory epithelium, the Notch signaling plays a key role [9, 10]. The manipulation of Notch signaling has been used for induction of transdifferentiation of supporting cells. Transdifferentiation of supporting cells to hair cells was firstly demonstrated by means of gene transfer. Introduction of Atoh1 gene into supporting cells using adenovirus vectors induced transdifferentiation of supporting cells into hair cells [11, 12]. In general, the inhibition of Notch signaling increases the expression of Atoh1, and its activation suppresses Atoh1 expression. Next to gene transfer, pharmacological inhibition of Notch signaling was used for this purpose. Pharmacological inhibition of Notch signaling by gamma secretase inhibitors induced an increase of Atoh1 expression in neonatal cochleae, leading to excessive generation of hair cells [13]. The activity of Notch signaling weakens in the supporting cells according to maturation. In adult cochleae, virtually no expression of Notch ligands and receptors was identified in supporting cells. However, during certain duration after damage, temporal activation of Notch signaling was found even in adult cochleae [14, 15]. Topical application of gamma secretase inhibitors into cochleae resulted in hair cell induction [6]. However, at present hearing restoration by this approach is not satisfactory, and the therapeutic time window is limited.
2.2.3 Dedifferentiation
In case that insufficient numbers of supporting cells remain, transdifferentiation is not an effective strategy. Transdifferentiation of one supporting cells is equal to the loss of one supporting cells. In such a case, induction of proliferation in supporting cells is necessary. In the mammalian cochlea, cell proliferation rarely occurs after birth, while in the avian cochlea (basilar papilla) supporting cells proliferate in response to hair cell loss [16]. In the avian cochlea, both transdifferentiation of supporting cells to hair cells and proliferation of supporting cells followed by differentiation into hair cells occur [16]. To induce proliferation of supporting cells in the mammalian cochlea, the downregulation of cell cycle inhibitors is necessary. One of the major cell cycle inhibitors in mammalian supporting cells is p27, an inhibitor of cyclin-dependent kinase. Genetic deletion of p27 resulted in excessive generation of hair cells [17, 18]. Knockdown of p27 in supporting cells induced reentry of cell cycle in supporting cells, but the majority of supporting cells that had reentered cell cycle fell into apoptosis [19]. Thus, cell cycle reentry of supporting cells is not sufficient for regeneration of hair cells, suggesting that alterations in characteristics of supporting cells may be critical. Recently, challenges for induction of dedifferentiation in supporting cells have been reported [20, 21]. One possible strategy for dedifferentiation is introduction of transcription factors for generation of the iPS cell. Detailed analysis of mechanisms for alterations in characteristics of supporting cells in the avian cochlea after hair cell loss could provide useful information to induce dedifferentiation of mammalian supporting cells.
2.2.4 Cell Transplantation
Another option in case that supporting cells are severely damaged is the introduction of exogenous stem cells into the inner ear. In the early 2000s, the approach of cell transplantation for hair cell regeneration has gained considerable attention, because stem cells have been believed to accumulate in damaged sites and have the potential to repair damaged organs. However, migration of transplanted stem cells into damaged sensory epithelia of the inner ear rarely occurred [22]. In addition, the circumstance of the endolymphatic space in the inner ear is hard for the survival of transplants, because of its high concentrations of potassium. On the other hand, recent progress in research for induction of pluripotent stem cells for differentiation into inner ear cells has demonstrated that generation of sensory hair cells from pluripotent stem cells is possible. In the near future, specific guidance cues for hair cell induction from pluripotent stem cells would be discovered. As for spiral ganglion neurons, a cell transplantation approach is realistic comparing with hair cells. Recently, functional restoration of spiral ganglion neurons by transplantation of human ES cell-derived neural progenitors has been reported [5]. The iPS cells have a similar potential for regeneration of spiral ganglion neurons to ES cells [23]. However, the use of iPS cells involves the risk of tumor formation [24]. To realize cell-based therapy for regeneration of spiral ganglion cells, autologous transplants that are fully differentiated and completely eliminated undifferentiated cells must be used.
2.2.5 Technological Regeneration
As an alteration of biological approach for hair cell regeneration, an artificial cochlear epithelium has been investigated as technological regeneration. A cochlear sensory epithelium converts mechanical vibration to electrical signals. In response to mechanical stimuli, a piezoelectric material generates electricity and so could be used in place of a cochlear epithelium as a bionic cochlear epithelium. A thin membrane of a piezoelectric material framed with silicon generated electricity in response to sound stimuli after implantation into a guinea pig cochlea [25]. Problems to be resolved included insufficient power of the device to stimulate spiral ganglion neurons and limited sensitivity for sound frequencies. A combination of technological and biological approaches may be required to resolve these problems. Neurite induction from spiral ganglion neurons to the device by gene therapy might contribute to reduction of required electrical power for stimulation of spiral ganglion neurons.
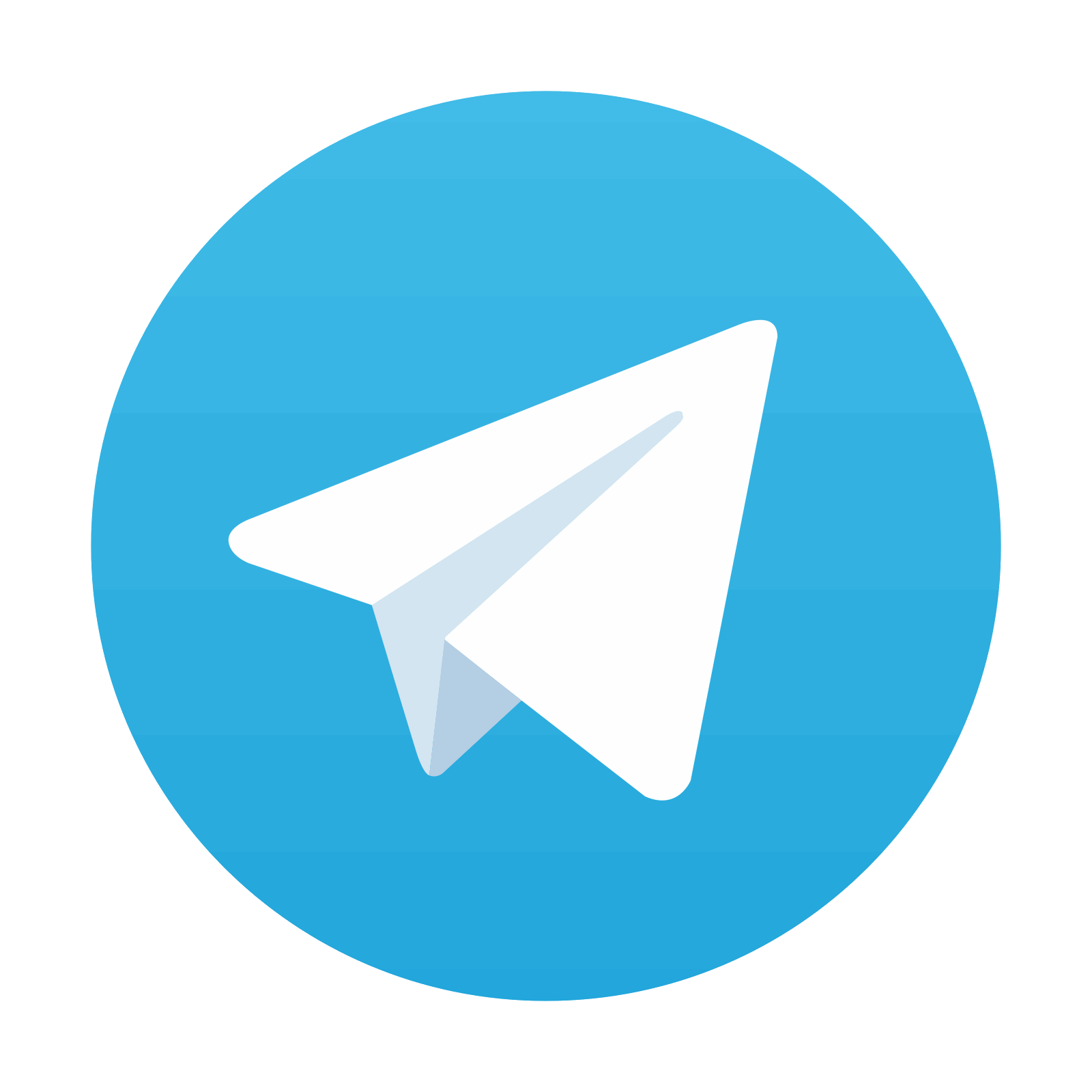
Stay updated, free articles. Join our Telegram channel

Full access? Get Clinical Tree
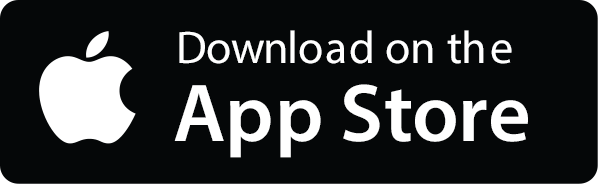
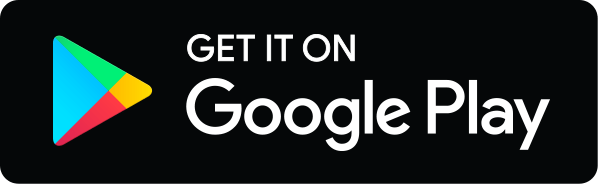