29 The Role of Stereotactic Navigation in Endoscopic Pituitary Surgery
Advances in instrumentation including specialized dissectors, powered drills and microdebriders, straight and angled rigid endoscopes, and high-definition monitors have provided a solution to the various challenges of endoscopic pituitary surgery, including its cavitary nature, dissection of bony and soft tissue structures, proximity of the target site to critical neurovascular structures, and limited vectors of motion. Inherent to endoscopic surgery is the ability to maintain robust visualization throughout the procedure. The absolute need for anatomical orientation remains an ongoing focus of development in endoscopic pituitary surgery.
Although modern endoscopes and monitors provide a magnified panoramic view of the operative field, several limitations continue to exist, the largest of which is the inability to see through bone or tissue to predict what anatomy lies just beyond the penetration of visible light. The two-dimensional view of current systems lacks the depth perception afforded by microscopic surgery. Additionally, the cavitary nature of endoscopic surgery implies that only limited perspectives are available, especially early in the course of the procedure. Anatomical structures are dissected in an anterior to posterior trajectory, in comparison to open access surgery, where circumferential anatomical exposure is possible. Finally, the separation of target sites by bony boundaries requires transgression of a given structure before successful arrival at the target is confirmed. Examples of these boundaries include the anterior face of the sphenoid sinus and the floor of the sella. Surgical experience, preoperative imaging studies, and the stability of anatomical landmarks are more than adequate to maintain orientation in the majority of patients. However, the potential for anatomical disorientation and inadvertent injury to surrounding neurovascular structures exists. Certain situations are identified as potentially higher risk, including anatomical variants, revision surgery, and complex pathology. The need for increased anatomical information is the theoretical basis for stereotactic navigation in endoscopic pituitary surgery. The triplanar radiographic correlate of the surgical field allows for an improved understanding of the relationship of a given structure to its surrounding anatomy. The radiographic information provided by neuronavigation complements the endoscopic view and has the potential to result in improved outcomes. This chapter reviews the history, technology, indications, clinical use, limitations, recent advances, and future directions of stereotactic navigation in endoscopic pituitary surgery.
History
The historical landmarks in stereotactic neuronavigation have been based on adaptation of diagnostic radiology to the operating room. The earliest neuronavigational device was described in 1908 and utilized a rigid frame for fixing the head and a system of levers and pulleys to predict the location of intracranial structures based on external landmarks of the skull.1 The application of plain radiography to stereotaxy was described by Spiegel and Wycis in the 1940s, and although conceptually similar to modern neuronavigation, this device lacked sufficient accuracy for practical use.2 The era of modern image guidance began in the 1970s with the incorporation of computed tomography (CT). The CT-based system described by Bergstrom and Greitz3 in 1976 utilized a fixed metal ring worn by the patient during both the scan and the procedure. Although this allowed for improved accuracy, it also blocked complete surgical access. The practical refinements over the ensuing decade, including semirigid articulating arms, frameless systems, and incorporation of magnetic resonance imaging (MRI)-based data sets, increased the utility of neuronavigation.4–7 Reductions in the cost and improvements in the flexibility of these devices resulted in their widespread adoption for neurosurgical procedures during the 1980s and 1990s, including for microscopic transsphenoidal surgery.8 The popularity of image-guided surgery significantly broadened with its application to endoscopic sinus surgery (ESS) in the 1990s. Similar to transcranial surgery, ESS involves surgical dissection within stable bony confines, namely the paranasal sinuses and skull base. This allows for maintenance of accuracy throughout the procedure. The use of image guidance in ESS has been described for a variety of indications including revision procedures, anatomical variations, cerebrospinal fluid leak closure, endoscopic tumor resection, endoscopic orbital surgery, and endoscopic skull base surgery.9–14 The inability of traditional image guidance to reflect intraoperative changes has led to interest in incorporation of “realtime” imaging modalities into the operating room. Multiple modalities used for intraoperative imaging have been reported over the past 50 years including x-ray fluoroscopy,15 gas cisternography,16 ultrasound,17,18 Doppler ultrasound,19 CT scan,20 and MRI.21–23 These technologies have the potential to provide critical information, including the necessary extent of resection and the presence of residual tumor. However, as explored in this chapter, the role of these technologies in endoscopic pituitary surgery is currently evolving.
Technology and Practical Use
The field of neuronavigation is, in large part, driven by a continual stream of technologic advancements. Although the specific solutions offered by the different commercially available systems vary, the basic tenets of image guidance are similar. The hardware component houses the computer workstation, a monitor for image display, and an interactive component that communicates between the surgical team and the computer (Fig. 29.1). The last varies among the systems, with examples including keyboard, mouse, and touch-screen models. The tracking device interfaces between the patient and computer and enables navigation to be performed during the surgery. Three different models of surgical tracking have been described. The earliest systems were mechanical and relied on the use of rigid and semirigid arms. The cumbersome nature and limited degrees of motion associated with these systems eventually resulted in their obsolescence. Modern systems are based on either line-of-sight or electromagnetic technology. Lineof-sight technology utilizes light-emitting diodes and infrared communication between the computer workstation and the tracking device, whereas electromagnetic technology achieves this with radiofrequency emission. The practical limitation of the infrared systems is the need to maintain an unobstructed spatial path between the tracking device and the cameras on the computer workstation. In contrast, electromagnetic systems require a direct communication between the tracking device and the computer, typically in the form of cables. An additional distinction of electromagnetic systems is the potential for interference with ferromagnetic instrumentation.
Fig. 29.1 The computer workstation, visualization screen, and infrared tracking sensors. The direct line of sight required for this type of system contrasts with the radiofrequency emission used in electromagnetic based systems. (BrainLab VectorVision2, image courtesy of BrainLab AG.)
Similar to operating systems for personal computers, those used in image guidance are continually upgraded with newer generations. Specialty-specific parameters (e.g., for neurosurgery, otolaryngology, and spine surgery) are incorporated in the workstation. Newer generations of operating systems include more refined parameters for the registration, manipulation, and display of radiographic data. The software component is the patient’s radiographic data set. Reformatting and manipulation of these images allow for the creation of triplanar views, three-dimensional (3D) models, and different window settings (e.g., soft tissue, bone). The choice of the imaging modality that is used for tracking is at the discretion of the surgeon.
The individual steps of performing image-guided surgery require coordination by the entire surgical team. The decision to perform image-guided surgery is at the discretion of the surgeon and is further explored in the surgical indications section below. The patient undergoes the appropriate imaging study. Specific protocols for these scans are utilized typically with 1-mm-thick slices around the area of the surgical field. Inclusion of fiducial markers or headsets varies depending on the system. Coordination with the imaging center is required for the correct performance of the study, as the formatting of these scans differs from traditional diagnostic studies. The images are transferred to the computer workstation and reviewed by the surgical team preoperatively to confirm that the indicated study was performed with the correct formatting and that the anatomical area of interest is well displayed. Successful transfer of images is additionally confirmed with reproduction of a 3D model of the patient’s face. Distortion of this model indicates an error either with the protocol used for the scan or the transfer of the raw data onto the computer workstation. Surgical planning including visualization of 3D reconstructions and determining the surgical trajectory can be performed at this point.
Once the patient is brought into the operating room and anesthetized, registration and calibration are performed. Registration is defined as the 3D correlation of radiographic images with the surgical anatomy. This critical step allows for accurate navigation during the procedure. The method of registration varies based on the image guidance system and may incorporate adhesive fiducial markers, disposable head set, rigid fixation in a neurosurgical pins with attachment of the reference array to the head frame, specialized rigid pin with reference array placed directly into the skull, and elastic headband. Calibration refers to the preparation of neuronavigational instruments for intraoperative tracking. The instruments available for neuronavigation were initially limited to suctions and straight probes. However, modern systems allow for tracking with the complete array of standard surgical instrumentation including dissection and powered instrumentation (e.g., microdebriders, drills). During the course of the procedure, neuronavigational tracking is performed at the discretion of the surgical team; potential applications are discussed below. The possibility of degradation in accuracy exists, especially with longer procedures, and re-registration is indicated if this occurs. Some of the currently available systems additionally perform automatic re-registration at fixed time intervals throughout the surgery.
Following the surgery, neuronavigation is billed under the Current Procedural Terminology (CPT) code 61795, Stereotactic Computer-Assisted Volumetric (Navigational) Procedure, Intracranial, Extracranial, or Spinal. It is classified as an “add-on” code and must be linked with the primary procedure codes. Additionally, adequate documentation of its use must be detailed in the operative report, including the indications for its use, downloading and verification of images, intraoperative registration, calibration, and intraoperative tracking.
Clinical Indications
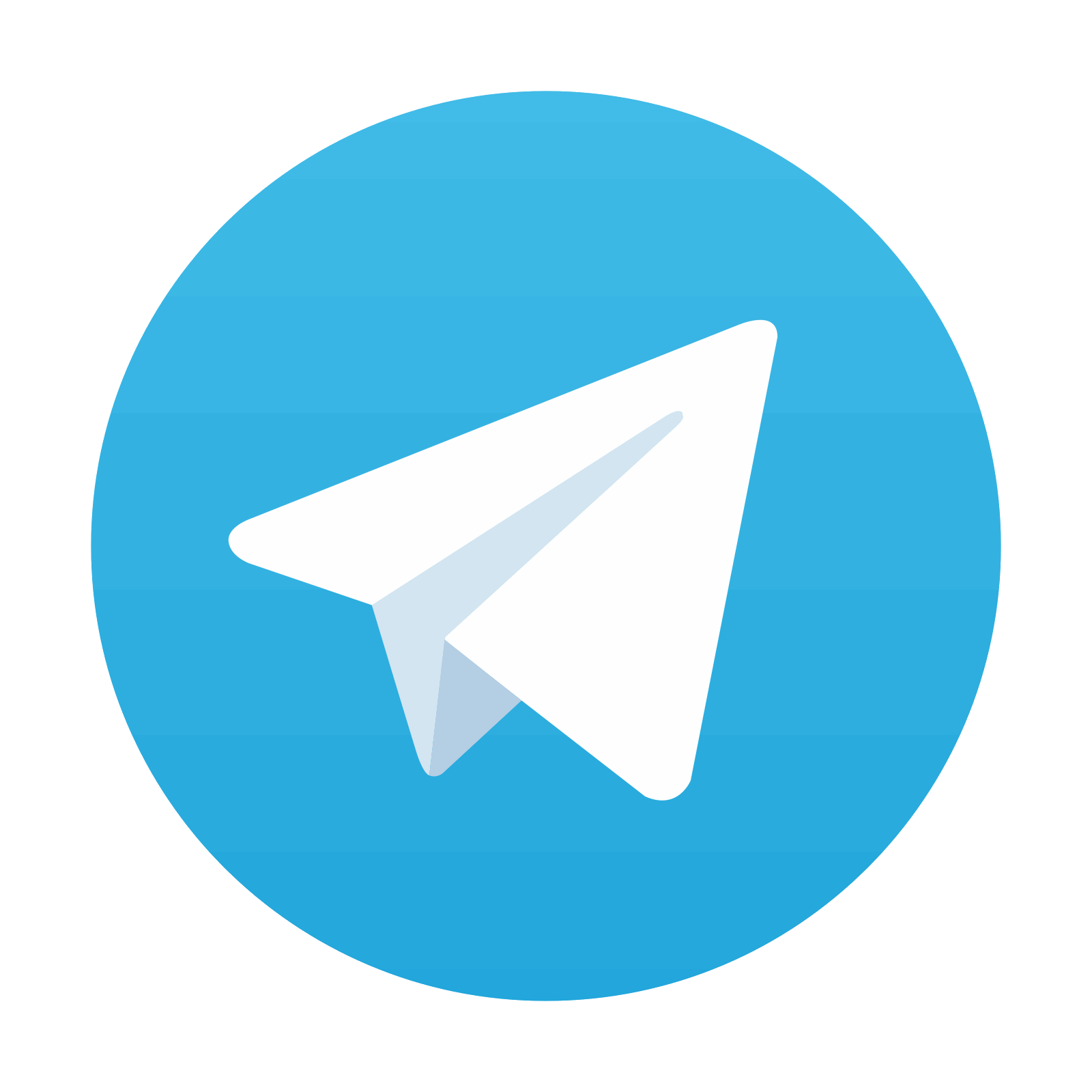
Stay updated, free articles. Join our Telegram channel

Full access? Get Clinical Tree
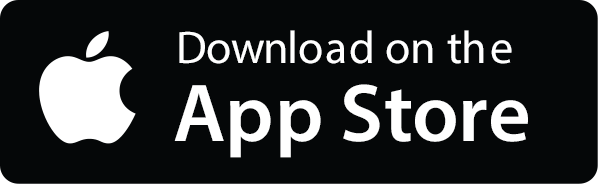
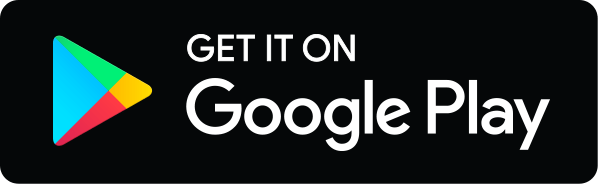