Fig. 8.1
(a) Electron microscopy of tissue eosinophil in CRS. Note the black arrow pointing to a characteristic secondary granule (transmission electron microscopy, original magnification ×7,000). (b) Electron microscopy of an eosinophil secondary granule with its characteristic, rectangle core, which is entirely made up out of MBP (transmission electron microscopy, original magnification ×55,000)
Human ECP is a basic neurotoxic protein, with antiviral and antiparasitic properties, and human EDN is a powerful neurotoxin that can severely damage myelinated neurons in experimental animals (Gleich and Adolphson 1986). EDN as well as ECP have antiviral activities and decrease the infectivity in RSV suspensions (Rosenberg and Domachowske 2001; Domachowske et al. 1998b). When purified eosinophils, EDN, or ECP was added to RSV viral suspensions, the viral titer is reduced, dependent on the ribonuclease activities of EDN and ECP (Domachowske et al. 1998a, b). Interestingly, ribonuclease A lacked this antiviral activity, suggesting that ribonuclease activity is necessary but not sufficient for the antiviral effects of EDN and ECP. Furthermore, in guinea pigs infected with parainfluenza, pretreatment with anti-IL-5 and reduction of eosinophils strikingly increased the viral content in the airways (Adamko et al. 1999), suggesting a potential role for eosinophils in viral immunity and explaining the influx of eosinophils in upper viral infections and the subsequent clinical relevant exacerbation of CRS and asthma during common colds.
EPO is a member of a mammalian peroxidase family. EPO is a central participant in generating reactive oxidants and radical species by activated eosinophils (Mitra et al. 2000). Eosinophil activation in vivo shows oxidative damage of proteins through bromination of tyrosine residues (Wu et al. 2000). Furthermore, eosinophils are a major source of nitric oxide-derived oxidants in specimens from patients with severe asthma (MacPherson et al. 2001).
Considerable evidence exists to link these eosinophil granule proteins and human diseases. For example, the concentrations of MBP in the bronchial alveolar lavage (BAL) fluids from patients with asthma and from monkeys are correlated with the severity of bronchial hyperreactivity (Gleich et al. 2000, 1993). MBP has been localized to damaged sites of bronchial epithelium in patients with asthma and chronic rhinosinusitis (Gleich 2000; Ponikau et al. 2005). Instillation of human MBP and human EPO provokes bronchoconstriction, and MBP increases airway responsiveness to inhaled methacholine (Gleich et al. 2000). Interestingly, polyglutamic acid antagonizes MBP’s ability to increase respiratory resistance and bronchial hyperreactivity in cynomolgus monkeys (Gundel et al. 1991), suggesting that the cationic nature of MBP contributes to the damage and physiological changes. In vitro, MBP acts as an antagonist for M2 muscarinic receptors. Many eosinophils localized close to nerves with extracellular MBP adhering to the nerves (Costello et al. 1997). Finally, neutralization of endogenously secreted MBP, either with a polyanionic peptide or with antibodies to MBP, can prevent antigen-induced bronchial hyperreactivity in guinea pigs (Costello et al. 1999). Marked deposition of free EDN is also observed in affected tissues from patients with eosinophilic esophagitis (EoE) (Kephart et al. 2010) (see Fig. 8.1). Deposition of EDN is reduced in certain patients with EoE who are treated with anti-IL-5 antibody (Straumann et al. 2010).
Several proinflammatory enzymes have been associated with the eosinophil (Gleich and Adolphson 1986). Arylsulfatase B is located predominantly in the small granules of the eosinophil. β-glucuronidase activity in eosinophils is about twice that in neutrophils, and exposure of eosinophils to opsonized zymosan particles releases up to 24 % of the total cellular β-glucuronidase.
8.4.2 Activation of Human Eosinophils Takes Multiple Stages
In early 1980s, increased number of unusual human eosinophils with a specific gravity <1.085 g/ml (Rothenberg et al. 1988) was reported in peripheral blood of patients with eosinophilic disorders, such as hypereosinophilic syndrome (Winqvist et al. 1982) and asthma (Fukuda et al. 1985). These eosinophils, called “hypodense eosinophils,” were highly reactive to stimuli and showed increased survival, adhesion, leukotriene synthesis, superoxide production, and antibody-dependent cytotoxicity as compared to “normodense eosinophils” (Lopez et al. 1986, 1988). Thus, eosinophils in human blood are not a homogenous population but represent various magnitudes of activation. It was found later that eosinophil exposure to activating cytokines, such as IL-3, IL-5, and GM-CSF, leads to development of the hypodense eosinophil.
IL-3, IL-5, and GM-CSF, besides being growth and maturation factors for eosinophils, stimulate several functions of mature human eosinophils. Among human peripheral blood leukocytes, eosinophils are the only cells having detectable levels of IL-5 receptors in agreement with the specific action of IL-5 on human eosinophils (Ingley and Young 1991; Migita et al. 1991). Other Th2 cytokines, such as IL-4 and IL-13, also activate eosinophils. IL-4 upregulates the binding of eosinophils to IgA (Bracke et al. 1997). IL-4 or IL-13 acts synergistically with TNF-α or IL-5 for increased expression of CD69.
8.4.3 Eosinophil Activation in Innate Immunity
Fully activated human eosinophils appear to defend against large, nonphagocytosable organisms, most notably the multicellular helminthic parasites and fungi. Some of the mechanisms used by eosinophils in host defense against these organisms may also produce detrimental effects on the host. Several lines of evidence have indicated that bacterial and/or viral infections may exacerbate allergic inflammation. Direct activation of eosinophils by microbe-derived molecules may explain the mechanism.
Importantly, eosinophils are activated by a natural cysteine protease from mite allergens, Der f 1, and release their granule proteins (Miike and Kita 2003). Eosinophils also recognize the aspartate protease activity and cysteine protease activity that are produced by fungus Alternaria alternata (Matsuwaki et al. 2009) and cockroaches (Wada et al. 2010), respectively, and they release granule proteins and cytokines. Thus, human eosinophils are equipped with machineries that recognize and respond to proteases, such as those found in microbes and at allergic response sites, resulting in active release of proinflammatory mediators.
An association between fungal exposure and asthma has been widely recognized (Bush and Prochnau 2004). Moreover, exposure to Alternaria is a risk factor for respiratory arrest in patients with asthma (O’Hollaren et al. 1991). These airborne fungi and their products may contribute to the development and exacerbation of allergic airway diseases. For example, fungal products, e.g., proteases, induce immunological and inflammatory reactions, resulting in a Th2-like cytokine response and the destruction of mucosal barrier functions (Kheradmand et al. 2002). Extracts of Alternaria potently induces eosinophil degranulation (Inoue et al. 2005). Alternaria also strongly induces other activation events in eosinophils, including increases in intracellular calcium concentration, cell surface expression of CD63 and CD11b, and production of IL-8. Interestingly, Alternaria does induce neutrophil activation, suggesting specificity for fungal species and cell type. In addition, when human eosinophils are exposed to live Alternaria alternata fungus, eosinophils release their cytotoxic granule proteins into the extracellular milieu and onto the surface of fungal organisms and kill the fungus in a contact-dependent manner (Yoon et al. 2008). Eosinophils do not express common fungus receptors, such as dectin-1, but use their versatile β2 integrin molecule, CD11b (see below for more details), to recognize and to adhere to a major cell wall component, β-glucan.
The role of IgE in mediating eosinophil activation is controversial. Eosinophils isolated from patients with eosinophilia degranulated in response to anti-IgE antibody or IgE-coated parasites (Kita et al. 2003; Moqbel et al. 1990). Eosinophils can potentially express three types of IgE receptors, the low-affinity IgE receptor, lectin-type IgE-binding molecule (Truong et al. 1993), and high-affinity IgE receptor. It has been claimed that the high-affinity IgE receptor, FcεRI, is present on eosinophils from patients with eosinophilia and that various functions of eosinophils, including degranulation and parasite cytotoxicity, are mediated through this receptor (Gounni et al. 1994). On the other hand, the number of high-affinity receptors expressed on the surfaces of eosinophils from patients with allergic diseases or airway eosinophilia was minimal or undetectable (Seminario et al. 1999), and the ligation of IgE FcεRI receptor did not result in detectable eosinophil degranulation (Kita et al. 1999).
8.5 Differences in the Eosinophilic Inflammation Between Allergic Rhinitis and Chronic Rhinosinusitis
The events and the pathophysiology of allergic rhinitis have been well understood. The inhalation of an allergen where an individual has produced corresponding, circulating IgE antibodies will cause (within 10 min) a cross-link of the IgE FcεRI receptors situated on the mast cells. This will result in an immediate degranulation and histamine release from the mast cells, resulting in histamine-mediated symptoms of sneezing, clear anterior rhinorrhea, and nasal obstruction. About 2–8 h after antigen challenge, eosinophils enter the nasal tissue as part of the so-called late-phase allergic reaction (together with further mast cells, B and T lymphocytes), leading to further nasal obstruction.
In contrast to allergic rhinitis, the early-phase immediate reaction is missing in CRS, explaining the lack of allergic rhinitis specific, histamine-related symptoms (sneezing, anterior clear rhinorrhea). Another clinical distinction between CRS and AR is that CRS can occur with or without nasal polyps, while AR never leads to nasal polyposis. While some investigators follow the notion that due to different severities in the cytokine pattern, CRS with and without nasal polyps should be viewed as two different entities, while others see it as a different spectrum of disease, with inflammatory mucosal thickening on one side of the spectrum, to gross nasal polyps on the other side. The severity of the inflammation can be easily overlooked if patients are given systemic steroids or other anti-inflammatory medication before harvesting the tissue for examination. This distinctive eosinophilic inflammation is also very heterogeneous (i.e., without eosinophilic infiltration in one area of a nasal mucosal tissue specimen, but with intense eosinophilic infiltration in another area of the same specimen) (Hamilos et al. 1998). Thus, reports in which only single biopsies are examined and in which it was unclear whether patients had received steroids before the biopsies were taken need to be interpreted carefully regarding the intensity of the eosinophilic infiltrate.
The eosinophilic inflammation in CRS occurs independently of an IgE-mediated inflammation, as evident by the fact that more than 50 % of CRS patients have no detectable IgE-mediated allergies. This in return suggests nonallergic mechanism driving recruitment, migration activation, and degranulation. Indeed, very different mechanisms have been identified.
Central to the migration of eosinophils from the vasculature into the tissue is the expression of vascular cell adhesion molecule-1 (VCAM-1), which has been identified in the vascular endothelium in CRS patients (Rains and Mineck 2003). This expression occurred independent of any IgE-mediated allergy and explains the presence of eosinophils in allergic as well as nonallergic patients with CRS (Rains and Mineck 2003). VCAM-1 is known to specifically bind to the VLA-4 (very late-appearing antigen-4) on eosinophils, thus causing selective adhesion and migration of eosinophils from the vasculature to the sinus and nasal tissue (Rains and Mineck 2003). VCAM-1 expression is induced via either IL-4 or IL-13, which shares the same receptor on the endothelial cells. IL-4 is present and IL-13 is absent in allergic rhinitis, which is in contrast to nonallergic CRS, where only IL-13 is present in the tissue, but IL-4 is absent. In patients with CRS and allergies, both IL-4 and IL-13 are present. This cytokine pattern indicates that eosinophils are recruited via two distinct cytokines, IL-4 in AR and IL-13 in CRS; however, both diseases can coexist as CRS with allergies (comorbidity), with both Il-4 and Il-13 present.
Significantly elevated levels of IL-5, the key cytokine that mediates eosinophil differentiation, survival, and activation, are present in tissue specimens of CRS patients and AR patients, and not in those of healthy controls (Ricchetti et al. 2002; Salo et al. 2005; Sanchez-Segura et al. 1998, 2000; Shin et al. 2004; Simon et al. 1997). A majority of the IL-5 staining cells are lymphocytes (68 %), followed by eosinophils (18 %) and mast cells (14 %) (Taylor et al. 2002). The exact combination of source cells for IL-5 in AR is not known. While many different allergens can lead to the release of IL-5 in AR via the IgE-mediated pathway in AR, a trigger for the nonallergic production of IL-5 in CRS was unknown.
Recently, Shin et al. (2004) demonstrated that certain molds induced the elevated production of IL-5 in isolated peripheral blood mononuclear cells (PBMCs), which contained lymphocytes and other cells that can serve as antigen-presenting cells from 16 out of 18 CRS patients stimulated with Alternaria antigens. More importantly, PBMCs from none of the 15 healthy controls did produce elevated IL-5 levels (Shin et al. 2004). PBMCs from allergic and nonallergic CRS patients produced similar amounts of IL-5, indicating that this reaction is independent from an IgE-mediated allergic reaction (Shin et al. 2004). In addition, PBMCs from CRS patients stimulated with either Cladosporium (6/18) or Aspergillus (4/18) antigens also show increased production of IL-5; no response is seen with Penicillium antigen (Shin et al. 2004).
But not only IL-5 was produced by the CRS patients’ immune cells in response to Alternaria. The mold also induced the release of large amounts of IL-13 in all the CRS patients studied, the cytokine triggering the recruitment of eosinophils from the vasculature into the tissue in CRS. Again, not of the healthy controls were producing any detectable IL-13.
Furthermore, production of interferon-γ (IFN-γ), a Th1 cytokine which facilitates destruction of parasites by eosinophils, was 5.5 times higher in PBMCs from CRS patients stimulated with Alternaria antigen compared with production by healthy control PBMCs (Shin et al. 2004). When nasal secretions from nine healthy controls and nine CRS patients were examined, there were no differences in their levels of total Alternaria proteins (Shin et al. 2004), indicating that both groups had similar levels of Alternaria in their nasal mucus. This study was important since it was the first to demonstrate a nonallergic pathway in CRS leading to the production of the crucial cytokines for the eosinophilic inflammation. In addition, it also showed a specific trigger for the cytokine production, a common mold being present in nose of every person tested.
Recently it was shown that CRS patients exhibit severely damaged epithelium and thickened basal membrane, features of airway remodeling seen as also seen in asthma, which is in contrast to their absence in AR (Fig. 8.2a, b). It has been demonstrated that eosinophilic MBP is capable to produce those changes, and indeed MBP has been localized with the epithelial damages found in CRS (Gosepath et al. 2004; Hamilos et al. 1995). Interestingly, toxic MBP levels have measured in CRS, but free MBP could not be measured in AR mucus, explaining the damage in CRS, and its absence in AR. This suggest that MBP is released in the mucus in CRS, but not in AR.
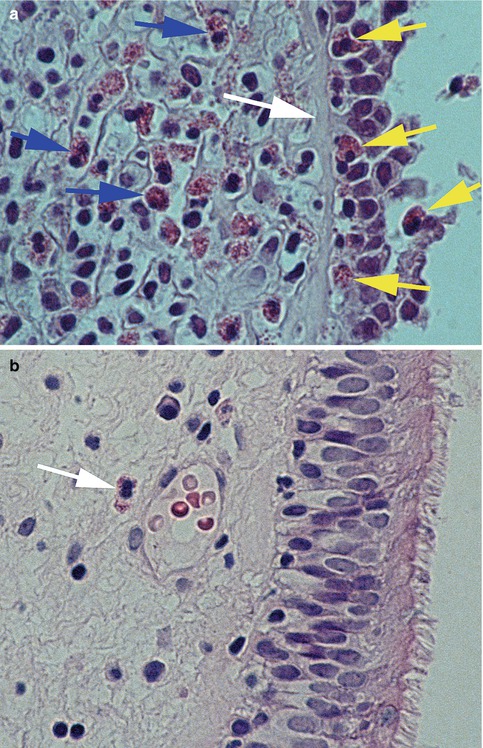
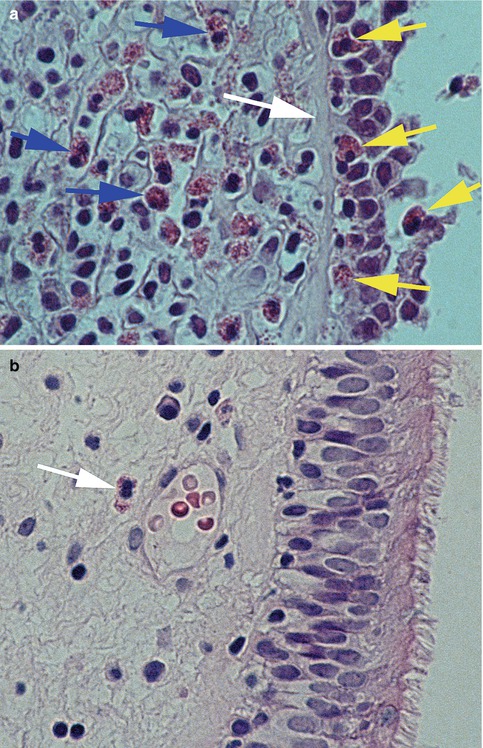
Fig. 8.2
(a) Tissue from CRS patient shows intact tissue eosinophils (blue arrows) and eosinophils traveling through the severely damaged epithelium (yellow arrows). Note the missing upper layers of epithelial cells and missing cilia, suggesting that the damage is inflicted from the luminal side. The white arrow highlights the thickened basal membrane (original magnification ×1,000, HE). (b)Tissue from AR patient reveals intact epithelium including cilia and scattered eosinophils (white arrow). Note also the absence of basal membrane thickening (original magnification ×400, hematoxylin and eosin)
Two prospectively designed histologic studies of tissue and mucus obtained during CRS surgery used extra caution to preserve the mucus. While eosinophils were intact in the tissue and in the epithelium, eosinophilic-rich mucus with clusters of aggregated eosinophils was found in 96 % (97/101) and 94 % (35/37) of consecutive CRS patients (Inoue et al. 2005; Kita et al. 2003). Another study demonstrated that eosinophils released their toxic MBP in the mucus within these clusters, and not in the tissue (Hamilos et al. 1996). Estimated concentrations of MBP within the clusters, based on digital analysis of the intensity of the MBP staining, were as high as 2 mM and exceeded those capable of mediating epithelial damage. Overall, the clusters of eosinophils and intense eosinophil degranulation in the mucus suggest that eosinophils merely travel through the CRS tissue to the mucus (Fig. 8.3a, b).
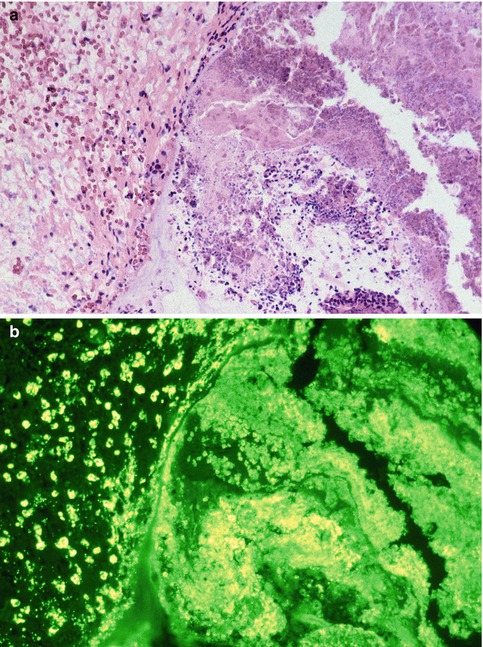
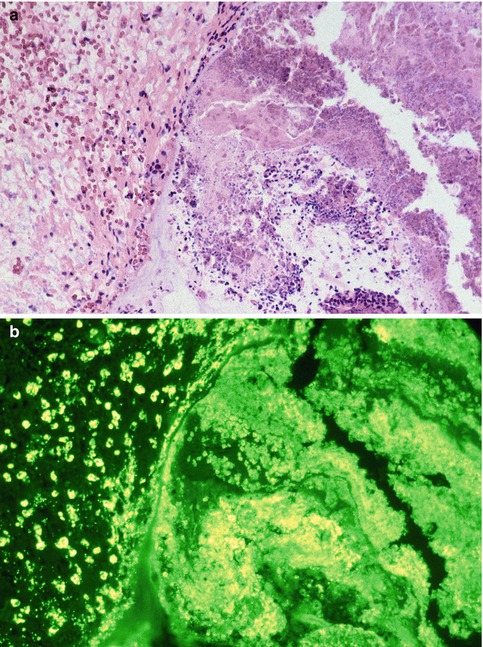
Fig. 8.3
(a) CRS tissue and attached eosinophilic mucin shows massive eosinophilic migration of eosinophils from the tissue (left side of the image) into the mucus (right side of the image). The mucus contains large sheets (clusters) of eosinophils and eosinophilic debris (original magnification ×400, HE). (b) Serial section of 2a with immunofluorescent staining with an antibody against MBP reveals intact eosinophils in the tissue (left side of the image) and free eosinophil granules. In contrast, once the eosinophils have reached the mucus, MBP is diffusely release in toxic concentrations. Note that MBP staining reaches brightness in the mucus exceeding the one inside the intact tissue eosinophils, indicating continues deposition of free MBP into the same eosinophilic clusters in the mucus (original magnification ×400, anti-MBP)
These in vivo observations explain the patterns of damage in CRS, where only the outer layers of tissue are damaged (Fig. 8.2a, b), suggesting that the damage to the epithelium is inflicted from the outside (luminal side). This epithelial damage may predispose CRS patients to be susceptible for the secondary bacterial infections, leading to acute exacerbations, which are observed clinically and absent in AR. Because bacteria typically elicit a neutrophilic inflammation, these acute exacerbations of CRS are presumed to be of bacterial origin. However, the underlying eosinophilic inflammation that predominates in CRS is unlikely to be caused by bacterial infection, suggesting a nonbacterial etiologic mechanism for CRS.
In a recent study, eosinophils from healthy people that were incubated with Alternaria and Penicillium antigens released significant amounts of eosinophil-derived neurotoxin (EDN), a marker of eosinophil degranulation (Weschta et al. 2004). When eosinophils from patients with asthma or allergies were used, they even released about 70 % more EDN compared to the healthy controls. The fraction from Alternaria alternata, which induced the degranulation, had a molecular weight of ≈60 kDa, was highly heat labile, and worked protease dependant through a G protein-coupled receptor, identified as β2 integrin of the CD11b receptor (Weschta et al. 2004). Other fungal antigens, including Aspergillus, Cladosporium, and Candida, did not induce eosinophil degranulation, nor did neutrophils respond to Alternaria extracts, suggesting the presence of a fungal species and cell-type-specific novel innate immune response to certain fungi in human. Thus, both innate and acquired immune responses to environmental fungi such as Alternaria (independent of IgE antibodies to Alternaria) may increase production of the cytokines and provide cellular activation signals necessary for the robust eosinophilic inflammation in CRS patients.
8.6 Summary and Future Directions
Eosinophils fulfill distinctive and different function in CRS versus AR, although frequently overlapping clinically. Those differences presumably result in two different pathophysiological mechanisms, mainly distinguishable through the clustering of eosinophils and the subsequent release of the eosinophil-specific toxic major basic protein (MBP) into the mucus in CRS. In contrast, in allergic rhinitis, the eosinophils appear to follow more a process of a controlled cell death, without the release of toxic major basic protein (MBP) and without the subsequent epithelial damage. This difference in the degranulation patterns explains the different clinical and pathophysiological presentation between CRS and AR. Understanding the details of those mechanisms and the eosinophil’s function is needed to further improve the care of patients suffering from these two eosinophilic, inflammatory diseases in rhinology.
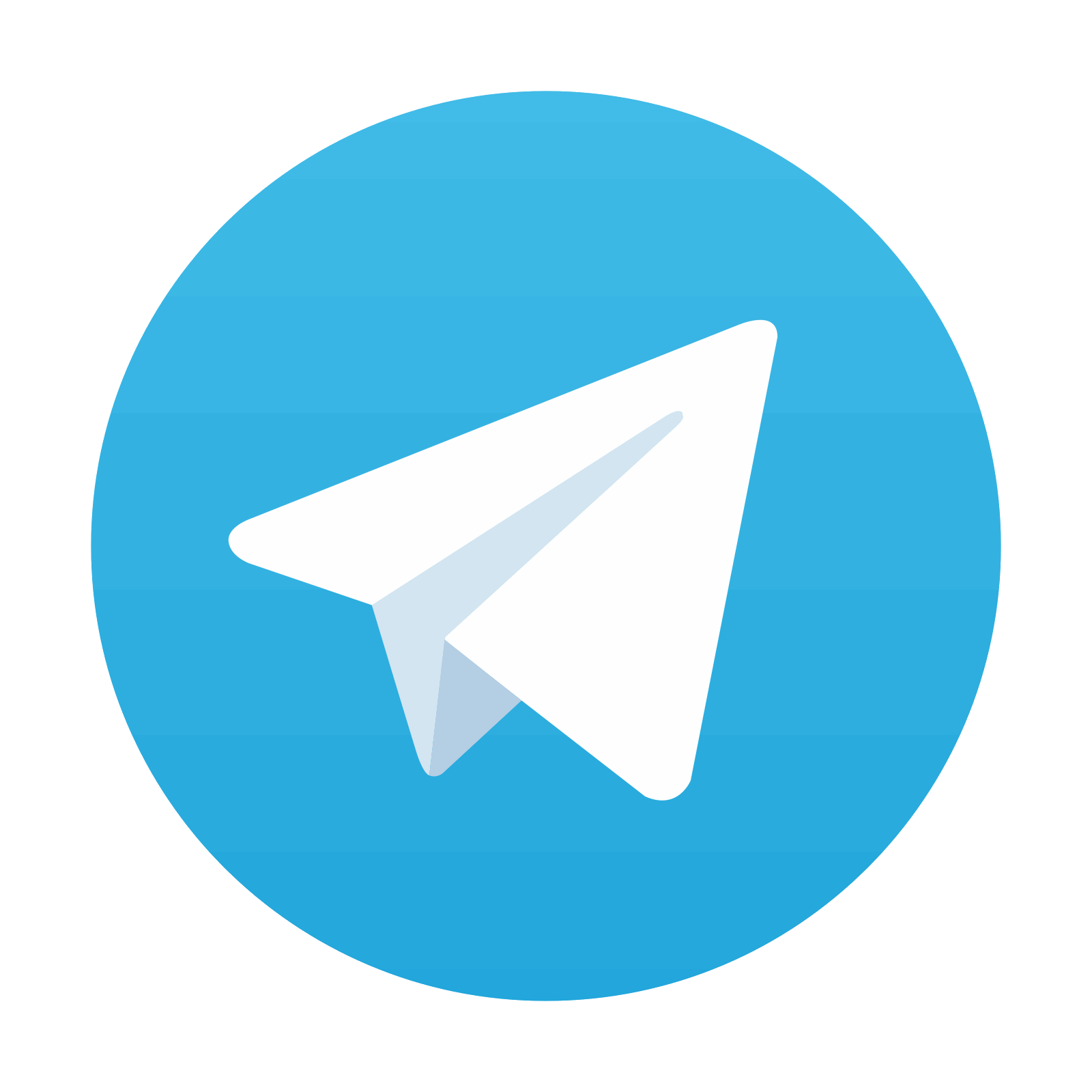
Stay updated, free articles. Join our Telegram channel

Full access? Get Clinical Tree
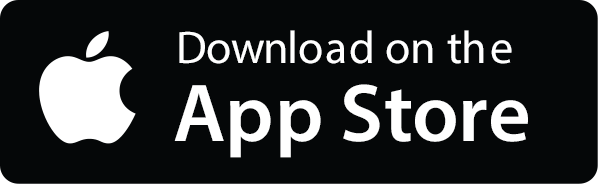
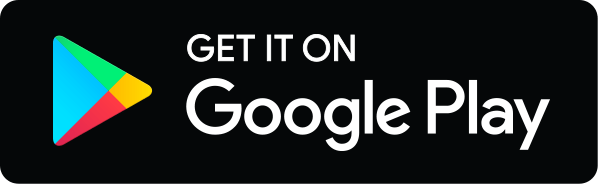