(1)
University of Sydney, Sydney, Australia
Structure and Development
Overview
The retina is a highly specialized neural tissue that converts light into neural signal.
It lines the inner surface of the globe and is separated from the sclera by the choroid.
Light is focused by the ocular media onto retinal photoreceptors (rods and cones).
Light induces a chemical change in photoreceptor cell photopigment leading to a change in cell membrane potential.
This results in neural signal conveyed by retinal interneurons to reach the brain via the optic nerve.
Embryogenesis and Development (Fig. 8.1)
The eye develops from an outgrowth of the developing neural tube, the optic vesicle (a) [1].
Invagination of the optic vesicle results in an optic cup with an inner and outer wall (b, c).
The developing outer wall becomes a single layer of RPE cells.
Multipotent retinal progenitor cells in the inner wall proliferate into retinal neuroblastic layers.
By further proliferation, migration, maturation, and selective apoptosis, these give rise to all retinal cell types in a highly organized, layered structure characteristic of the neural retina [2–4].
Cellular differentiation occurs sequentially from inner to outer layers, beginning with ganglion cells [3, 5].
Foveal development is incomplete at birth and continues into childhood [6].
Foveal cone density is significantly lower in newborns than in adults [7].
Organization of the Neural Retina (Table 8.1, Fig. 8.2)
Table 8.1
Layers of the neural retina (from outermost to innermost)
Retinal layer | Cellular substrate |
---|---|
Photoreceptor layer (Ph) | The inner and outer photoreceptor cell segments |
Outer limiting membrane (OLM) | Made of zonulae adherens between Müller cells and photoreceptor inner segments |
Outer nuclear layer (ONL) | The photoreceptor cell bodies |
Henle’s fiber layer (HL) | Photoreceptor axons |
Outer plexiform layer (OPL) | Synapses between photoreceptor, bipolar, and horizontal cells |
Inner nuclear layer (INL) | Horizontal, bipolar, and amacrine cell bodies; Müller cell nuclei |
Inner plexiform layer (IPL) | Synapses between bipolar, amacrine, and ganglion cells |
Ganglion cell layer (GCL) | Ganglion cells and displaced amacrine cells |
Nerve fiber layer (NFL) | Ganglion cell axons traveling towards the optic nerve |
Internal limiting membrane (ILM) | Formed by Müller cell endfeet |
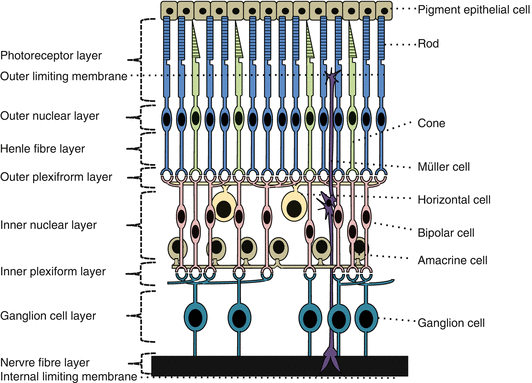
Fig. 8.2
The neural retina and retinal pigment epithelium, from outermost (retinal pigment epithelium) to innermost (internal limiting membrane) (Based on Dyer and Cepko [12])
The neural retina is organized into ten layers.
Retinal neurons consist of photoreceptor cells (outermost); horizontal, bipolar, interplexiform, amacrine cells; and ganglion cells (innermost) [9, 10].
Neural signals are passed from cones onto bipolar cells and then onto ganglion cells.
From rods the signals are passed onto rod bipolar cells and then indirectly to ganglion cells via amacrine cells.
Ganglion cell axons travel in the retinal nerve fiber layer towards the optic disc and pass uninterrupted through the optic nerve to central nervous system targets.
Retinal neurons are mainly supported by radial glial cells called Müller cells [11].
Macula Lutea (Fig. 8.3)
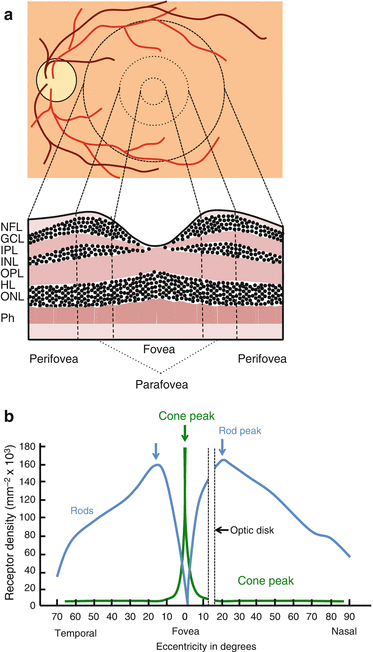
Fig. 8.3
The macula. (a) The zones of the macula (fovea, parafovea, and perifovea) and (b) rod and cone density as a function of retinal eccentricity [20]
1.
The fovea centralis
The fovea centralis is a small depression at the central macula that provides highest visual acuity.
The depression is due to centrifugal displacement of inner retinal cells to maximize image clarity [15].
Vessels are absent from the central fovea (foveal avascular zone) to minimize light scattering [16].
The central fovea relies on diffusion from the choroid for O2 and metabolic supply.
The fovea has no rods or blue-sensitive cones. Foveal cones are elongated and densely packed [13].
2.
The parafovea
The fovea is surrounded by the parafovea which is in turn surrounded by perifovea.
With increasing eccentricity the density of cones decreases while the density of rods rises to peak at approximately 200 off fixation [17, 18].
The parafovea contains the thickest GCL, IPL, and INL layers in the retina; these process signals from foveal and parafoveal photoreceptors [19].
The Henle’s fiber layer is formed by the axons of the photoreceptors and is especially prominent in the parafovea.
Photoreceptor Cells
Photoreceptor cells convert light into neural signal by phototransduction.
Photoreceptor cells consist of an outer segment, inner segment, and cell body (Fig. 8.4a).
Fig. 8.4
(a) The photoreceptor cell (structure), (b) the rod spherule and cone pedicle, and (c) the dark current
Two classes of photoreceptor cell, rods and cones, have distinct functions, morphology and distribution (Table 8.2).
Rods
Cones
Function
Scotopic vision (very dim light)
Photopic vision (bright light)
Color sensitivity
No
Yes
Number in retina
92 million
5 million
Location
Absent at fovea
Maximal density at 20° off fixation
Predominant photoreceptor cell type at the fovea
Found throughout the retina (most are non-foveal)
Pigments
All have rhodopsin
Peak sensitivity 500 nm
3 types of cones based on distinct pigments and spectral sensitivity peaks:
• Blue (420 nm)
• Green (531 nm)
• Red (563 nm)
Outer Segment
Cone outer segments (OS) are conical with a tapered end; rod outer segments are non-tapered [26].
The outer segments consist of 600–1000 disks made of bilayered lipid membrane.
The disks are stacked on each other, connected by microfilaments [27].
In cones the disks are invaginations of the lipid membrane [28].
In rods the disks are separate structures connected to the surrounding plasma membrane [29].
The protein peripherin/RDS maintains disk stability and possibly plasma membrane adhesion [30].
The disks are continually formed at the OS/IS junction and sloughed at the apex; the lipid debris is phagocytosed by RPE cells (see Chap. 9, The Retinal Pigment Epithelium) [29, 31].
Photopigments and enzymes involved in phototransduction including rhodopsin, transducin, and phosphodiesterase (PDE) are imbedded in the disk membranes [32].
The structure and composition of membrane lipids play an important role in maintaining optimal enzyme position for phototransduction [33].
Inner Segment
Its membrane contains cationic channels and Na + /K + ATPase pumps that restore resting membrane potential after light-induced hyperpolarization [35].
It synthesizes components for OS renewal including bilayered lipid membranes and molecules necessary for phototransduction [36].
It is connected to the OS via a thin connecting cilium [37].
Cell Body
The cell body contains the cell nucleus [38].
Apically it sends a synaptic projection (spherule in rods or pedicle in cones) into the OPL.
Synaptic Terminals (Fig. 8.4b)
1.
The rod spherule
The rod spherule is sphere shaped and contains mitochondria, glutamate-containing vesicles, and synaptic ribbons positioned close to the presynaptic membrane [39].
The glutamate-containing vesicles fuse to either side of the ribbon prior to release.
2.
3.
Inter-photoreceptor junctions
Rod-rod and cone-cone coupling are stronger than rod-cone coupling [9].
Membrane Potential (Fig. 8.4c)
1.
The dark current
In the dark, light-sensitive membrane cation nucleotide-gated (CNG) channels are kept open by cyclic guanosine monophosphate (cGMP) [45].
These maintain a resting dark current: Na+ and Ca2+ enter the OS, while K+ leaks out from the IS (Fig. 8.3c) [46].
This causes the membrane to be depolarized (near −40 mV) [47].
This results in release of glutamate, an excitatory neurotransmitter, from the synaptic terminal [48].
2.
Membrane response to light
3.
Differences between cone and rod membrane response to light
4.
Influences on membrane potential
Background light attenuates the response to a flash (photoadaptation).
IS K+ and Ca2+ inward conductances return membrane potential to resting value after hyperpolarization, maintaining a dynamic range of neurotransmitter release [35, 55].
Cell-cell coupling via gap junctions and synaptic negative feedback via horizontal cell inhibition allow neighboring photoreceptors to influence one another [43].
The Phototransduction Cascade (Fig. 8.5)
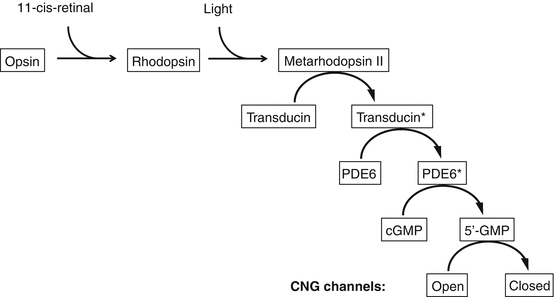
Fig. 8.5
The phototransduction cascade (*implies activated state)
Phototransduction is a series of biochemical events within the photoreceptor that leads from photon capture to membrane hyperpolarization and slowing of glutamate release [56, 57].
Phototransduction is fast, occurring within several milliseconds of light signal [58].
It occurs in the OS disk membranes where rhodopsin and phototransduction enzymes are found [32].
The process is similar in rods and cone; however, cones have different spectral sensitivities and faster biochemical responses than rods [49, 61].
1.
Activation
Rhodopsin consists of a protein (opsin) and a chromophore, 11-cis-retinal (vitamin A derivative).
This conformational change results in activation of rhodopsin into metarhodopsin II [63].
Metarhodopsin II moves freely in the lipid bilayer and activates several hundred transducin (G-protein) molecules, amplifying the signal. This is fuelled by guanosine triphosphate (GTP) [64].
Transducin binds to and activates phosphodiesterase 6 (PDE6) in a 1:1 molecular ratio [65].
Each activated PDE6 enzyme hydrolyses several hundred molecules cGMP to 5′-GMP providing a second step of amplification [58, 66].
2.
Recovery
Activated rhodopsin is rapidly inactivated by phosphorylation (rhodopsin kinase) and then by arrestin [67, 68]. Once inactivated it releases all-trans-retinal [63].
Lowered intracellular Ca2+ levels results in activation of guanylyl cyclase-activating proteins [69]. These stimulate guanylyl cyclase, reforming cGMP and restoring the cationic dark current.
Photoadaptation in Rods and Cones (See Chap. 21, Luminance Range for Vision)
Light adaptation allows the visual system to function effectively over a wide range of intensities [25].
Photoreceptors require high sensitivity at low light levels without saturating at higher light levels.
This is achieved by reduced photoreceptor light sensitivity as background light level rises [72].
1.
Membrane potential responses
With increased background light intensity, the photoreceptor membrane potential response to an incremental flash becomes faster, reduced in magnitude with a faster recovery [73].
In rods CNG channels are completely closed at moderate background intensities; the dark current becomes suppressed and the cell’s response to light saturated.
Cones maintain sensitivity over a much wider range of background illumination levels [52].
2.
Biochemical basis of photoadaptation
Light adaptation is primarily due to a marked acceleration of recovery steps in phototransduction.
Several mechanisms exist:
(i)
(ii)
(iii)
PDE6 activity increases in steady illumination, leading to a rapid reduction in cGMP levels. This allows more rapid photoadaptation and reduces peak hyperpolarization amplitude [81].
Inner Retinal Circuitry
Key Concepts
1.
Rod and cone pathways
Retinal neural channels are broadly grouped into rod and cone pathways that include all retinal neural cell types.
The rod pathway is tuned to scotopic visual information. It is sensitive at low levels of illumination and does not convey color [22].
The cone pathway(s) conveys photopic information. It is color sensitive and highly discriminative for fine acuity, requiring moderate to high illumination levels [21].
2.
Parallel processing
Parallel processing of visual information provides simultaneous analysis of various visual characteristics (e.g., line, shape, color, movement, and texture) [83].
3.
Convergence
4.
ON/OFF channels
These convey the appearance and disappearance of light, respectively.
Rods primarily contribute to the ON channels; their contribution to the OFF channels is indirect through influence of cone channels.
The ON/OFF division is preserved from the level of bipolar cells to ganglion cells [89].
5.
Center-surround organization (Fig. 8.6)
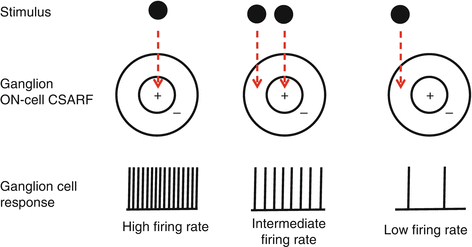
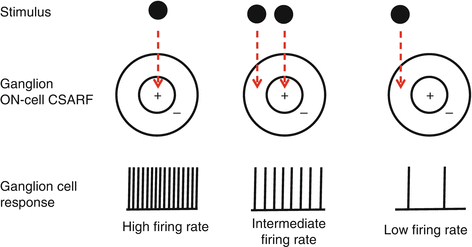
Fig. 8.6
Center-surround receptive fields
(i)
Center-surround antagonistic receptive fields
Many retinal neurons have center-surround antagonistic receptive fields (CSARFs).
CSARFs efficiently detect edges, changes in contrast, color opponency, and movement [90].
CSARFs rely on contrasts provided by ON/OFF channels; e.g., for ON-center CSARFs, ON neurons stimulate the center, while surround inhibition is provided by laterally displaced OFF neurons [87, 91].
The contrast is enhanced by lateral inhibition mediated by horizontal and amacrine cells.
(ii)
Double-opponent cells
Some bipolar and ganglion cells are double-opponent cells that convey color information [92].
For example, the central receptive field depolarizes to red and hyperpolarizes to green, while the surround depolarizes to green and hyperpolarizes to red.
6.
Temporally distinct responses
The ON/OFF functional subdivision allows temporally distinct responses to be coded [93].
Certain amacrine and ganglion cells are sensitive to transient light signal resulting in rapid sequential stimulation of ON and OFF channels [94].
This array of neurons allows coding of the duration of the stimulus.
Temporally distinct inputs provide detection of motion by motion-sensitive ganglion cells [95].
Neurotransmitters and Receptors
Nearly all neuropeptides or neuroactive substances found in the brain are also found in the retina.
Those found in the retina include:
1.
Glutamate
Glutamate is the predominant excitatory neurotransmitter within the retina.
It is used by photoreceptors, bipolar cells, and most ganglion cells [96].
Three families of ionotropic receptors exist: AMPA, kainate, and NMDA receptors [98].
Differential expression of ionotropic (AMPA/KA) and metabotropic glutamate (mGluR) receptors gives rise to the OFF and ON bipolar cells, respectively [99].
(i)
Ionotropic receptors
Ionotropic receptor activation results in increased cationic permeability, increasing inward Na+ and Ca2+ currents resulting in membrane depolarization.
These are sign conserving, maintaining the polarity of the presynaptic input [98].
(ii)
Metabotropic receptors
Metabotropic glutamate receptor activation involves a secondary biochemical cascade leading to the modification of proteins, for example, ionic channels [99].
In ON bipolar cells, mGluR6 receptors are sign inverting resulting in reversal of polarity of the presynaptic input.
2.
GABA and glycine
Gamma-aminobutyric acid (GABA) and glycine are the predominant inhibitory neurotransmitters.
GABA is used by horizontal and wide-field amacrine cells, whereas glycine is used by small-field amacrine cells [100].
3.
Dopamine
On release dopamine traverses through the retina targeting every retinal cell type [103].
Dopaminergic activation reduces cell-cell coupling of photoreceptors, horizontal, amacrine, and ganglion cells and increases transmission speeds.
4.
Acetylcholine
Acetylcholine is a fast excitatory neurotransmitter released by starburst amacrine cells in addition to GABA that targets directionally sensitive ganglion cells [106].
5.
Nitric oxide (NO)
NO is a rapidly diffusing gas that is produced by a variety of retinal neural cells [107].
Synthesis by NO synthase is activated by high intracellular Ca2+ levels [108].
NO increases intracellular cGMP, which reduces cell-cell coupling.
Like dopaminergic activation this facilitates retinal light adaptation [109].
Horizontal Cells
Horizontal cells (HCs) have long branching dendritic processes that form interconnections between photoreceptor cells and bipolar cells.
Like ganglion cells, HC dendritic field increases with retinal eccentricity [112].
HCs have gap junctions with each other permitting electrical coupling [113].
HCs use the inhibitory neurotransmitter GABA.
1.
Function
HCs provide inhibitory feedback to photoreceptor cells and inhibitory feedforward to bipolar cells, influencing signal transmission at the rod spherule and cone pedicle [112, 114].
HCs may be involved in lateral inhibition of surrounding photoreceptors, important for CSARFs in bipolar cells and subsequent ganglion cells.
2.
3.
Electrophysiology (Fig. 8.7)
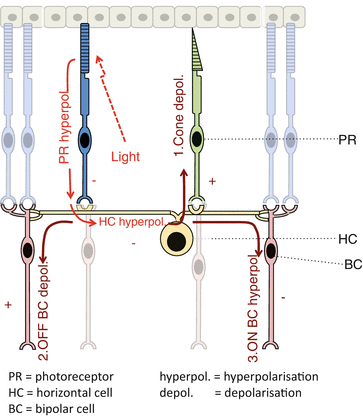
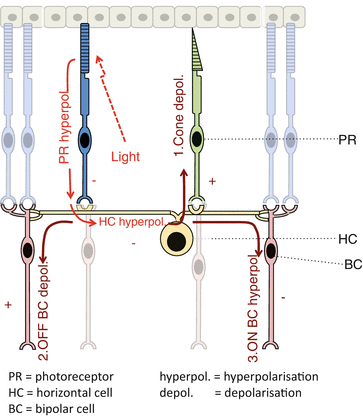
Fig. 8.7
Horizontal cell inhibitory feedforward and feedback loops. PR photoreceptor, HC horizontal cell, BC bipolar cell, hyperpol. hyperpolarization, depol. depolarization
Horizontal cell stimulation causes:
(a)
Depolarization of photoreceptors (inhibitory feedback)
(b)
Depolarization of OFF (hyperpolarizing) bipolar cells (inhibitory feedforward signal)
Bipolar Cells
Bipolar cells (BCs) carry signal from photoreceptor cells to ganglion cells and amacrine cells.
They contain synaptic ribbons and use glutamate as their neurotransmitter, released on graded depolarization in response to photoreceptor and/or HC signal [120].
In the IPL, BCs form dyads (i.e., ribbon synapses which typically have two postsynaptic processes deriving from one amacrine and one ganglion cell, two ganglion cells, or two amacrine cells) [122].
1.
Convergence
At the fovea and perifovea:
With increasing eccentricity BCs have a larger receptive field as more photoreceptor cells converge onto a single BC [86].
Convergence allows BCs to sample signal from multiple photoreceptor cells, important in resolving dim light signal from background electrical activity (“noise”) of photoreceptor cells.
2.
Bipolar cell types (Fig. 8.8)
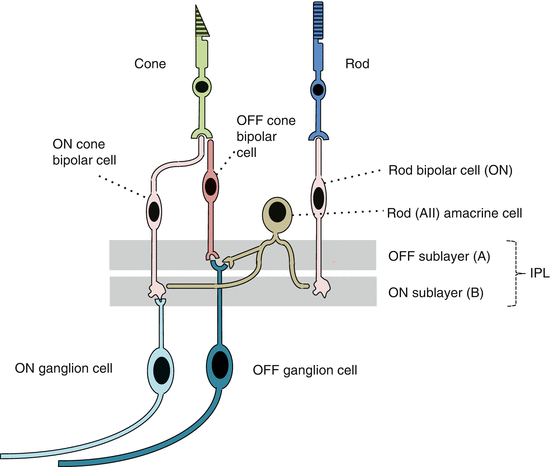
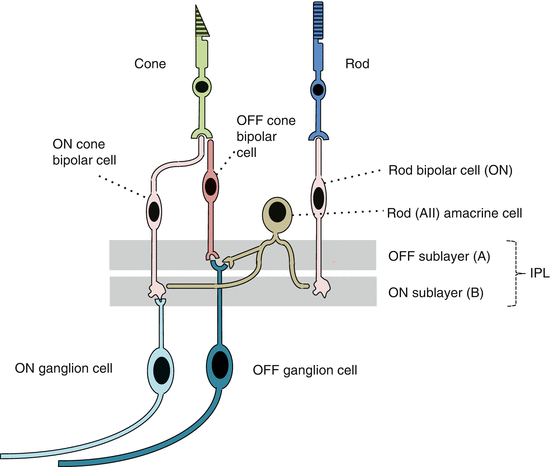
Fig. 8.8
ON and OFF bipolar cell channels. Rod bipolar cells synapse directly with amacrine AII cells and modulate cone ON and OFF pathways (Based on Daw et al. [132])
BCs can be classified into cone and rod BCs.
There are at least 11 cone bipolar types which contact cones and a single type of rod bipolar cell which contact rods. Cone bipolar cells can be subdivided by the number of cones they contact and by their stratification in the inner plexiform layer [126, 127].
Physiologically BCs can be classified into ON or OFF BCs; cone BCs can be ON or OFF; rod BCs are ON [121].
3.
OFF channels
OFF BCs express ionotropic glutamate receptors and hyperpolarize (sign conserving) in response to light-induced photoreceptor hyperpolarization [83].
OFF BCs usually flat contacts with cone pedicles and their axons stratify in sublamina A of the IPL [128, 129].
OFF bipolar cells synapse with OFF ganglion cells, amacrine cells, and/or ON/OFF ganglion cells.
4.
ON channels
(i)
Cone transmission
Cone ON BCs synapse with ON ganglion cells, amacrine cells and/or ON/OFF ganglion cells.
(ii)
Rod transmission
Rod BCs synapse with AII amacrine cells, which also depolarized in response to a light stimulus.
AII amacrine cells:
(a)
Form electrical synapses with ON cone bipolar cells which in turn contact ON ganglion cells [130].
(b)
Make inhibitory chemical synapses onto OFF bipolar cells which in turn contact OFF ganglion cells; this is the classical rod pathway.
Rods also contribute to cone signal through electric coupling.
In addition a low proportion of rods contacts cone bipolar cells directly [131].
Amacrine Cells
There are at least 40 types of amacrine cell.
Most amacrine cells (ACs) are found in the IPL; some (displaced ACs) are found in the GCL [133].
ACs receive excitatory signal from BCs and provide inhibitory input to BCs, other ACs, and ganglion cells [134].
ACs inhibit ganglion cells and other ACs by release of glycine or GABA [135].
Some ACs are dopaminergic, projecting widely and facilitating light adaptation in the retina [133].
1.
Inhibitory function
Inhibitory ACs provide the majority of ganglion cell (GC) input, indicating the importance of amacrine inhibition in shaping retinal output [137].
Glycinergic ACs have narrow branching processes that extend vertically in the IPL. Glycinergic inhibition enhances light responses in BCs, ACs, and ganglion cells in reduced illumination [139–141].
GABAergic ACs have widely branching axonal processes used for lateral signaling. They contribute to surround inhibition for CSARFs [142, 143].
2.
3.
4.
Polyaxonal amacrine cells
Polyaxonal cells (also known as axonal cells) have distinct axons that project within the IPL.
Dopaminergic polyaxonal cells have predominantly ON responses. They reduce cell-cell coupling which facilitates retinal light adaptation by enhancing cone and diminishing rod signal [109].
Ganglion Cells
GCs collect all visual information processed in the retina to send neural message towards central nervous system targets via the optic nerve.
GCs receive input from BC and AC within the IPL [150].
Separate rod and cone channels converge onto GCs with information from both pathways [151].
GCs predominantly use glutamate for neurotransmission [152].
1.
Ganglion cell classification
Cells from each GC type form a mosaic distributed across the entire retina [154].
Broadly GCs can be classified into:
2.
Center-surround organization (Fig. 8.6)
Contrast-sensitive GCs have receptive fields consisting of a central area surrounded by an annular area. These can be either ON-center OFF-peripheral or OFF-center ON-peripheral [91, 155].
This enhances detection of borders and contrast.
ON-center GCs are stimulated by ON BCs in the center and OFF BCs in the periphery.
An ON signal in both areas results in significantly less stimulation.
3.
X- and Y-cell responses
Y (transient) cells are sensitive to texture motion; they are activated when a fine grating shifts in either direction despite no change in average illumination [94].
This is achieved by underlying BC connections: each shift excites some BCs and inhibits others.
4.
P (midget) and M (parasol) channels (Table 8.3)
P (midget) ganglion cells | M (parasol) ganglion cells | |
---|---|---|
Receptive field size | Relatively small | Relatively large |
Number in retina (approximately) | 1,000,000 | 100,000 |
Location | More common in the central retina | More common in the periphery |
Axonal conduction speed | Relatively slow | Relatively fast |
Flicker fusion frequency | Relatively low | Relatively high |
Peak spatial sensitivity | High spatial frequencies (i.e., high acuity) | Lower spatial frequencies |
Contrast sensitivity | Low | High |
Color sensitivity | Yes | No |
Motion sensitivity | No | Yes |
Stereopsis | No | Yes |
Lateral geniculate nucleus projections | Parvocellular layers | Magnocellular layers |
Midget GCs are highly prevalent in the foveal region, where there is minimal convergence.
The M channel (consisting of parasol GCs) conveys motion and form vision at low contrast and gross stereoacuity [166].
Both have ON/OFF subdivisions; in addition the P system has color opponent sub-channels [83].
5.
Color-selective ganglion cells (see Chapter 24, Color Vision)
Color is encoded by midget GCs.
(i)
(ii)
Blue/yellow opponency
Blue ON/yellow OFF opponent small bistratified GCs receive excitatory signal from blue ON BCs that receive signal from blue cones outside the central fovea [88, 89, 169].
Inhibitory yellow OFF input is summated from red and green signals from diffuse OFF BC inputs [170].
In addition melanopsin-containing GCs may contribute to coding of blue OFF/yellow ON opponency [171].
6.