Spectral Domain and Swept-Source Optical Coherence Tomography Optical coherence tomography angiography (OCTA), with the ability to visualize vasculature in vivo without the injection of dye, represents an exciting advancement in the field of ophthalmic imaging. Over the next few years, there are likely to be rapid advances in both the software and the hardware components of this technology, which will enhance our ability to visualize ophthalmic structures in health and disease. In this chapter, we will touch upon some of the advancements that are on the horizon for OCTA technology. Spectral-domain optical coherence tomography (SD-OCT) devices are widely used to evaluate retinal and choroidal diseases and form the majority of the machines that are available to clinicians for performing OCTA. Currently, all the commercially available OCTA devices are spectral-domain devices, with the exception of the Topcon DRI Triton, which is a swept-source optical coherence tomography (SS-OCT) device. However, visualization of vascular structures beneath the retinal pigment epithelium (RPE) using shorter wavelength spectral-domain devices may be limited, which will be discussed later in this chapter. Longer wavelength SS-OCT technology may provide a solution for imaging through media opacities and better visualizing the choroid.1,2,3,4 Like SD-OCT, SS-OCT is a variation of Fourier-domain OCT.5,6,7,8 The hardware of SS-OCT differs from SD-OCT in several ways, including the light source, bulk optics components, and photodetection devices. Current ophthalmic SS-OCT light sources use a wavelength centered at approximately 1 µm that sweeps across a band of wavelengths. SS-OCT utilizes a point photodetector, while SD-OCT uses a spectrometer consisting of a diffraction grating and a detector array or a line-scan camera.9 Both SD-OCT and SS-OCT devices have been used in OCTA configurations to visualize vasculature in vivo.7,8 New commercially available SS-OCTA devices are likely better able to visualize the choroid and choriocapillaris. However, this is not only due to the swept-source nature of the devices.10,11 The wavelength of the light source also plays an important role in the visualization of vasculature on OCTA, particularly at deeper locations beneath the RPE. Longer-wavelength light sources currently in use in swept-source devices are capable of improved visualization of the choriocapillaris and choroid and improved immunity to ocular opacity. Thus, they may be useful for improved visualization of choroidal neovascularization (CNV), especially the sub-RPE components of the membrane. One trade-off of longer wavelengths, however, is that longer wavelengths have lower axial resolution compared to shorter wavelengths. In a comparative study, a prototype long-wavelength, high-speed SS-OCT (~1,050 nm) OCTA was shown to have improved visualization of CNV compared to a shorter-wavelength (~840 nm), commercially available SD-OCT device.11 In another study using the same SS-OCT prototype, SS-OCTA images of the choriocapillaris underlying drusen showed improved visualization as compared to the shorter wavelength SD-OCTA images.45 The OCT signal contains both amplitude and phase components, and angiography methods can be based on the amplitude, phase, or the complex signal, which is a combination of both amplitude and phase information.12 There are several software-based angiography techniques. An OCTA method that calculates speckle variance was reported by Mariampillai et al.13 More recently, Jia et al described split-spectrum amplitude-decorrelation angiography (SSADA), which splits the spectrum into multiple smaller bands, resulting in improved signal-to-noise ratio.14 The optical microangiography algorithm utilizes a combination of phase and magnitude information for a theoretical improvement in sensitivity.12,15,16,17 Recently, Zhang et al proposed a novel feature space-based optical microangiography method (fsOMAG) in which the flow and static background are differentiated in the feature space, leading to the suppression of angiographic signals from the static background.18 Reisman et al also recently reported the OCT angiography ratio algorithm (OCTARA), which utilizes a ratio method that keeps the full spectrum intact which may preserve axial resolution46. The rapid expansion of OCTA may lead to further development of software-based angiography methods, as well as improvements on existing methods. One additional challenge in the interpretation of OCTA is that projection artifacts can confound interpretation and affect quantification of vascular abnormalities. Zhang et al recently reported a projection-resolved OCTA algorithm that is able to generate OCTA images while minimizing projection artifact, resolving ambiguity between projection artifact and in situ flow.19 OCTA enables rapid, noninvasive visualization of the retinal and choroidal vasculature. It can be easily performed at multiple follow-ups, making it a promising tool for monitoring the progression of disease and guiding treatment decisions. Algorithms for analyzing vessel density and assessing ischemia have been reported in diseases such as diabetic retinopathy (DR), where ischemia has been associated with worse visual outcomes.20,21,22 These studies have demonstrated increased ischemia in DR and other vascular diseases and correlated ischemia with worse visual outcomes. To date, multiple novel algorithms have been applied to eyes with DR to quantify the ratio between areas of flow and areas of flow impairment.23,24 Jia et al have described a quantitative flow index that was applied to a prototype SS-OCT dataset, enabling the quantification of blood flow in CNV, optic disc perfusion in glaucoma, and other vascular abnormalities.25,26,27 Chu et al proposed a five-index quantitative analysis of OCT angiograms, which includes vessel area density, vessel skeleton density, vessel diameter index, vessel perimeter index, and vessel complexity index. This represents a tool that can be used to quantify multiple features of vasculature on OCTA that represents a rapid strategy for interpreting OCT angiograms from multiple perspectives.28 Moving forward, it is also important to consider the utility of these automated algorithms in real-life clinical datasets and normal patients, which include motion artifact and noise, which can affect accurate analysis. Larger studies are needed to validate these methods, as these may represent potential clinical endpoints on OCTA that can be used as biomarkers for future clinical trials. A limitation of OCTA imaging is that the images of the vasculature provide limited information on the speed of flow in vessels. Variable interscan time analysis (VISTA), proposed by Choi and Moult et al,29 is a tool that has been used to differentiate blood flow speeds on OCTA. High-speed systems are able to acquire multiple sequential OCT B-scans at the same location. These sequentially acquired OCT B-scans can then be analyzed with variable interscan time between the pairs. By varying the interscan time, different ranges of blood flow speeds can be visualized.29 The VISTA analysis can be visualized using color coding ( ▶ Fig. 18.1 and ▶ Fig. 18.2) in which the color of the pixel represents the erythrocyte flow at a given location. The hue value of each VISTA pixel is a ratio of the OCTA signal obtained from the 1.5-ms interscan time to the OCTA signal from the 3-ms interscan time. In this case, blue pixels indicate slower speeds and red pixels indicate faster speeds.47 Fig. 18.1 Variable interscan time analysis (VISTA) visualization applied to a 3 × 3 mm optical coherence tomography angiography image from a patient with nonproliferative diabetic retinopathy. In this image, flow speeds of the vasculature in the superficial plexus are visualized using a color-encoded images in which red represents relatively high flow and blue represents areas of relatively low flow. Imaging was performed using a prototype ultrahigh-speed swept-source optical coherence tomography device developed at Massachusetts Institute of Technology. The device uses a 1,050-nm vertical-cavity surface-emitting laser (VCSEL) swept light source with a 400-kHz A-scan rate. Fig. 18.2 Variable interscan time analysis (VISTA) visualization applied to a 3 × 3 mm optical coherence tomography angiography image from a patient with choroidal neovascularization secondary to age-related macular degeneration who had been previously treated with multiple intravitreal injections of anti–vascular endothelial growth factor. In this image, flow speeds of the vasculature in the superficial plexus are visualized using a color-encoded images in which red represents relatively high flow and blue represents areas of relatively low flow. Lower flow vessels (blue and green) can be seen at the periphery of the neovascular membrane, with a central area of relatively higher flow (yellow and red vessels). Imaging was performed using a prototype ultrahigh-speed swept-source optical coherence tomography device developed at Massachusetts Institute of Technology. The device uses a 1,050-nm vertical-cavity surface-emitting laser (VCSEL) swept light source with a 400-kHz A-scan rate.
18.2 Advances in Optical Coherence Tomography Angiography Algorithms
18.3 Quantitative Analysis of Optical Coherence Tomography Angiography
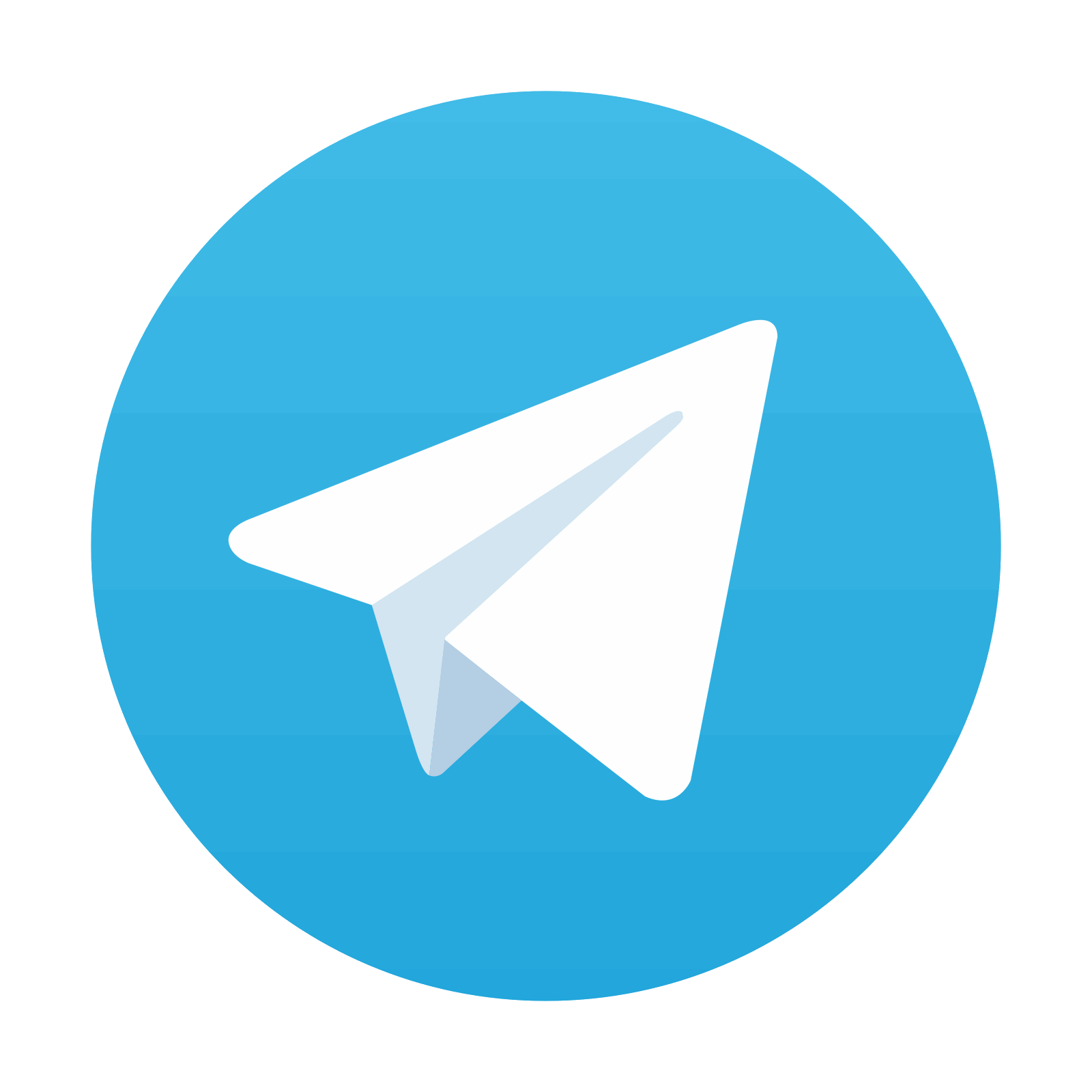
Stay updated, free articles. Join our Telegram channel

Full access? Get Clinical Tree
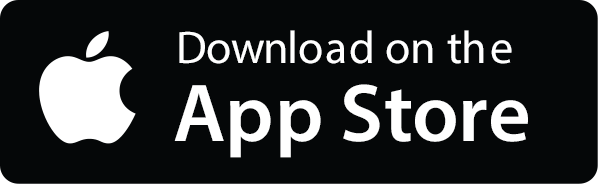
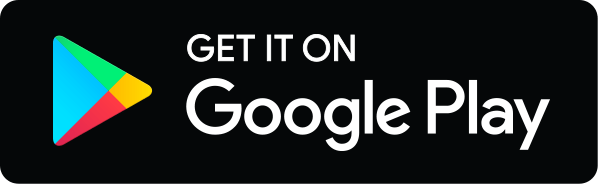