(1)
University of Sydney, Sydney, Australia
Ciliary Body
Overview
The ciliary body is continuous with the iris anteriorly and the choroid posteriorly.
Together these three tissues make up the uveal layer of the eye.
The ciliary body has two main functions:
(a)
Production of aqueous fluid
(b)
Accommodation via ciliary muscle contraction
Anatomy (Fig. 5.1) [1]
The ciliary body, triangular in cross section, is a continuous ring inside the anterior sclera.
It consists of the ciliary muscle, ciliary stroma, and ciliary epithelium.
It extends anteriorly to the scleral spur, where it is firmly attached to the sclera.
Its posterior extent is demarcated by the ora serrata (anterior limit of the retina).
The ciliary body is innervated by parasympathetic, sympathetic, and sensory nerve fibers.
It is divided into the anterior pars plicata and the posterior pars plana.
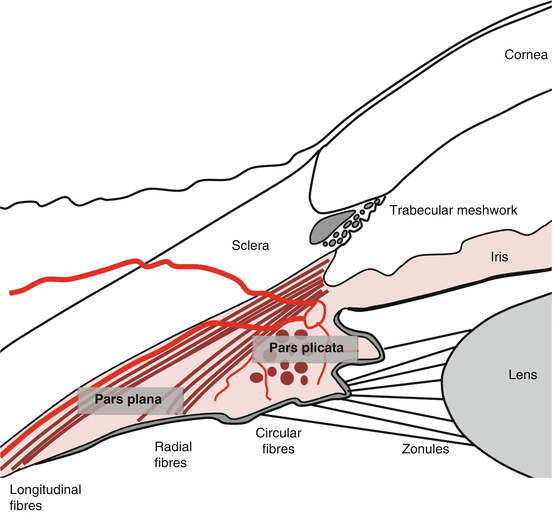
Fig. 5.1
The ciliary body and iris
1.
The pars plicata
The inner surface of the pars plicata is corrugated, with ciliary processes extending from 70 ridges [2].
Ciliary processes are fingerlike projections with:
(a)
A fibrovascular core, surrounded by
(b)
Specialized ciliary double epithelium
Aqueous fluid formation occurs over the ciliary double epithelium.
The corrugated surface increases the surface area for the secretion of fluid.
2.
The ciliary muscle
The ciliary muscle makes up the bulk of the ciliary body.
It has three smooth muscle fiber groups: longitudinal (outer), radial, and circular (inner).
The longitudinal fibers insert at the scleral spur and trabecular meshwork.
On accommodation all muscle groups contract, releasing tension on the zonules (see Chap. 4, The Lens and Accommodation).
3.
The ciliary stroma
The ciliary stroma consists of highly vascularized, loose connective tissue.
It contains multiple capillaries that have fenestrated endothelium.
Fluid accumulates in the stroma by bulk flow across the capillary endothelium.
This fluid is the reservoir of ultrafiltrate from which aqueous is secreted.
4.
The ciliary epithelium (Fig. 5.2)
The ciliary epithelium consists of two layers:
Epithelial cells in these layers are arranged apex to apex; abridging gap junctions permit rapid solute exchange [4].
The double ciliary epithelium is derived embryologically from the optic cup.
(a)
The outer PE from the external layer of the cup.
The outer PE is cuboidal with few organelles.
The inner NPE is columnar with multiple basal foldings; it is highly metabolically active and responsible for active secretion of aqueous from the stromal ultrafiltrate [6].
Tight junctions around the apical margins of the NPE cells form the major permeability barrier of the blood aqueous barrier.
Aqueous Fluid
Overview
Aqueous fluid is a clear plasma-derived ultrafiltrate.
It is normally devoid of proteins, cells, or other macromolecules.
1.
Passage of aqueous fluid
2.
Functions of the aqueous fluid [2]
(a)
Delivery of oxygen and nutrients and removal of waste products, inflammatory products, and other cellular debris from the posterior cornea and crystalline lens
(b)
Provision of a low refractive index transparent medium between the lens and cornea
(c)
Maintenance of intraocular pressure (IOP) for optimal shape and alignment of ocular structures
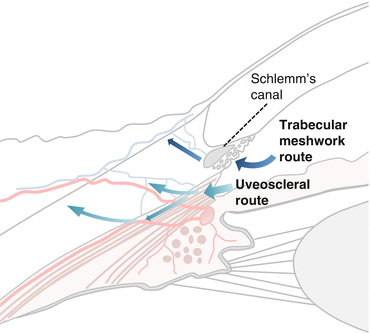
Fig. 5.3
Aqueous outflow pathways
Aqueous Formation
Active secretion of aqueous from ultrafiltrate occurs across the ciliary epithelium.
The majority of aqueous formation is via energy-dependent active secretion and is relatively pressure independent.
1.
Ultrafiltration and diffusion
(i)
Ultrafiltration
Hydrostatic pressure pushes plasma through fenestrated capillaries to create an ultrafiltrate within the stroma of the ciliary processes [12].
This is pressure sensitive, decreasing with increased IOP.
IOP-related resistance to ultrafiltration is an important regulatory mechanism to prevent excessively high IOP.
(ii)
Diffusion
The oncotic pressure gradient between the ciliary stroma and capillaries encourages only a small volume of fluid to extravasate and may in fact favor fluid resorption [11].
2.
Active secretion (Fig. 5.4) [3, 4, 6]
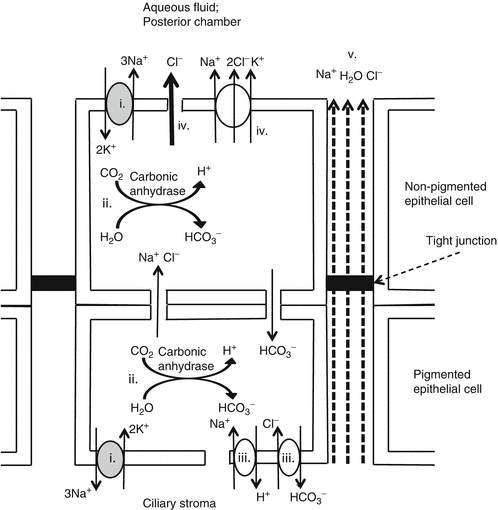
Active secretion is energy dependent.
Under normal conditions it accounts for 80–90 % of aqueous production.
The mechanism of aqueous fluid secretion is as follows:
(ii)
Intracellular carbonic anhydrase converts H2O and CO2 into H + and HCO 3 2− .
(iii)
H + and HCO 3 2− .are transported into the ciliary stroma via Na+/H+ and Cl−/HCO3 − exchangers using the Na+ electrochemical gradient [15].
(iv)
This causes epithelial cells to accumulate high Cl − which enters the posterior chamber by Na+/K+/Cl− cotransport or Cl− channels.
(v)
In response to Cl− flux, Na+ and H2O enter the aqueous transcellularly and paracellularly to maintain electroneutrality and isoosmolarity [16].
The consequence is the secretion of an isosmotic NaCl solution with additional HCO 3 2− .
Ascorbic acid, amino acids, and glucose are actively transported into the aqueous to supply the cornea and lens.
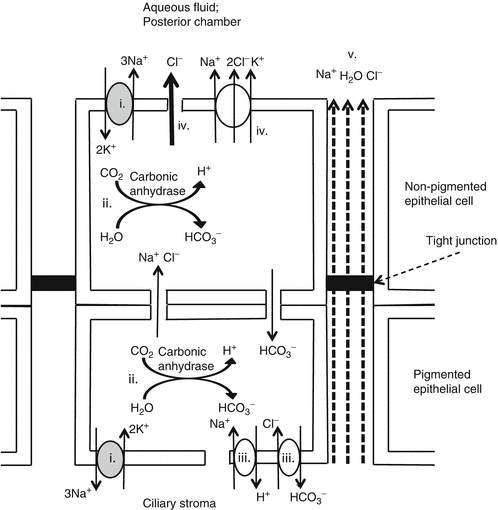
Fig. 5.4
Active secretion of aqueous fluid
3.
Regulation of aqueous formation
IOP-lowering agent | Aqueous production | Trabecular drainage | Uveoscleral drainage | Net effect on IOP |
---|---|---|---|---|
Carbonic anhydrase inhibitors | ↓ | ↓ | ||
β-blockers | ↓ | ↓ | ↓ | ↓ |
α2-agonists | ↓ | ↑ | ↓ | |
Cholinergics | ↑ | ↓ | ↓ | |
Prostaglandin analogues | ↑ | ↓ |
Composition of Aqueous Fluid (Table 5.2)
Once secreted, aqueous composition is maintained by the blood aqueous barrier preventing mixture with the serum.
There is a passive and active exchange of solutes from the aqueous to surrounding structures (vitreous, cornea, lens, and iris).
The aqueous has a similar osmolarity and Na+ concentration to the serum.
Due to blockage of large molecules by the ciliary epithelium, it has significantly less protein than plasma [24].
Lactate content is high due to anaerobic glycolysis in the lens and cornea.
Table 5.2
Concentration of aqueous solutes relative to plasma
Solute | Aqueous concentration relative to plasma |
---|---|
Sodium | = |
Chloride | ↑ |
Glucose | ↓ |
Amino acids | ↓ |
Bicarbonate | ↓ |
Proteins | ↓↓ |
Lactate | ↑ |
Ascorbate | ↑↑ |
Aqueous Drainage from the Eye (Fig. 5.3)
Aqueous exits the eye via two pathways: the TM and uveoscleral routes.
1.
The trabecular meshwork route [8]
Aqueous traverses the TM, across the inner wall of Schlemm’s canal (SC) into SC.
From there it passes into collector channels, aqueous veins, and into episcleral veins.
It accounts for the majority of aqueous drainage (50–75 %); this may increase with age [26].
This pathway is pressure sensitive; outflow increases with greater IOP.
The degree of pressure sensitivity is called the facility of trabecular outflow or facility [27].
2.
The uveoscleral route [9]
(i)
Pathway
The aqueous passes from the anterior chamber angle into the connective tissue spaces within the ciliary muscle via the iris root and anterior face of the ciliary body.
This occurs freely as the anterior ciliary body and iris root lack an endothelial lining [28].
The fluid then passes into the suprachoroidal space and exits the eye through the sclera via scleral perforations or the vortex veins.
The uveoscleral route accounts for 25–50 % of total outflow in young adult; the proportion reduces with age [29].
The role of the uveoscleral route in aqueous outflow was previously underappreciated and is clinically very important as the site of action for prostaglandins, a major class of medications used to treat glaucoma (see Sect. 5.2.6).
(ii)
Flow: independent of intraocular pressure
Uveoscleral flow is IOP independent at IOP levels greater than 7–10 mmHg [30].
As suprachoroidal pressure (P S) is directly dependent on IOP, P S is consistently less than IOP, and uveoscleral flow is constant despite IOP fluctuations.
(At IOP less than 7 mmHg, uveoscleral drainage decreases because of reduced net pressure gradient) [28].
(iii)
Proposed benefit of uveoscleral route
Uveoscleral outflow may be somewhat analogous to lymphatic drainage in the circulatory system.
The uveoscleral system probably evolved to protect the eye from very high IOP rises during inflammation.
Inflammation causes the TM to become clogged by inflammatory cells and debris reducing trabecular outflow; however, locally produced prostaglandins enhance uveoscleral drainage of the aqueous preventing dangerously high IOP [31].
The Trabecular Meshwork and Schlemm’s Canal (Figs. 5.1 and 5.5)
The TM is located at the angle of the eye near the insertion of the iris root.
It extends from Schwalbe’s line anteriorly to the scleral spur posteriorly.
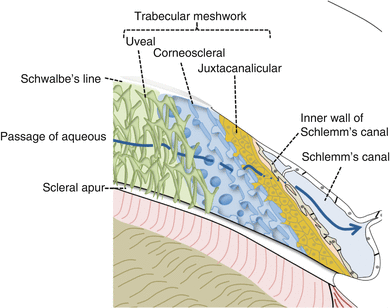
Fig. 5.5
The trabecular meshwork
1.
The uveal and corneoscleral layers
These have trabecular lamellae of extracellular matrix surrounded by endothelial cells.
There are wide openings between the strands allowing passage of aqueous fluid [33].
2.
The juxtacanalicular layer
It consists of several endothelial cell layers embedded in the extracellular matrix (ECM).
The aqueous must pass through these endothelial cells and the ECM [8].
It overlies the continuous endothelium of the inner wall of SC.
3.
Mechanisms of outflow
Several models have been used to explain pressure-sensitive outflow at the TM.
They do not necessarily contradict, and all may be present to some degree.
(i)
(ii)
Alternative model 1: the pumping model [37, 38]
Get Clinical Tree app for offline access
TM outflow occurs via a pumping phenomenon determined by tissue compliance.
Pumping is powered by transient IOP rises on blinking, eye movement, and systole.
Pumping causes fluid to pass:
(a)
Across valves that span SCStay updated, free articles. Join our Telegram channel
Full access? Get Clinical Tree
