10 Temporary Gas Tamponades without Drainage: Minimal Intraocular Surgery for Retinal Detachments The use of an intraocular gas bubble in retinal detachment surgery was first described by Ohm in 1911 [1]. At that time, however, the importance of the retinal hole in relation to a retinal detachment was not yet known. Ohm injected air into the vitreous via the drainage site (7 mm from the limbus). To push the retina back into place. – It was a good idea. He applied the method in 2 patients, and the retina reattached. Yet the gas technique did not gain importance. In 1938, Rosengren [2] re-introduced the use of an intraocular air bubble for the treatment of a retinal detachment. To tamponade the retinal hole after drainage (Fig. 10.1). Fig. 10.1 The use of an intraocular air bubble to treat a retinal detachment by tamponading the retinal hole, first performed by Rosengren in 1938 (Figure from [2]). In contrast to Ohm, Rosengren had the advantage that Gonin [3] had since pointed out that the retinal hole is the cause of retinal detachment. Rosengren integrated this concept into his gas operation. That a gas bubble would not go through a retinal break was an original concept. Today, this concept is obvious to all those, who are knowledgeable about the surface tension of a gas bubble. Now a question you might raise: – It improved by a further 10% the results of retinal detachment surgery, which up to that time consisted only of diathermy around the break and drainage. – The rate of reattachment rose to 77%. Rosengren’s operation restored the intraocular volume with gas, flattened the retina with gas, and tamponaded the diathermized break. However, there was a drawback; the gas did not tamponade the break long enough. – The tamponade with air did not last long enough in some eyes for a retinal adhesion to develop sufficient strength to secure the retinal break. – The time required to develop a secure retinal adhesion is at least 7 days. You might remember that after 1 week the retinal adhesion is strong enough to secure a retinal break, e.g., when a temporary balloon buckle is removed after 1 week (see Chapter 9.3, pp. 5–8). The maximum of a retinal adhesion after thermal coagulation is reached at 12 days (see Part 1, Chapter 7.2, pp. 96–109). – A certain number of detachments pulled apart on the 3rd or 4th day when the air tamponade had disappeared. However, the information available today about the time it takes for a retinal adhesion to develop sufficient strength was not available in 1938. This information did not become available until more than 30 years later, after extensive animal experiments had been performed [4,5]. Redetachment after the Rosengren procedure called for a better procedure. Retinal surgeons did not give up and the search for a better method continued. There was indeed a small group of detachments (about 7%) which proved unresponsive to minimal extraocular surgery, i.e., to segmental buckling without drainage. – Tears at the posterior pole – Multiple tears close together, but at different latitude. Having perceived the limits of scleral buckling, in 1965 Lincoff started animal experiments with various gases in search of a gas that would persist in the eye for a longer time than air. At that time the expansion potential of a gas (which would provide conditions for a nondrainage procedure with a gas) was not yet an issue. The longevity of an intraocular gas bubble depends largely upon its solubility. Sulfur hexafluoride (SF6) proved to be the best of the gases initially tested. Additional reports about the clinical use of SF6 followed from: Norton [12], McLean and Norton [13], Abrahms et al [14], Fineberg et al [15], Laqua and Wessing [16], Kreissig [17], Kroll et al [18], Hausmann [19], etc. Sulfur hexafluoride or SF6 (Fig. 10.2) had been used in medicine for pneumothorax procedures. That was one of the reasons why Lincoff assumed it would not be toxic and might be suitable for the eye [10,11]. First of all let us recapitulate: SF6 is colorless and odorless. It doubles its volume over a period of 24 to 48 hours. Fig. 10.2 The chemical structure of SF6, the first expanding gas, that was used in detachment surgery. The disappearance or absorption time of SF6 is twice as long as that of air. It takes 6 days for the bubble to diminish to half volume and 3 to 4 weeks for the last small gas bubble to disappear. However, for clinical use only the so-called half-life of a gas bubble, representing the time of a therapeutic gas volume, is of clinical interest (see Chapter 10.3, pp. 121–148). A temporary increase in intraocular pressure can occur. However, if the eye has a normal outflow and care is taken in the postoperative positioning of the patient’s head (not to occlude the chamber angle by the gas bubble), a rise in intraocular pressure can be avoided. A posterior subcapsular cataract can develop if more than ⅓ of the lens capsule is subtended by the gas bubble. The FDA assigned a special toxicity to SF6 due to traces of toxic contaminants, such as, sulfur pentafluoride or hydrogen fluoride, present in commercially available SF6 containers. However, toxicity of SF6 to the retina could not be found, as confirmed by Fineberg et al after electron microscopic studies in monkeys and rabbits [15]. – Either because of the extent – Or the localization of the tear or tears. – This was a relative drawback in the treatment of retinal detachment. – You also will recall that nondrainage constituted the essentials of a minimal procedure with a minimum of complications. For this reason we tried to use the expansion potential of the gas when we began to treat selected problem detachments with SF6. The aim was to further reduce the morbidity of the gas operation. Actually we wanted to limit this procedure to a gas injection with cryopexy or laser coagulation and without drainage. Fig. 10.3a An expanding gas is injected into the vitreous. The bubble will expand to maximal size as nitrogen, oxygen, and carbon dioxide diffuse into the bubble. Fig. 10.3b The expanding gas has acquired its maximal volume. An equilibrium is reached when the rate of nitrogen diffusing into the bubble is equal to the diffusion of the expanding gas into the surrounding fluids. Fig. 10.3c The gas bubble starts to decrease in volume gradually as the gases diffuse into the surrounding fluids. At the Meeting of the German Ophthalmological Society in 1978 the first results of this gas technique were reported [17]. Here are some details of this clinical study with SF6. The expanding-gas operation without drainage, described by Kreissig in 1979 (17), was applied in a small series of detachments, not suitable for external buckling, by using SF6 combined with cryopexy and/or laser coagulation. As you might recall, several years later the indication of this gas technique was extended to the treatment of uncomplicated detachments by Dominguez in 1985 [20] and by Hilton and Grizzard in 1986. However, not aware of the report of Kreissig in 1979 on the use of SF6 without drainage, Hilton re-introduced this gas technique without drainage as “pneumatic retinopexy” [21]. In our series the expanding-gas technique was applied to the following 15 problem detachments: 9 giant tears (extending from 75° to 195°), 3 reoperations with moderate PVR, and 3 detachments with breaks at the posterior pole. In 6 of these detachments SF6 was injected without prior drainage of subretinal fluid. You might raise the following question: Postoperative PVR had caused failure in 6 out of 15 problem detachments treated. – A second and more cogent reason was that in 1979, an optimal alternative, i.e., the balloon operation representing as well a temporary tamponade without drainage, became available for uncomplicated detachments [22]. – But it was an extraocular procedure, i.e., there was no need to drain or to enter the vitreous for an intraocular injection. – Therefore, with the balloon operation the risk of postoperative PVR was minimal at 0.2% in comparison to the higher incidence with pneumatic retinopexy at 4% [23]. Let us return to SF6 and the results we obtained in treating problem detachments. The expanding-gas operation had failed in all 3 eyes with preoperative PVR because the intraocular gas caused a progression of PVR. Of the 9 detachments with a giant tear, 6 were reattached and 3 failed due to the development of PVR. Of the 3 detachments with holes at the posterior pole, all of them were reattached and it was achieved: – Without redetachment after the gas bubble had receded. – Without reoperation. As already pointed out, the worst complication of intraocular gas was the development of PVR. An abrupt increase in intraocular pressure or the development of a persisting cataract was not observed. Subcapsular opacities in the posterior lens capsule disappeared within days after the gas bubble had been absorbed. The favorable results concerning the lens were obtained by postoperative positioning, which kept at least ⅔ of the lens free from contact with the gas bubble. In 9 of the 15 problem detachments the retina was reattached with the expanding-gas technique. The visual acuity remained unchanged, if the macula was attached preoperatively, or it increased. The increase in visual acuity was between 20/100 and 20/20. During the follow-up (4 months to 4 years) the visual acuity remained unchanged. The results of the expanding-gas operation with SF6, performed without drainage in 6 out of 15 problem detachments, were encouraging. Reattachment was obtained in all of the 3 detachments with posterior holes. The expanding-gas operation also proved to be better than external buckling in the treatment of a detachment with a giant tear: In 6 out of 9 eyes with a tear between 75° and 195° in extent the retina was reattached. – Optimal postoperative visual acuity – No deterioration of visual acuity during long-term follow-up. The high incidence of postoperative PVR experienced after treatment with intraocular gas prevented us from extending the expanding-gas operation without drainage into the treatment of uncomplicated detachments. – Nondrainage – A temporary tamponade, but in addition: – An extraocular approach (see Chapter 9.3 pp. 5–8) – Significantly less postoperative PVR than with the expanding-gas technique (later called pneumatic retinopexy), since the vitreous had not to be entered when applying a parabulbar balloon (see Chapter 9.7, pp. 92–98). The first report on an intraocular injection of a perfluorocarbon gas was by Vygantas et al [24] in 1973. They used octafluorocyclobutane or C4F8 (Fig. 10.4) in their attempt to find a non-toxic gas, which would be more slowly absorbed than air. Vygantas et al demonstrated that C4F8 and SF6 were superior to air in terms of longevity in the vitreous. C4F8 would stay 7½ days in the vitreous and seemed to be less toxic than SF6. Fig. 10.4 Chemical structure of C4F8, a cyclo-perfluorocarbon gas, with an expansion coefficient similar to that of SF6. – The straight-chain perfluorocarbon gases were extensively tested in animal experiments and in clinical studies. These gases provided a spectrum of intraocular longevities and, in addition, expansile potentials. – Suddenly it was feasible for the surgeon to select the appropriate gas for the individual detachment and to perform again a surgery without drainage of subretinal fluid. These gases were studied in detail first in animal experiments and then in clinical trials by Lincoff, Kreissig, and their retinal fellows from 1980 [25,26] to 1996 [27–29]. There are 4 straight-chain perfluorocarbon gases (Fig. 10.5): CF4 (perfluoromethane), C2F6 (perfluoroethane), C3F8(perfluoropro-pane), and C4F10 (perfluoro-n-butane). These gases are inert, colorless and odorless like SF6 or C4F8. The specific gravity of the gases is less than that of intraocular fluid and therefore the gas bubble can exert a buoyant force superiorly. The gas can push the retina against the pigment epithelium and, because of surface tension, the gas bubble will not pass through the retinal hole. Fig. 10.5a Chemical structure of the 4 straight-chain perfluorocarbon gases: Intraocular expansion and longevity varies with the length of the carbon chains. The straight-chain perfluorocarbon gases came about when we (Lincoff, Kreissig, and the retinal fellows) were looking for a gas that might last longer than SF6 in the eye. Fig. 10.5b 4 perfluorocarbon gases in gas tanks of laboratory size. You will also recollect that the Rosengren technique sometimes failed because the air left the eye too early. SF6 stayed in the eye longer, but for some detachments it was not yet an optimal gas. – Or the volume after expansion was not large enough, e.g., to cover a posterior break. As the chain of the perfluorocarbon gases increases in length, the insolubility of the gas increases. You should know that it is not the molecular size of the gas, but its insolubility that leads to a longer duration of the bubble in the eye. Fig. 10.6 Disappearance times of intraocular gas in rabbit eyes: Bars compare intraocular disappearance times of 0.4 ml of air, SF6, CF4, C2F6, and C3F8 with 0.2 ml of C4F10. The solid or shaded portion of bars represent the time taken for half of the maximum of expanded volume to disappear. The remainder of bars demonstrates the disproportionately long time that it takes for small bubbles to be absorbed [30]. – A gas has to go into solution in order to leave the eye, that is, to cross the blood aqueous barrier. Now, for your recollection, some physics which is needed to understand the following: Because the blood gases, such as N2, O2, and CO2, are more soluble than the perfluorocarbon gases, they diffuse more rapidly across the blood aqueous barrier towards the gas bubble, resulting in expansion of the perfluorocarbon bubble [25]. In the next Figure (Fig. 10.6) you see the longevity of the 4 perfluorocarbon gases, the data which were obtained in animal experiments [30]. CF4 has a longevity almost identical to that of SF6; C2F 6stays in the eye 3.3 times longer than SF6,C3F8 stays 6 times longer, and C4F10 stays in the eye 13 times longer. For instance, C4F10 has left the eye of a rabbit at 90 days, but weeks before there are only small bubbles present. Therefore, the total longevity of a gas bubble in the eye is not the significant factor that a surgeon would like to consider when selecting a gas for an optimal tamponade. – It is the volume for a sufficient tamponade. – Therefore, when talking about longevity of a gas, we define it better as the half-life of a gas. As you will recall, small residual bubbles of a gas are of little value for a retinal tamponade, and they persist for an unproportionally long time in the eye. When the disappearance time of the 4 gases was plotted on a log scale [26], it was found that the disappearance rate plots as a straight line. You will notice that the slope of the line in the diagram is the same for the same gas (Fig. 10.7), regardless of how much of it was injected into the eye. This is because its disappearance rate, i.e., the half-life, is the same whether you start with 1 ml or 2 ml of gas. This data was obtained in a rabbit model. The rabbit retina is thinner than that of the human; therefore, the gases will disappear less rapidly in patients. The half-life of the 4 perfluorocarbon gases in patient eyes is diagrammed in Figure 10.8. This data was condensed in a Table (Table 10.1) to facilitate memorizing and looking it up as needed (e.g., in the operating room, at rounds). Fig. 10.7 Disappearance rates of 4 perfluorocarbon gases in rabbit eyes: CF4, C2F6, C3F8, and C4F10. Volume is plotted against time on logarithmic scale. Closed symbols indicate data points for initial injection of 0.2 ml; open symbols indicate data points for initial 0.3 ml injection. Volume determinations of less than 0.1 ml are not included [26]. Now let us transfer this knowledge to a clinical situation. • After 3 days the volume will be 1 ml. • After 12 days it will be 0.5 ml. • After 24 days only 0.25 ml, a little bubble of no therapeutical value remains. Fig. 10.8 Disappearance time of the 4 straight-chain perfluorocarbon gases in patient eyes: The volumes injected varied according to the retinal problem to be treated. The dark gray portion of the bars indicates the time taken for the expanded volumes of gas to diminish to half volume [31]. • After 3 days it will expand to 2 ml. • After 12 days it will be 1 ml. • After 24 days it will be 0.5 ml, after which only small bubbles will remain. Seeing all 4 gases in perspective: The retinal adhesion reaches maximal adhesive strength at 12 days. During subsequent months it will remodel, but its adhesion will not increase further. You might remember that a light or medium lesion matures in 12 days and a heavy lesion in 14 days. So 12 days represent the time a gas bubble must tamponade a retinal break to obtain a maximum of adhesions around it. The half-life of the gases C3F8 and C4F10 is much longer, being 20 to 24 days and 45 days respectively.
10.1 History
Why did Ohm inject air into the vitreous?
To compensate for the loss of intraocular volume after drainage and
What did Rosengren do differently?
He used the intraocular air bubble
Did the Rosengren technique improve the rate of reattachment?
Yes:
Why?
– You might recall that air has a half-life of 1½ to 3 days (this is dependent upon how old you are and whether you are a high myope).
Bearing these facts in mind, Rosengren’s final results were as follows:
In the early 50’s various scleral buckling procedures were developed that yielded better results than the intraocular gas tamponade—though the air injection represented a significant conceptual advance.
But the idea for an external tamponade of the break was first realized by Custodis [6] in 1953: He reduced extensive buckling then in practice to a buckle limited to the area of the break. In addition, he eliminated drainage of subretinal fluid. This new concept was made feasible by the use of elastic plombages. However, there were complications that jeopardized the acceptance of this procedure. Full thickness scleral diathermy facilitated scleral abscess in a number of patients and the explant Polyviol, made of gum Arabic, Congo red, and polyvinyl alcohol, was irritative.
In 1963 Lincoff modified this operation [7,8] to eliminate the complications induced by the explant and diathermy: The cryo-surgical detachment operation was developed (see Part 1, Chapter 8.1, pp. 126–127).
The operation was further refined during the 1970’s: The so-called minimal extraocular surgery for retinal detachment (Kreissig [9]) provided optimal results with a reattachment rate of substantially above 90%.
For which retinal detachments might external buckling have reached its limits? Which detachments will cross your mind?
– Giant tears
Therefore, in 1967 Lincoff re-introduced the gas technique, limited to detachments not suitable for scleral buckling [10, 11]. He had replaced air by SF6 which stays twice as long in the eye. With this modified gas technique the problem of the Rosengren technique—a tamponade of too short duration—was eliminated.
The injection of SF6 was, however, preceded by drainage of subretinal fluid, but it could provide a tamponade of sufficient intraocular duration for a retinal adhesion to develop. It was this which represented the progress.
10.2 Sulfur Hexafluoride (SF6)
10.2.1 History
Why was SF6 preferred to air?
Because it remained twice as long as air in the vitreous.
Why did SF6 remain in the eye longer?
SF 6 is an inert gas that is relatively insoluble in the watery vitreous and therefore stays in the eye for a longer period.
Why does the increase in volume occur?
SF6 expands because blood gases, i. e., nitrogen, oxygen, and carbon dioxide, diffuse into the intraocular gas bubble. At equilibrium the volume of the SF6 bubble has increased 1.9 times. It takes about 48 hours to reach the peak volume.
What about intraocular complications, since SF6 will be in the vitreous longer?
I assume you are recalling the previous statement: Every new treatment will eventually present some complications or limitations.
In the subsequent years the gas operation with SF 6 evolved to become a technique of choice for the treatment of detachments not suitable for scleral buckling:
Why?
– Because already with segmental buckling (sponge operation), drainage of subretinal fluid was no longer needed.
Convinced of the advantages of a nondrainage procedure, Kreissig had been trying since 1974 to transfer this concept to the upcoming gas technique for special tears unsuitable for buckling [17].
How to sustain nondrainage with the gas technique?
It crossed our mind that we could use the expansion potentials of the gas (Fig. 10.3) in the same way as we had already been using the elasticity of the sponge to obtain, in spite of nondrainage, a larger tamponade. This was enabled by the egress of intraocular fluid.
Between 1974 and 1978 Kreissig [17] succeeded in applying this SF 6-gas technique without drainage and additional thermal coagulation in 6 problem detachments.
10.2.2 Clinical Study with Expanding-Gas Operation without Drainage
Why, in 1979, was the indication of the expanding-gas operation without drainage not also extended to uncomplicated detachments, as Hilton and Grizzard did 7 years later?
– Our main reason was the morbidity associated with intraocular gas:
But why was the balloon operation preferable to the expanding-gas operation?
– The balloon procedure was also a minimal operation because no scleral sutures were needed.
These were the 3 reasons why, as of 1979, we preferred the balloon operation as treatment for uncomplicated detachments.
As a result, the expanding-gas operation was reserved for complicated detachments, not suitable for scleral buckling.
Anatomical results
Therefore, in 1979 we had concluded that for detachments with hole (s) at the posterior pole and to a lesser degree giant tears the expanding-gas operation without drainage represented a procedure of choice [17].
Functional results
10.2.3 Conclusion
Therefore, detachments with posterior holes seemed to represent the indication of choice for the expanding-gas operation.
In detachments with a giant tear the gas technique represented an atraumatic procedure with:
Instead, as of 1979, we treated uncomplicated detachments with the balloon procedure which also provided:
10.3 The Perfluorocarbon Gases
10.3.1 The Cyclo-Perfluorocarbon Gas
Why did C4F8 not become popular for detachment surgery?
– This was not due to the toxicity or adverse side effects of this gas, but—as is often the case in research—something better and more versatile appeared at that time.
10.3.2 The Straight-Chain Perfluorocarbon Gases
10.3.2.1 Physical Properties
As the length of the carbon chain increases, the solubility decreases. This results in an increase of expansile ability and intraocular longevity of the gases.
Intraocular longevity
An optimal gas should remain in the eye for at least as long as it takes for a retinal adhesion of sufficient strength to develop; this takes 1 week [4,5].
Why?
– Either the tamponade was not long enough, e.g., for the development of secure retinal scars around a complex tear formation.
How does a longer intraocular duration of a gas bubble come about?
– A gas can only leave the eye in a fluid phase.
But what is important when you select a gas as tamponade?
The time at which you will still have a therapeutic volume:
This indicates that the gases disappear as a first-order exponential function, and so can be talked about in terms of half-life.
– If you start, for example, with an injection of 0.3 ml of undiluted C2F6 into the vitreous:
– If, however, you start with 0.6 ml of C2F6:
C2F6, with a half-life of 10 to 12 days, seems to be the ideal gas: Why?
Because it provides a therapeutic bubble during the time it takes for a maximal adhesion to develop from laser or cryopexy treatment (see Part 1, Chapters 7.2.2 and 7.2.3, pp. 98–101).
Gas | Longevity (days) |
CF4 | 6 |
C2F6 | 10 |
C3F8 | 35 |
C4F10 | 45 |
However, such a long half-life of a gas is unnecessary: Why?
– Because gases serve no purpose beyond 12 or 14 days.
– Instead, they only increase morbidity, i. e., PVR and new breaks.
– This also implies that whatever you want to achieve with an intraocular gas bubble, will have to be obtained within 12 days, the time needed for the development of a maximum of adhesions around the tears.
Admittedly, early in our gas research and clinical work [17, 30] we thought that a longer gas tamponade might inhibit proliferative vitreoretinopathy (PVR), but this proved not to be the case.
On the contrary:
– Gases provoke a break down of the blood aqueous barrier, which might provoke PVR.
Consequently:
– The shorter period a gas remains in the eye, the better.
– Whenever possible, select a shorter acting gas, such as CF4 or C2F6, for the retinal problem.
Expansion
Expansion is another aspect of the perfluorocarbon gases [25]. Because the perfluorocarbon gases are relatively insoluble and if you have an insoluble perfluorocarbon gas on one side of the blood aqueous barrier and nitrogen, oxygen, and carbon dioxide on the other, the more soluble gases will diffuse into the eye. All 4 perfluorocarbon gases, because they are less soluble than the gases in the blood stream, increase in volume when they are injected into the eye. This occurs over a short time.
Fig. 10.9 Expansion of the 4 perfluorocarbon gases and SF6 in patient eyes [31].
The maximal increase in volume of all 4 gases occurs during the first 24 hours:
– Therefore, during this period it is necessary to measure the intraocular pressure at intervals if diminished outflow of the eye is suspected.
– The expansion of CF4 is complete in 1 day, and of C2F6, C3F8, and C4F10 in 3 days (Fig. 10.9).
These data are condensed in a Table (Table 10.2) to facilitate looking them up as needed (e.g., in the operating room).
Gas | Intraocular expansion |
CF 4 | x 1.9 |
C2F6 | x 3.3 |
C3F8 | x 4.0 |
C4F10 | x 5.0 |
The expansion potential of the perfluorocarbon gases can be used to one’s advantage, i.e., as already practiced with SF6 [17], to avoid drainage of subretinal fluid and still obtain a large intraocular gas bubble.
Clinical example
If you want to have a 1 ml bubble to tamponade 2 superior tears, such as the ones in Figure 10.10a, you can inject 0.3 ml of C 2F6 without drainage and the gas will expand to 1 ml (Fig. 10.10b). The most you can inject into an eye without draining subretinal fluid is about 0.3 ml of gas. This is because the eye can expand by approximately 5% of its volume and 5% of a 6 ml eye is 0.3 ml.
Fig. 10.10a 2-quadrant detachment with a large tear and a small horseshoe tear in the area of the superior rectus muscle: The macula is attached and the patient has full visual acuity. The 2 tears are too large in extent for a temporary balloon buckle, and 2 radial sponges in the area of the superior rectus muscle might cause diplopia.
Fig. 10.10b 0.3 ml of C2F6 was injected without drainage and after expansion at day 3, the gas has a volume of 1.0 ml. The patient was advised to sleep elevated at least 45° to cover the tears. After reattachment, the posterior edge of the large tear was secured with laser lesions; the lateral edges up into the pars plana and the smaller horseshoe tear were treated with cryopexy prior to gas injection. The half-life of C2F6 ranges between 10 and 12 days which is a sufficient time for an adhesion to develop.
If you inject more than 0.3 ml of gas without prior decompression, what will happen?
– Intraocular pressure will rise enough to close the central retinal artery.
– Therefore, whenever you inject a gas, be sure to look at the central retinal artery for patency. It can be a fatal error not to know that you have closed off the central artery, because the patient may lose light perception if the closure persists.
Consequently, every time you inject gas into an eye, examine the central artery for perfusion.
– By and large, 0.2 ml of gas is safe and 0.3 ml is usually possible if you decompress the eye a little before injecting.
– For a larger volume to inject without prior drainage, more decompression can be obtained by applying the balloon-gas procedure, which will be discussed below (see Chapter 10.5, pp. 159–166).
How could it have been possible to inject a larger volume of gas (e.g., 0.6 ml), without closing off the central artery, in an emmetropic eye and without prior drainage of subretinal fluid or decompression by the balloon-gas procedure?
– Reflect!
– The gas had escaped via the connection between the syringe and the millipore filter or at the injection site. The explanation is as easy as this.
Injecting 0.3 ml of gas is about the same as injecting 0.3 ml of water. The effect of gas compression is insignificant, the intraocular pressure will rise either to 50 mmHg or more. This can be understood if you compute the change in volume in relation to absolute pressure: You are really going from 780 mmHg to 830 mmHg, and the reduction in volume 780/810 x 0.3 ml is insignificant.
What is important?
– If you want 1 ml of gas as tamponade in an eye during the period a retinal adhesion will develop,
– Inject 0.3 ml of C2F6 without drainage.
– In 48 to 72 hours there will be 1 ml of gas for a tamponade.
This technique is used for pneumatic retinopexy [21], a method for treating an uncomplicated detachment without drainage of subretinal fluid, which will be discussed below (see Chapter 10.7, pp. 256–258). However, as already explained, the morbidity of the intraocular gases does not, in our opinion, justify their use for uncomplicated detachments.
Another feature of the gases is that they provide a better tamponade than silicone oil or a liquid perfluorocarbon.
Why do the gases provide a stronger tamponade effect than silicone oil?
Because the buoyant force of a bubble of gas is 16 to 17 times more than that of silicone oil.
10.3.2.2 Complications
Lens
The gases will interfere with the metabolism of the lens and cause a posterior subcapsular cataract, if they cover more than ⅓ of the lens. A cataract will begin to manifest itself within 24 hours. At that time you might see a faint crystalline effect within the posterior lens capsule. In 3 days it will become a significant posterior subcapsular cataract in most patients.
This phenomenon was studied in a rabbit model [32]. It was found that if only ⅓ of the lens was covered with gas, you will sometimes get a superior cataract, but this usually disappears. Apparently, there is still enough transfer of metabolic products across the inferior portion of the lens capsule to prevent a cataract from forming.
Consequently, when injecting a gas bubble into the eye of a patient, remember that you do not want to cover more than ⅓ of the lens. If more gas is required, the patient has to tilt the head forward to keep the gas bubble off the back of the lens.
As a rule of thumb:
– If, after a gas operation, there is only a little cataract in a lens, it will usually diminish after some days and result in almost no opacity.
– If you cover the lens completely with a gas bubble for 4 or 5 days, it is likely to cause a permanent lens opacity.
– In the presence of a large gas bubble, if at least ⅓ of the lens is kept free of the bubble by head tilting, after 6 to 12 days vacuoles in the posterior lens might occur.
– In general, however, these vacuoles will regress in subsequent weeks.
Intraocular pressure
Assuming you inject an expanding gas into an eye with a normal outflow and the patient is positioned so that the chamber angle is not compressed by the gas bubble, a rise in intraocular pressure is unlikely.
What are the effects of gas on intraocular pressure during the period of expansion?
As the gas expands, aqueous goes out and intraocular pressure remains unchanged.
It is a fortunate circumstance that the outflow can compensate for the expansion of intraocular gas.
Occasionally there are reports of an increase in pressure after a gas injection. This increase is rarely above 30 mmHg unless the patient has a diminished outflow.
Glaucoma or a diminished outflow is a contraindication for the use of an intraocular gas tamponade: What about going on an airplane with a gas bubble in the eye?
Examine the question in terms of absolute pressures.
At sea level, the absolute intraocular pressure is 777 mmHg and the atmospheric pressure is 760 mmHg. The difference, 17 mmHg, is the intraocular pressure as measured by a tonometer. An ascending airplane depressurizes the cabin to 564 mmHg, the atmospheric pressure at 8,000 feet, even if the plane ascends higher than 8,000 feet. A gas bubble in an eye must increase in volume by the fraction 777/581 to maintain an intraocular pressure of 17 mmHg. This is an increase in volume of approximately ⅓. If the intraocular gas bubble is not too large, the eye can accommodate the expansion.
The increase in intraocular gas volume during flight is partially compensated for by:
– Scleral expansion
– Choroidal compression, followed by
– Accelerated aqueous outflow.
Here you see what actually happens, depicted in a diagram (Fig. 10.11). The lower line represents the change in atmospheric pressure from 760 to 564 mmHg, the change from sea level to 8,000 feet. The top line represents the change in absolute intraocular pressure for an intraocular gas bubble that is about 25% of the ocular volume. In the example the absolute ocular pressure falls, but not as rapidly as the atmospheric pressure. The difference between the 2 lines that increasingly separate is the increasing intraocular pressure. In this example, intraocular pressure rises above 80 mmHg and is symptomatic.
Fig. 10.11 Effect of an ascent being on an airplane from sea level to 8,000 feet on an eye containing a gas bubble too large to be compensated for. The absolute intraocular pressure (AIOP) has fallen, but not as rapidly as the fall in atmospheric pressure (AP). As a result, the intraocular pressure (IOP) has increased (see yellow area) [33].
Fig. 10.12 Chart showing the volume of an intraocular gas bubble in ml and the percentage of total volume measured ophthalmoscopically in disk diameters (dd) of the meniscus above the top of the disk. When the meniscus of the bubble is 4 dd above the top of the disk, the fill is less than 10% (shaded in gray). 10% is the maximal volume with which a patient can travel safely on an airplane.
Therefore, let me make specific recommendations:
How much gas can a patient have in the eye and still go on a commercial airplane?
Approximately 10% of the ocular volume, which is about 0.6 ml.
– The expansion of 0.6 ml can be compensated for by outflow and will not cause pain providing the patient has a normal outflow.
How will you know that the patient has only 0.6 ml of gas or less in the eye?
To determine this in your office, there is a chart from which you can easily read the volume of gas in a patient’s eye in relation to the level of the gas meniscus at the retina (Fig. 10.12).
What is a better way to determine the level of the meniscus of a gas bubble in the eye?
Sighting it with indirect ophthalmoscopy to determine the level of the meniscus of the gas bubble at the retinal surface [29]and to measure it in disk diameters (dd) above the top of the disk:
– If the meniscus of the gas bubble is at the top of the disk, the patient has a 50% bubble.
– If the gas bubble is 4 dd above the top of the disk, the patient has a bubble that is less than 10% of the ocular volume (Fig. 10.12).
Consequently, when your patient asks:
Can I go on an airplane with the gas in my eye?
You will say:
“Let me examine your eye, dilated.”
Measure the level of the meniscus of the gas bubble in dd above the disk.
If it is less than 4 dd in a normal size eye, what will you do then?
You will say:
No, you cannot go on the plane, but come back in a couple of days.
–When the meniscus of the bubble reaches 4 dd above the top of the disk, intraocular volume is less than 10%.
Then you will say to the patient:
“Yes, you can fly on a plane.”
Gases affect the vitreous because they break down the blood aqueous barrier. Within 24 hours after gas is injected, cells and a protein flare appear in the vitreous.
You will recall the facts we addressed when we were talking about nondrainage.
Nondrainge provided the following advantages:
– You do not have to decompress the eye prior to sewing on a buckle and, as a result, you will not have the complications of drainage.
– Since you do not abruptly decompress the eye, there will be no break down of the blood aqueous barrier with an attendant influx of protein and cells into the vitreous.
– In addition, bear the following in mind for the gas operation:
• Every intraocular injection of gas is noxious for the vitreous because it causes a vitreous reaction that is independent of the gas injected.
Constable demonstrated this in experiments almost 25 years ago [34]. He injected air and nitrogen, the gases available in those days, into the vitreous; both gases really caused the same reaction. The perfluorocarbon gases have no special quality, only that they are less soluble. Therefore:
Gases cause a break down of the blood aqueous barrier if they remain in the eye longer than 24 hours.
What can you observe 24 hours after you have injected gas into the vitreous?
1. If you look with the slit lamp, you can already detect a Tyndall effect in the vitreous. In another 12 to 24 hours, you will even see cellular infiltration of the vitreous. The cellular infiltration is not desirable for the vitreous.
Besides the cells in the vitreous, there is another, even more serious effect that a gas injection will cause.
2. If there is a substantial amount of gas in the eye, it will liquefy the vitreous (Fig. 10.13) [35]. We could show that a 90% displacement of the vitreous by gas causes a diminished concentration of hyaluronic acid by 18.6%. Lesser displacements of vitreous caused a lesser loss of hyaluronic acid, but the differences were not significant [36].
– The loss of hyaluronic acid after intraocular gas causes a diminished viscosity of the reconstituted vitreous.
In this context you should recall what will happen to an eye when it becomes aphakic. The eye will lose about ⅓ of its hyaluronic acid content. If you use a gas compression in an eye, you are causing a somehow similar effect on the vitreous. But when you inject gas into the eye, there is another, worse side effect. You can see this in an eye in which the vitreous was compressed by gas (Fig. 10.14). At first we thought that the gas compression might have induced a vitreous detachment.
3. After gas compression cortical vitreous can be seen densely packed over the pars plana and at the back of the lens (Fig. 10.15). This will persist unless it is removed with a vitrector. The same condensation of cortical vitreous can be seen over the disk [37].
Fig. 10.13 Left: The vitreous of an untreated eye is tested for viscosity. A cellulose sponge draws a strand for 10 to 12 cm; the untreated vitreous is viscous. Right: The vitreous after a gas compression. A cellulose sponge draws a strand for only 1 to 2 cm before it parts; the reconstituted vitreous is watery [35].
Fig. 10.14 A gas-filled vitreous cavity with compressed vitreous structures over the disk, the pars plana, and at the back of the lens.
Fig. 10.15 Histological section of the condensed vitreous anteriorly at the edge of the lens in a gas-filled rabbit eye. Note the cells within the vitreous [37].
After the gas has disappeared, the reconstituted vitreous appears optically clear, but only by ophthalmoscopy. With slit lamp bio-microscopy you can see cells and a protein flare. Sometimes the reconstituted vitreous, altered after a gas compression, has a convection current (Fig. 10.16).
What does this mean?
– You will recall the findings in an eye after a pars plana vitrectomy with the vitreous replaced by balanced salt solution.
– Also in the reconstituted vitreous you can observe that the cells behind the lens are descending and probably ascending at the posterior retina. This is caused by an intraocular convection current, which is possible because there are practically no vitreous structures within the vitreous cavity.
The cortical vitreous of a monkey’s eye, that has not been compressed by a gas bubble, looks like a rattan mat on the surface of the retina (Fig. 10.17). The mat appears to be several layers thick.
Fig. 10.16 A slit lamp examination of the reconstituted vitreous (rabbit eye) reveals cells in a convection current [37].
Fig. 10.17 The posterior pole of a monkey eye, not treated by a gas injection (control). In the center is the optic nerve (ON). Note the smooth appearance of the retinal surface [38].
Fig. 10.18 The posterior pole of a monkey eye 6 weeks after gas injection. The lamellae of the cortical vitreous are torn; 3 levels of lamellae can be recognized as well as areas of bald retina. ON = optic nerve [38].
In the next Figure (Fig. 10.18) you see the retina of a monkey that has undergone a gas compression with C3F8. The vitreous, in particular the cortical vitreous, is severely disorganized [38]. In some areas it is torn and in other areas there are strips of cortical vitreous. Three layers of disorganization in the cortical vitreous are evident (Fig. 10.19).
4. After a gas compression, the cortical vitreous on the surface of the retina is torn and in total disorganization (Fig. 10.20).
– What you have just seen is in a monkey retina, treated with gas.
– You can imagine that when you inject gas into a patient’s eye, you probably induce similar changes.
Fig. 10.19 Schematic representation of the lamellar pattern seen in Figure 10.18: 3 levels of lamellae (I, II, III) are defined. R = bald retina, ON = optic nerve, BP = Bergmeister’s papilla. Box depicts transition from lamellar area to bald retina. Box
corresponds with Fig. 10.20.
Fig. 10.20 Magnification of box in Figure 10.19 depicting a thick membrane-like structure. The collagen fibers are oriented in the long axis of the membrane. A relatively bald retina lies beneath it [38].
5. The combination of compressed remnants of cortical vitreous on the retinal surface and cells in the vitreous after intraocular gas will provide conditions for the following course:
– The development of PVR and new tears.
Consequently:
6. The complications at the vitreoretinal interface after an intraocular gas injection are significantly greater than those after an extraocular tamponade.
10.3.2.3 Conclusion
The use of gas as a tamponade for a retinal break has the followingadvantages:
The injection of gas into the vitreous implies a minimal procedure.
Another advantage of the perfluorocarbon gases is that they have an expansion potential and, as a consequence, make feasible a nondrainage procedure. Depending on the length of the carbon chain, the gas can acquire different volumes that can be pre-calculated and selected for various retinal problems.
In addition, the perfluorocarbon gases provide a spectrum of longevity for an intraocular tamponade, extending from days to weeks.
Another advantage of an intraocular gas bubble is that it leaves the eye spontaneously by going into solution. As a result—in contrast to silicone oil—a second procedure is not necessary to remove the gas.
But most important of all is the buoyancy of an intraocular gas bubble. In this respect it is superior to any other intraocular tamponade: The buoyancy of a gas bubble is 16 to 17 times greater than that of silicone oil.
However, there are undesirable side effects to an intraocular gas bubble:
Whenever you decide upon a gas tamponade, you should keep in mind the biological and histological changes it will induce. There will be a break down of the blood aqueous barrier, an infiltration of cells into the vitreous after 1 to 2 days, and a compression of torn and disorganized cortical vitreous on the surface of the retina.
In this way a gas injection might initiate an irreversible biological process in an uncomplicated detachment:
– The development of PVR in 4%
– New breaks in 15% of eyes [23] (see Chapter 11.3, pp. 275–279).
As a result, if you have to treat an uncomplicated detachment, in which neither the size nor the location of the break presents a problem for its tamponade;
– Segmental buckling (sponge, balloon) without drainage, an extraocular approach, provides the procedure with a lesser morbidity.
Another complication which might develop when using an intraocular gas bubble is the development of a cataract. It can be prevented if ⅓ of the lens is kept free of the gas bubble. If more gas is needed then the patient will have to tilt the head to provide an adequate metabolic exchange via the back of the lens.
The following complication, an increase in intraocular pressure, should not occur when using an intraocular gas bubble. How will you prevent this complication?
– Before injecting an undiluted perfluorocarbon gas, make sure the eye does not have a diminished outflow.
– After injecting an expanding gas bubble, make sure that the central artery is patent and the patient’s head is positioned so that the chamber angle will not be compressed.
– A patient with gas in the eye cannot have general anesthesia with N2O.
This warning has also to be forwarded to any nonophthalmic surgeon your patient might go to.
– For all of these reasons, we think that the use of an intraocular gas tamponade should be reserved for retinal detachments with tears not suitable for external buckling.
– But if the gas technique succeeds in reattaching these difficult detachments, it may represent the next level of a minimal operation with a minimum of morbidity in comparison to other more invasive and more extensive alternatives.
10.4 Instrumentation and Surgical Technique
Hilton and Grizzard [21], Tornambe et al [39], and others inject gas into the vitreous in an outpatient setting or at the bedside of a recently operated patient. However, we recommend that an intraocular gas injection should be done under sterile conditions in the operating room.
10.4.1 Preoperative Phase
What do you have to prepare in the operating room before starting with the gas injection?
Check whether:
– The special gas you have selected for use is still available, i.e.,
– The tank contains enough gas for the scheduled operation.
Fig. 10.21 Set of gases, desirable in an operating room for retinal detachments: Xenon (short-acting gas to replace volume, e.g., after accidental drainage), 3 perfluorocarbon gases: CF4, C2F6 (half-life of 12 days, optimal gas), C3F8.
The armamentarium of gases that is recommended as being available in your operating room for the full spectrum of gas surgery is demonstrated in Figure 10.21. To the left Xenon, a gas which does not expand; it has a very short intraocular half-life of 6 hours, and after 16 hours 90% of the gas will have left the eye [40]. Because of its short duration in the eye, Xenon minimizes the cellular response in the vitreous and the subsequent complications.
Therefore, Xenon is an optimal gas:
It should be available when doing buckling to restore intraocular pressure in the situation of an inadvertent perforation.
To the right in Figure 10.21 are the 3 perfluorocarbon gases: CF4, C2F6 (the optimal gas for tamponade), and C3F8. We no longer use C4F10.
10.4.2 Intraoperative Phase
How do you prepare the patient’s eye for a gas injection?
In a similar manner to preparing for a buckle or balloon operation.
After cleaning the periorbital skin with soap and painting it with Betadine (povidone–iodine), the fornix is irrigated with a dilute Betadine solution. The face and lids are covered with an adhesive drape. The drape is incised between the lids and a wire speculum inserted.
What type of anesthesia will you apply?
No retrobulbar, but instead topical anesthesia (e.g., cocaine 5% or tetracaine 2%).
Why only topical anesthesia?
– To preserve the patient’s light perception during the gas injection. In doing so, the patient can tell you the moment when light perception is fading.
– This is essential to avoid an occlusion of the central retinal artery.
Cryopexy prior to a gas injection will reduce the intraocular pressure to some degree. Others apply a Honan balloon at 30 to 35 mmHg to reduce intraocular pressure. More intraocular space can be obtained by inserting a parabulbar balloon prior to the gas injection and expanding it until the central artery starts to pulsate. After about 2 hours the balloon volume can be withdrawn and a volume of 0.7 ml to 0.8 ml of gas can be injected into the vitreous without prior drainage and without closing the central artery (for more details, see Chapter 10.5, pp. 159–166).
You might begin the gas procedure by placing a traction suture through the insertion of the lateral rectus muscle or the muscle closest to the intended injection site. The traction suture will help you to stabilize the eye during the gas injection. Two sterile millipore filters are inserted into the tubing of the gas tank. The circulating nurse slowly opens the outlet valve of the gas tank until the surgeon can hear the soft flow of the gas (Fig. 10.22a). After that, he or she inserts a 2 ml syringe with 2 sterile millipore filters (Fig. 10.22b).
Fig. 10.22a Preparation of gas for intraocular injection: Open the outlet valve of the gas tank until the surgeon can hear the flow (more like a soft whistle) of the gas.
Fig. 10.22b Insert a 2 ml Luer syringe with 2 sterile millipore filters.
Fig. 10.22c Let the slowly outstreaming gas displace the plunger of the syringe to the desired volume (do not actively withdraw the gas because otherwise air might be aspirated via the connecting parts). Repeat this to remove air that might be present in the “dead space” of tubing.
Fig. 10.22d The syringe ready for intraocular gas injection: It is filled with gas, covered with one millipore filter and a shortened (5 mm) 30-gauge needle. To keep the needle sterile, it is covered with a cotton swab.
Be careful that the pressure of the outstreaming gas is not too high.
You should not withdraw the gas with the syringe, but the pressure of the outstreaming gas should displace the plunger of the syringe to the desired volume (Fig. 10.22c). With the syringe filled with gas, disconnect and push out a small amount of gas to clear the air present in the end of the syringe and needle (30-gauge) that you will have placed on the syringe. To keep the needle sterile and for its protection, it is covered with a cotton swab (Fig. 10.22d).
Fig. 10.23 Shortened 30-gauge needle for intraocular gas injection: A piece of silicone tubing (see arrow) is slid over the needle to reduce its effective length to 5 mm.
To avoid the needle penetrating the eye too deeply when injecting the gas, how should you proceed?
Use a short 30-gauge needle with a length of only 5 mm. However, the commercially available 30-gauge needles have a length of 12 mm.
How can such a needle be shortened?
Slide a piece of silicone tubing (7 mm long) over the needle to obtain an effective length of 5 mm (Fig. 10.23).
Where should you enter the eye with the needle to avoid injury to the lens or retina?
After cauterization, enter the eye 4 mm from the limbus (emmetropic eye) and direct the needle towards the center of the vitreous.
How should you perform the injection itself?
It is important that the gas injection is done with the tip of the needle under constant observation.
Fig. 10.24 Intraocular injection of gas via the pars plana: To prevent the complication of “gas under the retina,” the tip of the needle has to be through the ciliary epithelium and through a proliferative membrane that might be adherent to the internal surface of the ciliary epithelium. If necessary, drill with the tip of the needle through the membrane, inject a small gas bubble and observe whether the bubble ascends towards the back of the lens. Only then can you start with the intraocular gas injection.
Why?
To be certain that the tip of the needle has gone through the ciliary epithelium and into the vitreous and is not under a transparent proliferative membrane that might be adherent to the internal surface of the ciliary epithelium (Fig. 10.24).
Why is this so important?
Because if gas is injected under a membrane, the gas will break through the ora serrata and enter the sub-retinal space:
Gas under the retina is a serious complication!
To avoid this complication of gas injection, observe the needle tip and look for a membrane tented on the needle tip. If it is present, drill through it by rotating the syringe and the tip of the needle. Then inject a small gas bubble and observe whether the bubble ascends towards the back of the lens.
– If this happens, you are through the membrane.
– You can start the gas injection.
But how do you obtain a single large gas bubble instead of so-called fish eggs?
To begin with, rotate the eye so that the injection site is at the highest level when you inject the gas. Be certain that the assistant keeps the tip of the needle within the gas bubble for the duration of the injection. If needed, the assistant might have to withdraw the tip of the needle for 1 to 2 mm, however, only under ophthalmoscopic control by you.
When do you stop the injection?
Before the central artery is closed:
– Inject the gas very slowly. Your patient, having been informed to watch out for the moment that light perception will dim, will suddenly say:
“It’s getting dark.”
– At that very moment you will stop your injection.
During a gas injection the interaction between patient and surgeon is vital to prevent the central artery from closing.
– This is only possible if the gas operation is done under topical anesthesia.
What should be done, if you have injected too much gas, the central artery is closed off, and the patient’s light perception gone?
Reflect and do not over-react:
– At this point many surgeons would apply a paracentesis, but this would risk vitreous incarceration in an aphakic eye or would be ineffective.
– Instead, with the needle still within the gas bubble, let the assistant aspirate under your ophthalmoscopic control until the patient says: “Light is coming back.”
– Then withdraw a little more of the gas and re-inject it during the removal of the needle from the eye to avoid the risk of vitreous incarceration in the injection site.
The central artery should not be closed off for longer than 5 minutes;
7 minutes should be a rare exception.
However, it should be mentioned that some surgeons permit up to 10 minutes.
Experiments on monkeys demonstrated that it is possible to close the central artery for 99 minutes and still retain a functioning retina. But these were young animals with no retinal degenerations or retinal detachment. This is the reason why we try to close off a central artery for no longer than 5 to 7 minutes.
How should you withdraw the tip of the needle without gas bubbles escaping at the injection site?
Prior to removal of the needle, you turn the injection site from the superior to a more inferior position by the one traction suture. During the moment of withdrawal of the needle you might close up the injection site in addition with a wet Q-tip.
To be certain again that the central artery is open, examine it after the needle is withdrawn.
The injection of an expanding gas under general anesthesia requires special precautions: Why?
Nitrous oxide (N2O, a soluble anesthetic gas, will increase the gas bubble volume during general anesthesia if the patient has SF6, for example, in the eye.
– N2O which is 100 times more soluble than SF6 will cause an expansion of a SF6 bubble by 3 times its volume in 20 minutes.
– As a result, N2O should be avoided as a general anesthetic in patients who have an intraocular gas bubble.
– It is your responsibility to inform the anesthesiolo-gist about this risk.
Let us summarize the complications you might be confronted with when performing a gas injection:
– Avoid injury to the lens by directing the tip of the needle towards the center of the vitreous, and use a 30-gauge needle of 5 mm in length or one shortened by a silicone tube over part of it.
– Avoid injecting gas under a ciliary body membrane and the retina by ascertaining that the first small gas bubble from the tip of the needle ascends within the vitreous. If a membrane is present, drill through it with the tip of your needle before injecting.
– Avoid the formation of fish eggs, i.e., stabilize the tip of the needle and keep it within the gas bubble during the injection.
– Avoid closing off of the central retinal artery, i.e., carry out the procedure under topical anesthesia and inform the patient to alert you when light perception is fading.
After the injection of 0.3 ml of gas you should check the central retinal artery for pulsation, because 0.3 ml is the maximal volume to be injected without drainage unless there has been a preceding decompression maneuver (e.g., balloon–gas procedure).
– Avoid the escape of gas bubbles during withdrawal of the needle, i.e., rotate the injection site from a superior to a more inferior position.
10.4.3 Postoperative Phase
For the patient with a gas bubble in the eye, especially an expanding gas,
Correct postoperative positioning is mandatory to avoid:
– Closing off of the anterior chamber angle, and thus causing an abrupt rise in intraocular pressure.
– Passage of gas under the posterior edge of a giant tear until the edge is reattached by a special maneuver (see Chapter 10.6.1, pp. 167–212).
– Avoid allowing more than ⅓ of the lens to be covered by the gas bubble. Alternatively, if a larger gas bubble is present, provide a metabolic exchange via the back of the lens by positioning the patient’s head forward.
Otherwise you might end up with lens opacities that preclude observation of the retina and subsequent laser treatment. Eye drops are administered postoperatively, i.e., an antibiotic and atropine 1% (no ointments!).
Why only eye drops postoperatively?
Because in a patient with gas in the eye the cornea should be clear and ready for a fundus examination at any time.
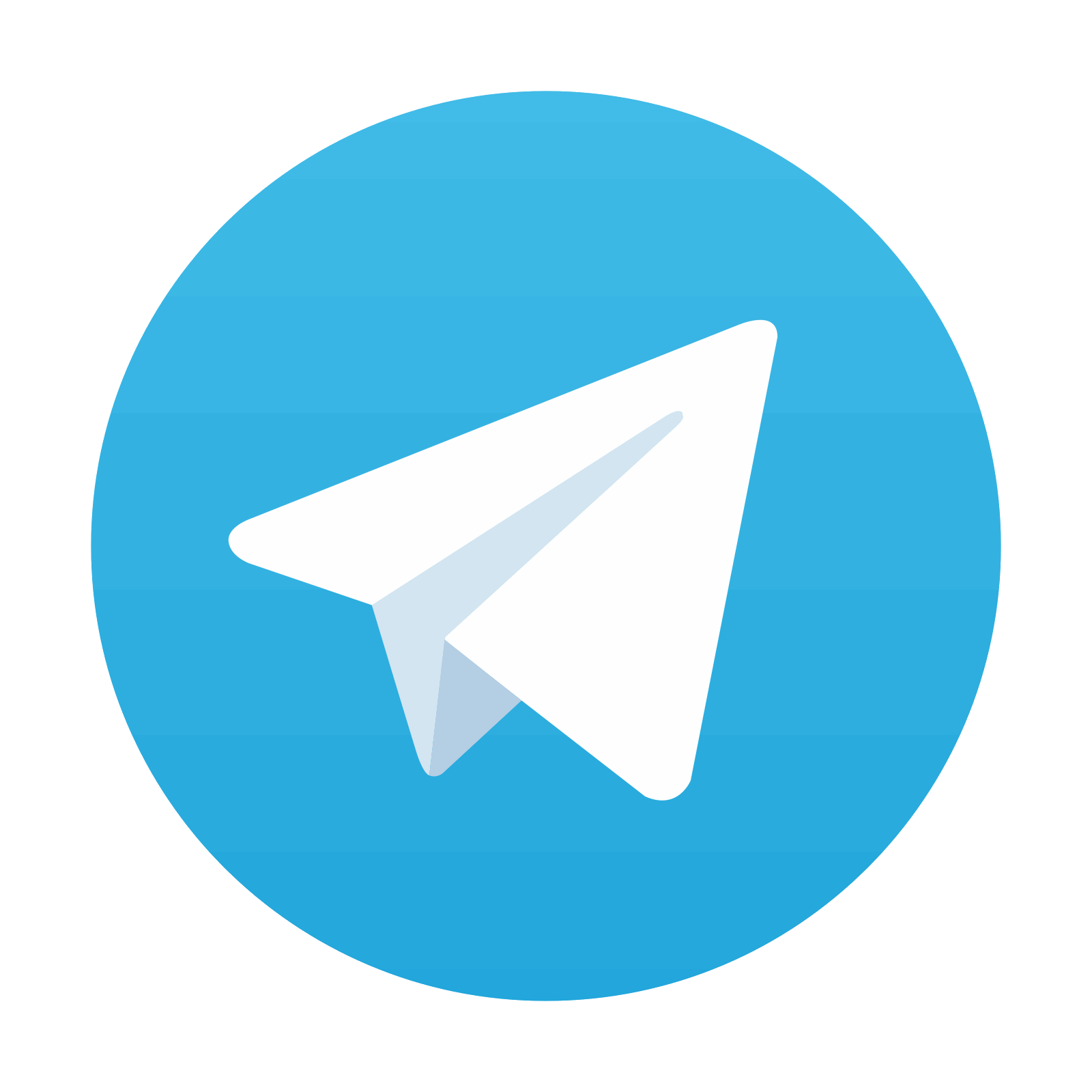