(1)
University of Sydney, Sydney, Australia
Overview
1.
The visual system in a changing environment
The visual system responds to variation in light over time, allowing instantaneous interpretation of a rapidly changing environment.
It concentrates on useful information (e.g., contrasting boundaries of objects, temporal changes in location and magnitude) and discards irrelevant features.
Successive retinal images are stored, integrated, differentiated, and erased, resulting in the perception of apparently stable scenes.
Temporal responsiveness varies between scotopic and photopic conditions.
2.
Temporal resolution of stimuli
The visual system is only able to detect stimuli at finite time intervals.
Stimuli presented closer together than this finite time are treated as a single stimulus event.
The time at which two discrete stimuli are just detected is the temporal threshold or limit of temporal resolution.
Temporal Summation and the Critical Duration (Tc)
The duration of a light stimulus influences its:
(a)
Ease of visibility
(b)
Subjective appearance
3.
Temporal summation
Temporal summation describes the influence of stimulus duration on its visibility [1].
It occurs because a longer duration stimulus emits more photons over time than a brief stimulus of the same intensity. Multiple, sequential photons may be required for the light to be seen.
4.
Critical duration (Fig. 22.1)
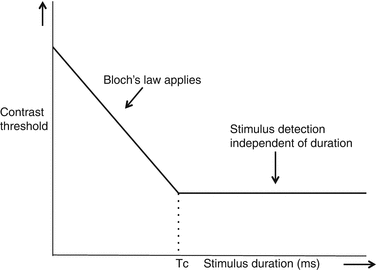
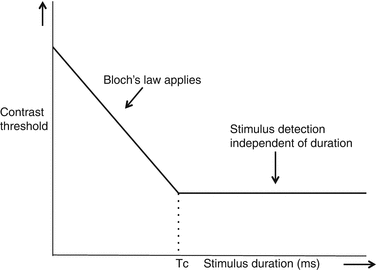
Fig. 22.1
Schematic representation of stimulus duration influencing detection threshold: Bloch’s law and critical duration (Tc)
The critical duration (Tc) is the maximum time period over which temporal summation can occur.
Beyond Tc temporal summation ceases and detection depends on luminance alone.
For flashes briefer than Tc, the chance of visual detection of a light source is based on luminance (B) and duration (t), described in Bloch’s law [2]:
where C is constant
5.
Factors that influence the critical duration (Tc)
6.
Critical duration and assessment of contrast threshold
Thresholds to light detection are measured using flashes longer than Tc, so that flash duration is removed as a variable that may influence threshold.
This is important in static perimetric testing: each test stimulus must be present for longer than Tc (see Chap. 23, The Visual Field).
The Broca-Sulzer Effect (Fig. 22.2)
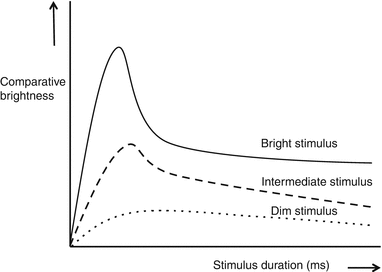
Fig. 22.2
The Broca-Sulzer effect
Brief stimuli appear subjectively brighter than a longer flash of the same luminance; this is the Broca–Sulzer effect [15, 16].
As flash duration increases, there is a transient peak brightness at 50–100 ms.
For stimuli of duration longer than this, subjective brightness is decreased and reaches a plateau of subjective luminance [17].
The Broca-Sulzer effect is most apparent for bright flashes and is less significant for dim stimuli.
Troxler’s Phenomenon
Troxler’s phenomenon is a time-dependant visual adaptive process (see Chap. 21, Luminance Range for Vision).
A fixed retinal image fades from perception in a few seconds; it is restored by a slight movement of the image or the eye [18, 19].
It demonstrates the visual system’s reliance on temporal as well as spatial contrast to capture visual information.
Troxler’s phenomenon is a neural, not photochemical, phenomenon.
The decay is slower with larger, brighter, and more central images [20].
Visual Fixation
1.
2.
Saccadic suppression
Saccades are brief voluntary conjugate eye movements to bring an object of regard into central view (see Chap. 18, Neural Control of Eye Movements) [23].
During saccades (10–80 ms) visual processing is temporarily suppressed and the visual system is unresponsive to visual input, preventing the sensation of movement and blur [24, 25].
Between saccades the eyes make fixed pauses of brief duration (200–300 ms) to take in visual information, during which suppression is released [26].
Critical Flicker Frequency
1.
Definition
When light is turned on and off repeatedly, it appears to flicker.
As the speed of the on/off cycle increases, we eventually perceive the flashes as a single fused light.
The critical flicker frequency (CFF) is the transition point of perception from flicker to continuous light.
The CFF is a measure of the temporal acuity (resolving power) of the visual system.
2.
Factors that influence the critical flicker frequency
(i)
(ii)
Spectral composition
When monochromatic light sources are used, the CFF increases linearly with log luminance, according to the Ferry-Porter law.
The increase in CFF with luminance is greater for green light and less for red (Fig. 22.3B) [30, 31].
This may be due to differences in signal processing speed between green and red cone pathways.
This is because short-wavelength-sensitive cones (and rods) have slower processing speeds than medium-wavelength-sensitive and long-wavelength-sensitive cones.
(iii)
(iv)
(v)
Background luminance/adaptive state
3.
The effects of flicker on perception
(i)
Brucke-brightness enhancement effect
(ii)
Talbot-Plateau law
The Talbot–Plateau law describes the brightness of an intermittent light source with a frequency above the CFF [41, 42].
This law states that above CFF, subjectively fused intermittent light and objectively steady light (of equal color and brightness) will have precisely the same luminance.
For example, a flickering stimulus at twice the CFF needs to be twice as bright as a steady stimulus.
Temporal Contrast Sensitivity
Temporal contrast sensitivity describes how temporal differences in visual input can be resolved.
It is typically measured as a subject’s CFF and varying contrast levels.
It is primarily related to the frequency of the flicker; however, it is also influenced by stimulus brightness, size, retinal eccentricity, and state of adaptation.
A curve describing human temporal contrast relative to temporal frequency can be derived, with:
(a)
y–axis: log temporal contrast sensitivity
(b)
Get Clinical Tree app for offline access
x–axis: log temporal frequency (Fig. 22.5) [43]
Fig. 22.5
The temporal contrast sensitivity curveStay updated, free articles. Join our Telegram channel
Full access? Get Clinical Tree