Temporal bone fractures occur from high-energy mechanisms, typically but not limited to motor vehicle accidents. However, as the automotive industry continues to introduce improved safety measures, violence and falls account for a larger proportion of cases. Given the great forces involved, temporal bone fractures rarely occur in isolation and initial evaluation must focus on the fundamental ABCs of Advanced Trauma Life Support with special attention to Glasgow Coma Scale, intracranial injury, and cervical spine injury. Subsequent evaluation relies on physical examination, high-resolution CT, and electrodiagnostic testing to address the neurotologic consequences of temporal bone fracture, including cerebrospinal fluid leak, facial nerve injury, and injury to the peripheral hearing and balance organs. Management algorithms must address immediate (eg, ABCs, neurosurgical issues), short-term (eg, cerebrospinal fluid leak, facial nerve injury, hearing loss), long-term (eg, facial nerve injury, hearing loss, vestibular injury), and delayed (eg, encephalocele, cholesteatoma, late meningitis) issues. This article reviews the current state of temporal bone fracture evaluation and management with special attention to mechanisms of injury, clinical presentations and emergency evaluation, and diagnostic workup, including the evolution of radiographic fracture classification systems and electrodiagnostic testing. Discussion of treatment approaches address management of immediate, short-term, long-term, and delayed complications.
Epidemiology and historical background
Approximately 4% to 30% of head injuries involve a fracture of the cranial base, including 18 to 40% with temporal bone involvement . Most of these fractures are unilateral, with bilateral fractures reported in 9% to 20% . These patients frequently present with multiple injuries of varying severity. Although the temporal bone fracture and related sequelae may not represent the patient’s most immediately threatening problems, early involvement of the otolaryngologist/neurotologist in evaluation and management can improve long-term functional outcome.
Clinical relevance
The importance of temporal bone fractures relates not only to functional deficits from injury to structures within the temporal bone but also to regional and intracranial complications. The more common sequelae of temporal bone fracture include injury to the facial nerve with facial paresis or paralysis; disturbance of the cochleovestibular apparatus with associated sensorineural hearing loss, conductive hearing loss, balance disturbance, tinnitus, and vertigo; and cerebrospinal fluid (CSF) leak through the fracture lines . Less common otologic manifestations include conductive hearing loss secondary to ossicular chain damage, sympathetic sensorineural hearing loss, perilymphatic fistula, posttraumatic endolymphatic hydrops, cholesteatoma, meningocele/encephalocele, and late otogenic meningitis .
Associated injuries to adjacent structures include palsies of other cranial nerves, especially cranial nerves VI and IX through XI, and vascular injury, including carotid artery and sigmoid sinus injuries . These patients also frequently experience critical intracranial injuries that often dictate their early management, including subarachnoid hemorrhage, subdural hemorrhage, brain contusion, and cerebral edema .
Classification systems
Historically, temporal bone fractures have been classified as either transverse or longitudinal based on Ulrich’s observations in 1926 and then experiments performed on cadaveric human heads in the 1940s ( Figs. 1 and 2 ) . Based on this initial evidence, and confirmed in clinical series, 80% to 90% of temporal bone fractures are longitudinal and 10% to 20% are transverse .
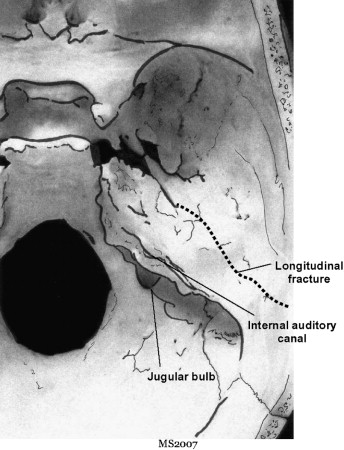
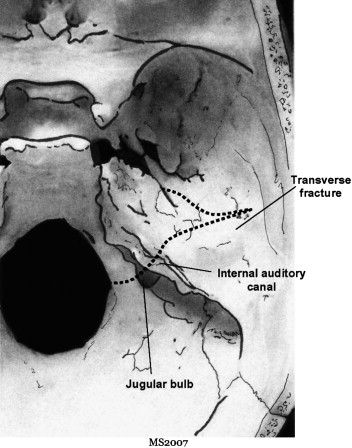
However, changing patterns of injury over time and the failure of longitudinal and transverse to accurately describe many clinically observed fractures led to the addition of oblique and mixed descriptors ( Fig. 3 ) . Despite improved accuracy in describing the fracture geometry, this expanded terminology still failed to correlate descriptors and clinical sequelae. To address this shortcoming, several authors over the past decade introduced new classification systems in attempt to better correlate fracture geometry with clinical outcomes . Of these, the classification as otic capsule–violating (OCV) versus otic capsule–sparing (OCS) has been the most predictive of clinical outcome .
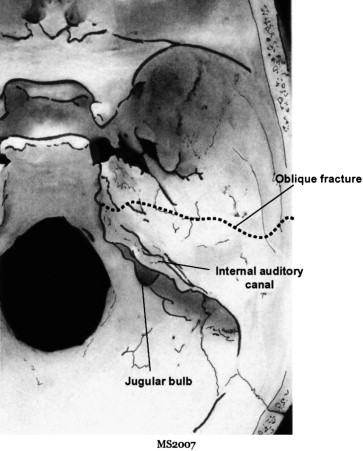
Etiology
The pattern of injuries resulting in temporal bone fracture has evolved over time. With the advent of improved automobile safety technology, the number of fractures from motor vehicle accidents has decreased . In contrast, increasing rates of violent crimes have led to increasing rates of temporal bone injury from assault, including those from blunt instruments and firearms . In addition, although overall temporal bone fracture incidence has trended down, advances in pre- and in-hospital trauma care have led to more patients who have temporal bone fractures surviving their initial injury than previously .
In contemporary series, the primary mechanisms of injury include motor vehicle accident (12%–47%), assault (10%–37%), falls (16%–40%), and gunshot wound (3%–33%) . In a subset analysis of 115 patients who had facial paralysis caused by temporal bone fracture, the mechanisms included road traffic (63%), industrial (7%), and sports accidents (3%).
Two unique subpopulations in which these injuries are seen include (1) large urban inner-city hospital settings where blunt assault and gunshot wounds account for most injuries, with a recent trend toward increasing numbers of violence-related temporal bone fractures , and (2) the pediatric population that shows a bimodal distribution centered at 3 and 12 years, with falls and motor vehicle accident most prominent in the younger children, and biking accidents and blows to the head more prominent in older children . In larger series, children account for 8% to 22% of patients who have temporal bone fracture . Of fractures inducing facial paralysis, 9% comprise children, with most injuries being associated with domestic accidents .
Anatomic and mechanical factors
Research models
In the 1940s, Gurdjian and Webster extensively studied deformation of the human skull secondary to low-velocity impacts using the “stresscoat” technique. Although they did not have the goal of studying fractures, they showed how force applied to a given point is distributed throughout the cranial skeleton, which led to an understanding of the relationship between point of impact and orientation of fractures.
To study the issue of fracture secondary to automobile accidents, Travis and colleagues conducted a set of experiments on human cadavers involving piston impact to the temporoparietal region and sled tests designed to simulate a side impact automobile accident. In this study, the average force leading to temporal bone fracture was 1875 lb with an average speed of 25 mph. In all cases, this force was enough to produce severe injury to the temporal bone and intracranial contents.
In 2004, Yoganandan and Pintar summarized 150 years of experimental analysis of temporoparietal impact biomechanics. Key findings include :
- 1.
The human head deforms before actual fracture in compression studies.
- 2.
The mechanical properties of the skull do not depreciate significantly with age after maturity, in contrast to other bones such as hips.
- 3.
The temporoparietal region requires as much if not more force to fracture than the frontal region.
Clinical specimen dissections
In 2005, Wysocki conducted a dissection study on 100 temporal bones obtained from forensic autopsy cases. Although the observed fractures and associated injury patterns did not correlate with the mechanism of injury, the study showed the anatomic details of the injury complex as it occurs in real rather than simulated patients. However, possible selection bias must be considered, in that forensics cases may not accurately reflect the pattern of injury in the general population, and many of the specimens originated from cases that did not survive the initial injury and therefore probably represent more severe levels of injury .
The detailed analysis showed a fracture pattern of 82% longitudinal, 11% transverse, and 7% mixed, correlating with the reported literature. Injury to the bony facial canal was shown in 44% of bones with longitudinal fractures and 64% of those with transverse fractures. In cases of longitudinal fracture, most facial nerve injuries occurred in the region of the genu, and transection of the nerve was rarely seen. In contrast, in bones with transverse fractures, the nerve injury predominantly occurred in the labyrinthine portion and was usually associated with complete transection of the facial nerve. Ossicular chain injuries consisted of dislocation at the incudomallear joint in 51% and the incudostapedial joint in 57% of patients, malleus fracture in 8%, and stapes fracture in 17%. No bones showed incus fractures. Vascular injuries included injury to the jugular bulb (21%) in association with all fracture orientations, and carotid canal fracture in 52%, although arterial wall injury occurred in only one bone .
Anatomic and mechanical factors
Research models
In the 1940s, Gurdjian and Webster extensively studied deformation of the human skull secondary to low-velocity impacts using the “stresscoat” technique. Although they did not have the goal of studying fractures, they showed how force applied to a given point is distributed throughout the cranial skeleton, which led to an understanding of the relationship between point of impact and orientation of fractures.
To study the issue of fracture secondary to automobile accidents, Travis and colleagues conducted a set of experiments on human cadavers involving piston impact to the temporoparietal region and sled tests designed to simulate a side impact automobile accident. In this study, the average force leading to temporal bone fracture was 1875 lb with an average speed of 25 mph. In all cases, this force was enough to produce severe injury to the temporal bone and intracranial contents.
In 2004, Yoganandan and Pintar summarized 150 years of experimental analysis of temporoparietal impact biomechanics. Key findings include :
- 1.
The human head deforms before actual fracture in compression studies.
- 2.
The mechanical properties of the skull do not depreciate significantly with age after maturity, in contrast to other bones such as hips.
- 3.
The temporoparietal region requires as much if not more force to fracture than the frontal region.
Clinical specimen dissections
In 2005, Wysocki conducted a dissection study on 100 temporal bones obtained from forensic autopsy cases. Although the observed fractures and associated injury patterns did not correlate with the mechanism of injury, the study showed the anatomic details of the injury complex as it occurs in real rather than simulated patients. However, possible selection bias must be considered, in that forensics cases may not accurately reflect the pattern of injury in the general population, and many of the specimens originated from cases that did not survive the initial injury and therefore probably represent more severe levels of injury .
The detailed analysis showed a fracture pattern of 82% longitudinal, 11% transverse, and 7% mixed, correlating with the reported literature. Injury to the bony facial canal was shown in 44% of bones with longitudinal fractures and 64% of those with transverse fractures. In cases of longitudinal fracture, most facial nerve injuries occurred in the region of the genu, and transection of the nerve was rarely seen. In contrast, in bones with transverse fractures, the nerve injury predominantly occurred in the labyrinthine portion and was usually associated with complete transection of the facial nerve. Ossicular chain injuries consisted of dislocation at the incudomallear joint in 51% and the incudostapedial joint in 57% of patients, malleus fracture in 8%, and stapes fracture in 17%. No bones showed incus fractures. Vascular injuries included injury to the jugular bulb (21%) in association with all fracture orientations, and carotid canal fracture in 52%, although arterial wall injury occurred in only one bone .
Radiology
Modalities
Imaging studies play a crucial role in the contemporary evaluation and management of temporal bone fractures. The evaluation of temporal bone fracture requires assessment of not only the temporal bone itself but also the surrounding structures, including the intracranial contents and cervical spine. Before high-resolution CT (HRCT), temporal bone fracture was diagnosed predominately based on clinical grounds. Typical acute clinical indicators of temporal bone fracture include hemorrhagic otorrhea, hemotympanum, tympanic membrane perforation, vertigo, hearing loss, facial nerve palsy, nystagmus, and Battle’s sign . Late indicators include subtle findings of wrinkles or folds in the tympanic membrane or a flaccid tympanic membrane, bony step-offs in the annulus or canal wall, and unexplained hearing loss with a history of head trauma .
Noncontrasted HRCT of the temporal bone, with cuts ideally no more than 1.5 mm, is the diagnostic imaging standard for evaluating temporal bone fractures. HRCT provides excellent depiction of bony anatomy (including axial) and reconstructions of coronal and sagittal images from the raw axial data. In addition to delineating fracture anatomy, it also allows evaluation of the facial canal, ossicular chain, otic capsule, carotid canal, and middle cranial fossa floor. Furthermore, contemporary scanners are capable of rapid imaging of critically ill patients with minimal requirements for patient manipulation .
Compared with physical examination, HRCT has greater sensitivity. On correlating physical examination findings with HRCT, radiographically evident temporal bone fractures were missed on physical examination in 14% to 35% of patients. This finding has led some authors to recommend HRCT of all patients who have head trauma .
For evaluating intracranial contents, and for nerve palsy not explained using HRCT, MRI remains the optimal choice once the patient has been stabilized enough to allow for the longer examination time . MRI also plays a role in preoperative evaluation for patients requiring surgical intervention for temporal bone fractures, especially for middle cranial fossa approaches that entail temporal lobe retraction . One series showed a 46% incidence of ipsilateral temporal lobe contusion in patients who needed surgical intervention for facial nerve injury secondary to temporal bone fracture. None of the temporal lobe contusions were clinically evident, and MRI showed four temporal lobe contusions and two subdural hematomas not identified on HRCT . MRI also differentiates between herniation of intracranial contents into the mastoid and a fluid-filled mastoid, which frequently cannot be distinguished on HRCT .
Additional imaging modalities include traditional four-vessel arteriography, which remains the gold standard for definitive evaluation of intracranial vascular injury, including the temporal portion of the carotid artery, and CT metrizamide cisternography, which can be helpful in localizing otogenic CSF leaks.
Classification systems
The division of temporal bone fractures into longitudinal and transverse has been attributed to Ulrich who described these fracture orientations in 1926. This classification system was reinforced in the 1940s when Gurdjian and Webster used a cadaveric model that supported the classification into longitudinal (80%) and transverse (20%) orientations, and which has been replicated in many clinical series . Longitudinal fractures are described as secondary to temporoparietal impact, leading to fractures extending from the squamosa through the posterosuperior bony external auditory canal, across the tegmen tympani and anterior to the labyrinth, terminating in the middle cranial fossa at the foramen spinosum (see Fig. 1 ; Fig. 4 ) . In contrast, transverse fractures result from fronto-occipital blows and course perpendicular to the long axis of the petrous pyramid from the foramen magnum through the posterior fossa, through the petrous pyramid, including the otic capsule, and into the middle cranial fossa (see Fig. 2 ; Fig. 5 ) .
However, in clinical practice this dichotomous system proved too limiting, with many fractures having mixed components. In some series, up to 62% to 90% of blunt trauma–induced fractures were more accurately described as mixed . In 1992 Ghorayeb and Yeakley proposed the inclusion of the oblique fracture to more accurately describe the three-dimensional anatomy of fracture planes based on the geometry of fractures observed in their series of 150 fractures, with oblique fractures accounting for 75% (see Fig. 3 ).
Although this classification allowed for more accurate description of fracture patterns, it failed to adequately correlate radiologic findings with clinical outcomes. To address this limitation, Kelly and Tami introduced the concept of OCS versus OCV as descriptors that better correlated with clinical sequelae of temporal bone fracture. In a detailed examination of this system, Dahiya and colleagues found a fourfold increase in CSF leak, a sevenfold increase in sensorineural hearing loss (SNHL) in OCV fractures, and a statistically significant association among OCV fractures, epidural hematoma, and subarachnoid hemorrhage.
Little and Kesser validated these findings, showing a 5-fold increase in facial nerve injury, a 25-fold increase in SNHL, and an 8-fold increase in CSF leak with OCV fractures. They also showed a correlation among OCV fracture and facial nerve paresis or paralysis, conductive hearing loss (CHL), and dizziness or balance dysfunction. In a large series of 820 temporal bone fractures, facial nerve paralysis occurred in 48% of OCV fractures versus only 6% in OCS fractures, and CSF leak occurred twice as frequently in the OCV fractures . In terms of fracture incidence, although some have reported a higher incidence of OVC fractures , the incidence is 2.5% to 5.6% in most series .
More recently, others have introduced a petrous versus nonpetrous fracture classification. Similar to OCS versus OCV, this system groups OCV and petrous apex fractures as “petrous,” and fractures involving the middle ear and/or mastoid as “nonpetrous.” Using this system, most complications occurred in the petrous group, whereas CHL was uniquely associated with the nonpetrous middle ear fracture group . Validation studies have not yet been reported.
Clinical presentations
Neurologic injury
Because of the tremendous forces required to produce a temporal bone fracture, patients frequently have multiple injuries, with associated nontemporal skull fractures (47%), maxillofacial fractures (21%), and orthopedic injuries outside the head and neck (16%) . Strict adherence to Advanced Trauma Life Support protocols is critical in treating these patients .
Many patients present with intracranial injury. In a series of 115 patients with facial paralysis secondary to temporal bone fracture, 33% presented with a Glasgow Coma Scale (GCS) score of less than 7 . In another series, the mean GCS was 12 (range 3–15), with 49% of patients showing mental status changes and 7% having limb paralysis . Despite improved pre- and in-hospital care, mortality related to neurologic sequelae in this population still runs as high as 10% , and for those who do survive, these severe injuries frequently have significant long-term consequences, with up to 16% of patients requiring institutional care beyond the initial acute management period .
Specific intracranial injuries encountered in the temporal bone fracture patient include subdural hematoma (21%), subarachnoid hemorrhage (23%), brain contusion (9%–46%), and tension pneumocephalus . In a series of 43 patients who had temporal bone fracture, 84% had at least one intracranial pathologic finding, of which 39% had two or more. Overall, 44% of these patients required open neurosurgical intervention, including 86% of those who had two or more findings .
Of particular importance in the initial evaluation of patients who have temporal bone fracture is adherence to cervical spine injury precautions. The general rate of cervical spine injury in the blunt trauma setting is 2% to 6% . Although they were not specifically evaluating patients who had temporal bone fracture, Diaz and colleagues reported on cervical spine injury in 1006 patients who had blunt trauma and altered mental status (average GCS of 12). In the setting of altered mental status, cervical spine injury was double the general population at 12%. Of the 116 patients who had cervical spine injuries, 17% had unstable fractures that required further intervention. This study further showed the superiority of HRCT in identifying cervical spine injury, with a missed fracture rate of only 1.7% compared with a miss rate of 52% for plain film radiography .
Facial nerve injury
The reported incidence of facial nerve paralysis after temporal bone fracture varies according to fracture pattern. Using the traditional classification system, facial nerve injury occurs in 10% to 25% of longitudinal fractures and 38% to 50% of transverse fractures . Brodie and Thompson reported an overall facial nerve injury rate of 7%, correlating with their low incidence of OCV fractures. Facial nerve injury in the pediatric population is much less common, with reports as low as 3%. This finding has been hypothetically attributed to the increased flexibility of the pediatric skull .
The timing of onset of facial paralysis has an important bearing on outcome. Immediate paralysis frequently indicates a transection of the nerve with loss of neural continuity, whereas delayed onset paralysis or paresis usually occurs in the context of an intact nerve and denotes the development of neural edema or expanding hematoma with neural compression inside a nonexpanding bony canal . Among 7% of patients who had temporal bone fracture with facial paralysis, 27% were immediate onset and 73% were delayed . Darrouzet and colleagues studied 115 patients who had temporal bone fracture with facial nerve injuries and found paresis in 23%, complete immediate paralysis in 52%, complete delayed paralysis in 15%, and 10% whose severe intracranial injuries precluded determination of paralysis onset.
Most injuries occur in the region of the geniculate ganglion or second genu . Nerve exploration showed the following lesions: geniculate ganglion in 66%, second genu in 20%, tympanic segment in 8%, and mastoid segment in 6%. Although 91% of patients showed only one injury, geniculate ganglion lesions occurred in conjunction with a separate lesion in the mastoid segment in 6%. The typical pathologic findings included contusion of the nerve, including edema and hematoma of the nerve sheath in 86% and nerve transection, either partial or complete, in 14% of the cases . Other series showed similar findings, with additional notation of fibrosis and bone spicules at the geniculate ganglion in up to 33% of patients .
Cerebrospinal fluid leak
The reported incidence of CSF leak in temporal bone fracture patients ranges from 11% to 45% . Most of these represent CSF otorrhea, but in a few cases, an intact tympanic membrane will shunt the fluid to the eustachian tube with resultant CSF rhinorrhea . In the acute phase, CSF is often mixed with blood and a high index of suspicion must be maintained for timely diagnosis. The presence of a halo sign, a reservoir sign when patients bend their heads, or clear fluid mixed with dried blood are often suggestive. In cases where the diagnosis is less obvious, fluid can be analyzed for beta-2 transferrin.
The presence of a leak has been correlated with the geometry of the temporal bone fracture with a two- to fourfold increased risk for OCV fractures . Brodie and Thompson reported an overall incidence of 18%, of whom 7% developed meningitis. Of these patients, 4% had additional CSF leaks secondary to concurrent anterior basilar skull fractures that also required surgical treatment.Meningitis was much more likely to occur in patients who had a CSF leak lasting more than 7 days and was more prevalent in the pediatric population (4/9 cases; 45%), although they only represented 3% of the study population .
Hearing loss
Hearing loss in patients who have temporal bone fracture may be immediate or delayed; may be transient, permanent or progressive; and may have a conductive, sensorineural, or mixed pattern . Reported incidence rates are 26% to 57% for CHL; 14% to 23% for SNHL, including 14% with complete SNHL; and 20% to 55% for mixed . Overall, approximately 24% of patients will have a subjective complaint of hearing loss . In a patient who had known SNHL after bilateral temporal bone fractures, microfractures involving the otic capsule were identified on histopathologic analysis of temporal bones. Although these fractures would escape detection on clinical imaging, these findings may underlie the phenomenon of cochlear concussion, wherein SNHL occurs without gross disruption of the hearing structures . Cochlear concussion was noted in 9% of 115 cases of facial nerve paralysis associated with temporal bone fracture . In an alert patient, a simple tuning fork test may give clues to the nature of the hearing loss. Audiograms are typically performed 3 to 6 weeks after the injury to allow enough time for resolution of hemotympanum, because middle ear fluid (CSF or blood) results in a conductive loss, and behavioral testing after resolution of the fluid will help determine the presence of ossicular discontinuity.
A unique form of delayed hearing loss is progressive SNHL in the contralateral uninjured ear presenting months to years after the initial trauma. Known as sympathetic hearing loss , this form of SNHL has a reported incidence of 1% to 11% in patients who have temporal bone fracture . This hearing loss has been shown to be associated with isolated autoimmunity to inner ear antigens . In theory, the cochleovestibular apparatus exists as an immunologically privileged site, and exposure of antigens after trauma is believed to sensitize the general immune system, leading to an autoimmune-mediated contralateral SNHL. Although these patients may initially experience response to steroid treatment, the hearing loss may still progress, in which case excellent response to cochlear implantation has been reported .
Other cranial nerve palsies
Depending on the fracture geometry, temporal bone fractures may result in palsies to cranial nerves other than the facial nerve, with a reported incidence of 7.8% . The jugular foramen syndrome consists of palsies of cranial nerves IX, X, and XI and occurs in petrous apex fractures that involve the jugular foramen or its surrounding structures . As with the facial nerve, the palsy may be immediate or delayed, with delayed onset portending a better prognosis . Darrouzet and colleagues reported a 2.6% incidence of lower cranial nerve palsy in three cases of jugular foramen hematoma.
Injury to the sixth cranial nerve occurs with an incidence of 5% to 7%, all of which occurred in fractures involving the petrous apex, and including three patients who had bilateral cranial nerve VI palsies associated with bilateral temporal bone fractures . This most frequently involves a stretch or impingement injury as the nerve enters Dorello’s canal where it passes under the petroclinoid ligament . Although fractures involving this area are likely to lead to a cranial nerve VI injury, this nerve, with its long intracranial course, is subject to stretch injury even in the absence of direct involvement by fracture. This injury is believed to occur during posterosuperior displacement of the brain during impact, causing temporary impingement of cranial nerve VI against the rigid petroclinoid ligament . As a rule, the patient will usually have spontaneous recovery between 2 weeks and 4 months from injury .
A cranial nerve V palsy has also been reported in a patient who had bilateral temporal bone fractures, bilateral cranial nerve VI palsies, and bilateral cranial nerve VII palsies. The mechanism is believed to be a stretch/impingement injury at the point where the sensory root enters the dural foramen at the entrance of Meckel’s cave, which is located just inferior to the margin of the petrous pyramid . Two months after injury and exploration for the cranial nerve VII palsies, the affected trigeminal nerve had regained function .
Vascular injury
In an anatomic dissection of 100 temporal bone fractures from autopsy specimens, all injuries involving either the sigmoid groove or the jugular fossa resulted in violation of vessel wall. In contrast, although fractures of the bony carotid canal were common, they rarely disrupted the vessel wall . In a clinical report involving a patient who had bilateral temporal bone fractures, the fracture line extended to the sigmoid sinus, which was found to be thrombosed at exploration for a facial nerve palsy . In this setting of aseptic thrombus, exploration is not usually warranted for the sigmoid sinus thrombus alone, because this region has a rich collateral drainage and therefore presents little threat .
With respect to the carotid artery, internal carotid artery dissection with carotido-cavernous fistulae was observed in 1% to 3% of cases . Resnick and colleagues examined the relationship between basicranial fracture and carotid artery injury in 230 patients, and found that 55 (24%) patients had fractures involving the carotid canal, 6 (11%) of whom had vascular complications directly related to the intracranial carotid injury. In contrast, only 4 (2.3%) of 175 in the noncarotid canal fracture group sustained vascular complications. Although 62% of fractures occurred at the junction of the lacerum and cavernous portions of the canal, most vascular injuries occurred in fractures of the petrous segment (67% of injuries), with 25% of patients with petrous fractures sustaining a carotid injury .
Vertigo
Vertigo frequently occurs after temporal bone trauma and may be secondary to either vestibular concussion in OCS situations or vestibular destruction in OCV settings . The vertigo is usually self-limiting and resolves within 6 to 12 months from central adaptation. Perilymph fistulae can also cause vertigo and may occur with injury to the otic capsule. Although most patients who experience disruption of the otic capsule present with profound SNHL and no vestibular function, some will retain some degree of residual inner ear function. These patients typically present with immediate postinjury fluctuating hearing loss and vestibular symptoms that may persist for months .
Another cause of vertigo after temporal bone fracture is posttraumatic endolymphatic hydrops. These patients present with aural fullness, tinnitus, fluctuating hearing loss, and vertigo similar to patients who have Meniere’s disease . The mechanism is theorized to be a disruption in the endolymph/perilymph balance secondary to an injury to either the membranous labyrinthine duct or the endolymphatic fluid drainage pathways, which occurs in cases of temporal bone fracture through the vestibular aqueduct .
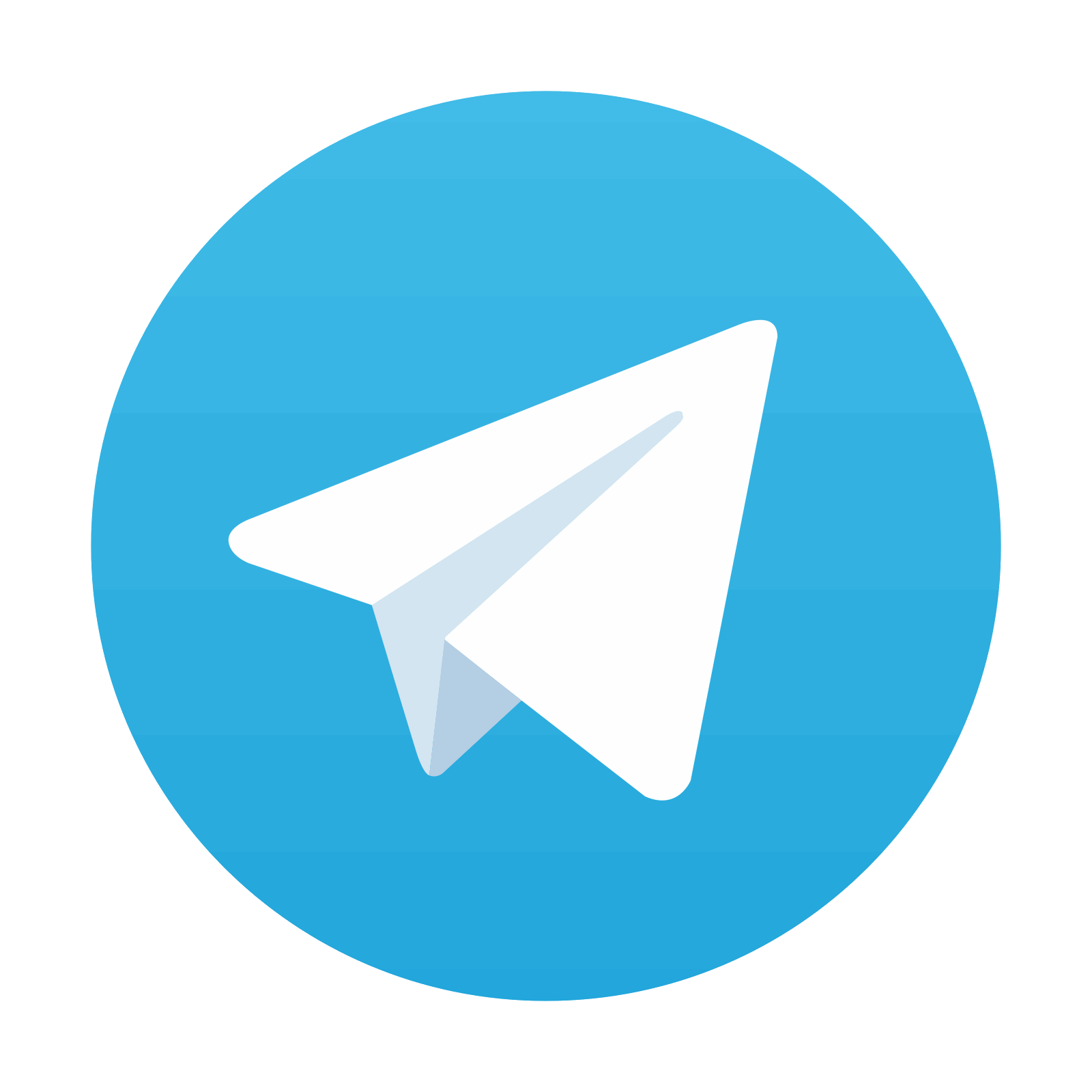
Stay updated, free articles. Join our Telegram channel

Full access? Get Clinical Tree
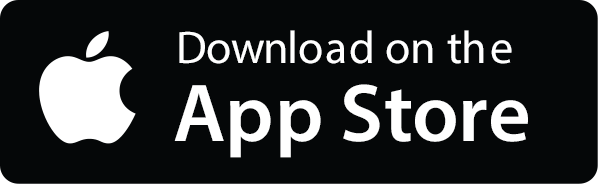
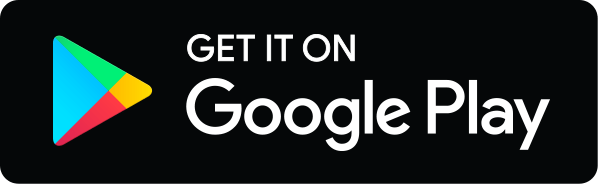