Category
Systolic BP (mmHg)
Diastolic BP (mmHg)
Hypertension
Stage I
140–159
or 90–99
Stage II
160–179
or 100–109
Stage III
≥180
or ≥110
Prehypertension
130–139
or 85–89
Normal
<130
and <85
Optimal
<120
and <80
The JNC-VII report also determined that the risk of cardiovascular complications secondary to arterial hypertension escalates in a graded, continuous, and predictable manner with no threshold value. The cardiovascular event risk doubles with each increment of 20/10 mmHg, beginning at a pressure of 115/75 mmHg. It also de-emphasized diastolic blood pressure and found that systolic blood pressure and pulse pressure is more important, particularly in those over 50 years of age. The reclassification of “normal” blood pressure values by the JNC-VI and VII reports, and the emphasis for tighter blood pressure control with the virtual elimination of a threshold blood pressure value distinguishing hypertensive from normotensive, represents a distillation of emerging data pooled from various clinical as well as epidemiologic studies. Vasan et al. showed that high-normal blood pressure was associated with a risk-factor-adjusted hazard ratio of 2.5 as compared to optimal blood pressure [13]. In the Framingham Offspring Study, patients with high-normal blood pressure had a two- to threefold increased risk of developing a coronary event as compared to those with optimal blood pressure [14].
At diagnosis, identifying the stage of hypertension with assessment for target organ damage is now the standard. As stated previously, emphasis for more intensive treatment of hypertension is in those patients who are at higher risk since they are the group with greater expected benefit, specifically those with manifest target organ damage [11]. Data from multiple studies including the Hypertension Optimal Treatment Study (HOTS) and the United Kingdom Prospective Diabetes Study (UKPDS) recommend lower target pressures in diabetics since diabetes is considered a coronary risk equivalent [15–17]. Even tighter control is recommended (target blood pressure <125/75) for diabetics with elevated creatinine, microalbuminuria, or overt proteinuria, especially those with greater than 1 g/day of proteinuria [18–20].
Analogous to some extent with intraocular pressure, blood pressure also follows a circadian rhythm and can be described and categorized by four distinct patterns (Table 24.2). The normal pattern is defined by a nocturnal dip in mean systolic and diastolic blood pressure by greater than 10 % but less than 20 %. Multiple studies have shown that nondippers and reverse dippers (i.e., those with sustained blood pressure elevations) are at significantly increased risk of ischemic and hemorrhagic stroke [20–29]. In Japan, the Ohasama epidemiologic study reported the prognostic significance of blood pressure variability in a population cohort followed for 20 years [27]. Using ambulatory and home blood pressure-monitoring data, the investigators found that flattening of the blood pressure profile (i.e., loss of the normal nocturnal dip) as well as wide blood pressure variability characterized by morning blood pressure surges and nocturnal hypotension are both associated with cerebral hemorrhagic strokes. Patients with the lowest risk for stroke are those with a normal nocturnal dip. Hence stroke risk, with respect to the circadian blood pressure pattern, follows a J-shaped curve [23, 27]. These and other similar studies have contributed to the emerging paradigm in the pharmacologic treatment of arterial hypertension in which the goal is not only achievement of lower target pressures according to level of cardiovascular risk but also the attainment of the normal circadian blood pressure profile whereby the normal nocturnal dip is restored. Indeed, some physicians have utilized data obtained from results of ambulatory blood pressure monitoring to plan tailored dosing and administration of antihypertensive agents [30, 31]. This newer concept of targeted blood pressure control derived from modeling of the patient’s circadian blood pressure profile and then using specific antihypertensive agents based on differences in kinetics and the benefits and side effects of each agent to achieve 24-h blood pressure control is called chronotherapy (see Sidebar 24.1). At the moment, this mode of treatment is predominantly conducted in a research setting and not yet the standard of care. Further clinical studies will help determine the feasibility of adopting this method in general practice and its impact on stroke and heart attack prevention. There is an ongoing clinical trial, currently in its fourth year, designed to investigate chronotherapy and its potential impact on cardiovascular, cerebrovascular, and renal risk [32]. Data from this trial are not yet available.
Table 24.2
Nocturnal blood pressure variability patterns
Category | Mean decrease in systolic and diastolic BPa |
---|---|
Normal | >10 % but <20 % |
Nondipper | <10 % |
Reverse dipper | Nocturnal BP > diurnal BP |
Overdipper | >20 % |
Sidebar 24.1. Antihypertensive Medications and Glaucoma
Kevin C. Leonard and2 and Cindy M. L. Hutnik2
(2)
K.C. Leonard, MSC, MD, Cindy M.L. Hutnik, MD, PhD Departments of Ophthalmology and Pathology, University of Western Ontario, Ivey Eye Institute, St. Joseph’s Health Care, London, ON, Canada
There are a variety of well-established risk factors for primary open-angle glaucoma (POAG) including age, race, positive family history, myopia, and, most importantly, ocular hypertension. However, a significant subgroup of patients with glaucoma lacks any of these known risk factors. Other potential risk factors have been proposed and investigated. Most of these are vascular risk factors and include both systemic hypertension and hypotension, low diastolic perfusion pressure, as well as altered ocular blood flow [1]. A risk factor that has garnered notable attention is the effect that treatment of systemic hypertension may have on the incidence or progression of open-angle glaucoma.
The potential impact of a demonstrated effect of antihypertensive treatment in glaucoma would be great, given the number of patients with glaucoma who are also being treated for systemic hypertension.
Rationale
Several concepts relevant to understanding the potential effect of systemic antihypertensives on glaucoma are important. Diastolic ocular perfusion pressure (DOPP) is the diastolic blood pressure (DBP) minus the intraocular pressure (IOP). It can be regarded as the “driving force” to move blood into the eye. The Baltimore eye survey [2] revealed an increased prevalence of POAG in the presence of systemic hypotension and low DOPP. Several major longitudinal studies have confirmed DOPP as a risk factor [2–4]. Decreasing IOP will improve DOPP while decreasing DBP will worsen DOPP. Medications that manipulate either BP or IOP can have an effect on the perfusion pressure. This provides a rationale for the possibility that systemic antihypertensive treatments may be linked to the progression of glaucoma due to the effect on DOPP.
There is considerable evidence that nocturnal hypotension may be important in the pathogenesis of glaucoma. Many studies have shown that it is associated with increased incidence of glaucoma, increased severity of disease at diagnosis, and increased progression of the disease [1, 5]. Increased progression of visual field loss has been reported in patients with nocturnal hypotension linked to the use of systemic antihypertensives [6]. Angiotensin-converting enzyme (ACE) inhibitors and calcium channel blockers (CBB) exaggerate the normal nocturnal hypotensive episodes, while the same has not been found for β-blockers and angiotensin receptor blockers. The effect of diuretics on the exaggerated nocturnal hypotensive dip is variable.
The concept of peripheral vascular dysregulation has also been associated with the pathogenesis of glaucoma. This concept can be defined as an imbalance between the blood supply to a tissue and its metabolic demands, resulting in either overperfusion or underperfusion. An imbalance between vasodilator and vasoconstrictor substances may result in vascular dysregulation. This phenomenon has been identified in subgroups of glaucoma patients, resulting in unstable ocular blood flow. The end result is a perfusion/reperfusion injury and oxidative stress [7, 8].
Evidence
Several studies have examined systemic antihypertensive use as a risk factor for the development or progression of glaucoma. The Thessaloniki Eye Study, which was a population-based cross-sectional study, measured the effect of BP status on optic disk structure in 232 patients without glaucoma using Heidelberg retina tomograph (HRT) [9]. This study revealed that diastolic BP <90 mmHg, specifically resulting from antihypertensive treatment in nonglaucomatous patients, was associated with increased cupping and decreased rim area of the optic disk [10]. Similarly, a positive association between low DOPP (<50 mmHg) and high-tension glaucoma (OR 4.68) was identified only for patients receiving systemic antihypertensive treatment in the Rotterdam Study [11]. Interestingly, an inverse association was noted for normal-tension glaucoma (OR 0.25). Another study used confocal scanning laser ophthalmoscopy to evaluate the effect of systemic antihypertensive treatment on progression of optic nerve parameters in glaucoma suspects. In comparison to age-matched normotensive controls, statistically significant increases were identified in cup area and cup-to-disk area ratio, while rim area, rim-to-disk area ratio, and global retinal nerve fiber layer (RNFL) thickness were all decreased [12].
Population data have provided some conflicting results. Data from the Barbados Eye Study Group suggested that hypertensive patients on treatment had a decreased risk of developing glaucoma over a 4-year span as compared to normotensive controls (RR 0.49). Hypertensive patients using antihypertensive medications were shown to have a decreased risk in comparison to nonusers (RR 0.74), although this was not a statistically significant effect [13]. Several authors have suggested that these contradictory results are likely attributable to different study designs and study populations.
Langman et al. [9]. have presented data suggesting that systemic hypertension was significantly more common in patients with glaucoma based on a case–control study with a very large sample size. Oral b-blockers were shown to have a statistically significant protective effect (OR 0.77), while oral calcium channel blockers and angiotensin-converting enzyme (ACE) inhibitors were associated with a significant increase in the risk of developing glaucoma (OR 1.34 and 1.16). Diuretics were not identified to have any significant effect either way in this study. The Rotterdam Study was a prospective population-based cohort study that revealed the effect of antihypertensive medications on the incidence of open-angle glaucoma over a 6.5-year period. Patients using calcium channel antagonists were found to have a statistically significant 1.8-fold higher risk. β-Blocker treatment was associated with a nonsignificant risk reduction (OR 0.60), while other types of antihypertensive agents were not significantly associated with the incidence of open-angle glaucoma [14]. This apparent risk associated with the use of calcium channel blockers contradicts the results of several other studies, including the initial reports of the Rotterdam Study. The initial Rotterdam Study demonstrated a lower IOP and protective effect on OAG in patients using systemic b-blockers, while ACE inhibitors and calcium channel blockers showed no effect on IOP [15]. Results from the European Glaucoma Prevention Study (EGPS) provided some additional insight into the effects of the various antihypertensive agents. The EGPS was a randomized, double-masked controlled clinical trial designed to assess treatment with dorzolamide versus placebo. Intercurrent factors that were associated with the development of open-angle glaucoma were examined. This study was the first to suggest systemic diuretics as a risk factor (HR 2.41) for the development of glaucoma [4].
Clinical Recommendations
Based on the studies done to date, it appears that systemic antihypertensive therapy may have an effect on the progression of optic nerve damage in glaucoma. A possible mechanism may be the reduction in DBP associated with antihypertensive therapy with exaggerations in the nocturnal dipping of DOPP. Vascular dysregulation may be an essential element linking systemic antihypertensive use and hypotension to the pathophysiology of glaucoma.
The available evidence suggests that β-blockers are the most well-tolerated systemic antihypertensive agents from a glaucoma standpoint and may even have a protective effect. Other agents, including diuretics, ACE inhibitors, and calcium channel antagonists, have been associated with an increased risk of glaucoma.
Collectively, this evidence brings the importance of systemic blood pressure into the realm of the ophthalmologist. Patients demonstrating glaucomatous progression at low intraocular pressures may benefit from 24-h ambulatory blood pressure monitoring. Collaboration may be required between the ophthalmologist and internist to select the ideal antihypertensive medication and dosing regimen. Long-lasting medications may be preferred. If short-acting medications are required, evening doses may need to be eliminated. However, it should be cautioned that although the evidence is growing, it may still be insufficient to make solid recommendations regarding appropriate blood pressure management for patients with glaucoma. At present, the recommendation may be to obtain a relatively stable blood pressure over 24 h with increased attention to hypotensive episodes, especially at night. The ophthalmologist must work closely with other care providers to balance the potential progression of glaucoma with other risk factors for systemic disease requiring control of hypertension.
Bibliography
Deokule S, Weinreb RN. Relationships among systemic blood pressure, intraocular pressure, and open-angle glaucoma. Can J Ophthalmol. 2008;43(3):302–7.
Tielsch JM, Katz J, Sommer A, Quigley HA, Javitt JC. Hypertension, perfusion pressure, and primary open-angle glaucoma. A population-based assessment. Arch Ophthalmol. 1995;113(2):216–21.
Leske MC, Heijl A, Hyman L, Bengtsson B, Dong L, Yang Z. Predictors of long-term progression in the early manifest glaucoma trial. Ophthalmology. 2007;114(11):1965–72.
Miglior S, Torri V, Zeyen T, Pfeiffer N, Vaz JC, Adamsons I. Intercurrent factors associated with the development of open-angle glaucoma in the European glaucoma prevention study. Am J Ophthalmol. 2007;144(2):266–75.
Pache M, Flammer J. A sick eye in a sick body? Systemic findings in patients with primary open-angle glaucoma. Surv Ophthalmol. 2006;51(3):179–212.
Hayreh SS, Zimmerman MB, Podhajsky P, Alward WL. Nocturnal arterial hypotension and its role in optic nerve head and ocular ischemic disorders. Am J Ophthalmol. 1994;117(5):603–24.
Nicolela MT. Clinical clues of vascular dysregulation and its association with glaucoma. Can J Ophthalmol. 2008;43(3):337–41.
Flammer J, Mozaffarieh M. Autoregulation, a balancing act between supply and demand. Can J Ophthalmol. 2008;43(3):317–21.
Langman MJ, Lancashire RJ, Cheng KK, Stewart PM. Systemic hypertension and glaucoma: mechanisms in common and cooccurrence. Br J Ophthalmol. 2005;89(8):960–3.
Topouzis F, Coleman AL, Harris A, et al. Association of blood pressure status with the optic disk structure in non-glaucoma subjects: the Thessaloniki eye study. Am J Ophthalmol. 2006;142(1):60–7.
Hulsman CA, Vingerling JR, Hofman A, Witteman JC, de Jong PT. Blood pressure, arterial stiffness, and open-angle glaucoma: the Rotterdam study. Arch Ophthalmol. 2007;125(6):805–12.
Punjabi OS, Stamper RL, Bostrom AG, Lin SC. Does treated systemic hypertension affect progression of optic nerve damage in glaucoma suspects? Curr Eye Res. 2007;32(2):153–60.
Leske MC, Wu SY, Nemesure B, Hennis A. Incident open-angle glaucoma and blood pressure. Arch Ophthalmol. 2002;120(7):954–9.
Muskens RP, de Voogd S, Wolfs RC, et al. Systemic antihypertensive medication and incident open-angle glaucoma. Ophthalmology. 2007;114(12):2221–6.
Dielemans I, Vingerling JR, Algra D, Hofman A, Grobbee DE, de Jong PT. Primary open-angle glaucoma, intraocular pressure, and systemic blood pressure in the general elderly population. The Rotterdam Study. Ophthalmology. 1995;102(1):54–60.
Vascular Factors and Glaucoma
Hypertension, Blood Flow, and Glaucoma Risk
The current evidence in the medical literature has firmly established that hypertension is an important risk factor in the development of stroke, heart disease, and renal failure, with increasing risk of developing target organ injury or death and disability with higher blood pressures. In contrast, cross-sectional data from population-based studies linking hypertension and glaucoma have been mixed [3–9]. To complicate matters, recently published data from the Early Manifest Glaucoma Treatment Study (EMGTS) and the Barbados Eye Study have shown an inverse relationship with respect to blood pressure and risk of glaucoma progression, results that are paradoxical as compared to data from the medical literature [33, 34]. Analyzing the data from the EMGTS, Leske et al. identified lower ocular systolic perfusion pressure (defined as systolic blood pressure minus IOP ≤125 mmHg) in all patients (HR 1.42) and lower systolic blood pressure (SBP ≤160 mmHg) in patients with lower baseline IOP as new baseline predictors of glaucoma progression in their study cohort [33]. Conversely, the risk of progression was halved (HR 0.46) in patients with SBP >160 mmHg. In stark contrast, the Systolic Hypertension in the Elderly Program (SHEP), the Systolic Hypertension in Europe (Syst-Eur) Trial, and the Systolic Hypertension in China (Syst-China) study have all shown that those with isolated SBP of greater than 160 mmHg have a significantly increased cardiovascular event rate and benefit from antihypertensive therapy [35–37]. Pooled estimates from the three trials demonstrated that active treatment, compared with placebo, reduced all-cause mortality by 17 %, cardiovascular mortality by 25 %, all cardiovascular end points by 32 %, total stroke by 37 %, and myocardial infarction including sudden death by 25 % [38]. Furthermore, patients with optimal blood pressure (<120/80 mmHg) have a two- to threefold lower cardiovascular event risk rate as compared to those with high-normal blood pressure [13, 14]. In other words, in terms of blood pressure as a vascular risk factor, it appears that high blood pressure may be “neuroprotective” from the glaucoma perspective but detrimental to the heart, brain, and kidney and vice versa thus creating an untenable therapeutic dilemma. In fact, Leske et al. suggested maintaining elevated blood pressures in hypertensive patients (Barbados Eye Study) [34], while clinicians who treat arterial hypertension and follow the prevailing guidelines will ideally aim for lower target blood pressures based on cardiovascular risk with restoration of a normal 10–20 % nocturnal dip in mean blood pressure. This contradictory or paradoxical relationship between glaucoma and hypertension needs further clarification. There are two distinctly separate but interrelated pathophysiologic mechanisms at hand that when treated separately may help resolve this apparent paradox: the relationship between hypertension and glaucoma and low perfusion and glaucoma. At the moment, it appears from the available evidence, mostly from prevalence-based epidemiological studies, that high blood pressure, at the very least, does not appear to increase the risk of future development of glaucoma. The logic in this observation is that high blood pressure is necessary to maintain perfusion to the optic nerve particularly in those with elevated IOP, which counteracts the driving force for perfusion. One must be careful, however, not to reflexively assume that a higher systolic pressure translates to greater perfusion to the optic nerve or any other tissue, especially in patients with known cardiovascular disease. Blood flow depends not only upon perfusion pressure but also vascular resistance. Patients with hypertension, particularly those who have higher blood pressures, often have correspondingly elevated catecholamine levels contributing to increased peripheral vascular resistance. Furthermore, intimal damage from chronic hypertension further compromises vascular integrity as well as resistance, thereby increasing the risk of hemorrhage or ischemic injury. What then might cause failure of finding an association between hypertension and glaucoma? In some of the glaucoma epidemiologic studies, a diagnosis of hypertension was considered to be present based on verbal history or on isolated blood pressure measurements in the clinical trial [3–9]. The white coat syndrome is a well-described cause of misclassification of normotensive individuals as hypertensives. Pickering reported that as many as 20 % of normotensives may be misclassified as hypertensives [39]. Such misclassification can certainly confound the data and underestimate the relationship. Also, since the incidence of glaucoma is low, it is possible that if only a subset of patients with more severe or labile hypertension is at higher risk of developing glaucoma, the association will be missed. Long-term incidence studies may identify an association that prevalence studies have failed to do. In contrast to high blood pressure, low blood pressure or low ocular perfusion pressure has been identified as a potential vascular risk factor in glaucoma [5, 6, 8, 9, 33, 34, 40–44]. In the Barbados Eye Study, low systolic, diastolic, and mean ocular perfusion pressures have been associated with an increased relative risk of developing glaucoma [34]. These data complement other evidence in the ophthalmic literature to show that nocturnal hypotension is associated with progressive injury in both normal tension and open-angle glaucoma. Moreover, low perfusion is consistent with increased brain ischemia and stroke as well as myocardial injury in hypertensive patients with an overdipping circadian rhythm. As shown in the Ohasama study, wide variability in blood pressure with nocturnal overdipping is also associated with an increased risk of cerebral hemorrhage [27]. Thus, it is likewise reasonable to presume that independent of IOP, similar episodes of extreme nocturnal blood pressure dipping due to unstable hypertension or autonomic dysfunction can lead to ocular hypoperfusion and visual field progression in glaucoma patients. Graham, Follman, Collignon, Kaiser, and Meyer all reported increased nocturnal dips or overdipping in both primary open-angle glaucoma (POAG) and normal-tension glaucoma (NTG) patients with disease progression [40–42, 45, 46]. Bechetoille found lower blood pressures, but the number of nocturnal low readings was no different in patients with focal ischemic NTG than in POAG patients [47]. Complementary to data from the medical literature, Collignon, Kashiwagi, and Detry found that disease progression may also be associated with absent or insufficient nocturnal dip [45, 48, 49].
Thus, labile hypertension with episodes of hypotension or overaggressive treatment of hypertension may create a low flow state especially in those with elevated IOP, thereby contributing to glaucomatous optic nerve damage. Also, dysregulation of the normal circadian blood pressure rhythm with absent or reverse nocturnal dip may also contribute to optic nerve injury. Further prospective studies investigating circadian blood pressure variability and risk to the optic nerve in glaucoma patients are warranted. At this time, it appears from both the ophthalmic and medical literature that episodes of exaggerated nonphysiologic nocturnal hypotension are likely detrimental and should be avoided as they appear to increase the risk of progressive optic nerve damage as well as stroke and cardiac ischemia. Thus, until further data are available, low perfusion states but not physiologic nocturnal dipping are bad, and the connection between hypertensive patients and glaucoma progression risk, at the moment, may be related to an overdipper circadian pattern in some patients caused by intrinsic autonomic instability or overaggressive antihypertensive treatment. Aggressive control of intraocular pressure, as well as coordination of targeted blood pressure therapy with the patient’s primary care physician or internist, to avoid nonphysiologic nocturnal hypotension would be appropriate management in these patients. Overly simplistic measures such as advising a high-salt diet or high-salt beverages and foods before bedtime in the absence of blood pressure data from ambulatory blood pressure monitoring, knowledge of the patient’s antihypertensive regimen, and appropriate supervision and input from the patient’s internist may in fact be detrimental.
Hypothyroidism and Glaucoma
Hypothyroidism is a common condition that increases in prevalence with age but is overwhelmingly more common in females than males. The prevalence of hypothyroidism depends upon the geographic area as it relates to iodine availability. In areas of adequate iodine supply, the prevalence is 0.8–1.0 %. The diagnosis is often elusive due to the nonspecific nature of the symptoms such as fatigue, weight gain, constipation, depressed mood, and cold intolerance. Biochemically, cellular metabolism is considerably slowed and there is increased tissue deposition of mucopolysaccharides. In advanced cases, myxedema develops, which imparts swelling and a change in skin texture to a doughy consistency that is nonpitting [50]. The relationship between hypothyroidism and glaucoma was first suggested by Hertel nearly 90 years ago [51]. Mucopolysaccharide deposition in the trabecular meshwork is thought to belie the mechanism of outflow obstruction. Indeed there have been multiple studies that have shown reduced tonographic outflow facility or elevated IOP in patients with hypothyroidism [52–55]. Smith et al. demonstrated in a series of 25 consecutive patients with hypothyroidism that treatment with thyroid hormone improved tonographic outflow facility, reduced IOP, and hence reduced the pressure/outflow facility ratio [54]. They also describe an anecdotal case report of a patient with hypothyroidism and glaucoma with refractory IOP elevation that improved only following treatment of hypothyroidism and which also resulted in reduction of the medical regimen to treat IOP [56]. As opposed to trabecular meshwork dysfunction, vasculopathy causing altered ocular blood flow and weakening of the lamina cribrosa are also theoretical mechanisms by which hypothyroidism can contribute to the development of glaucomatous optic neuropathy in patients in the absence of elevated IOP.
Although mucopolysaccharide deposition causing trabecular dysfunction and elevated IOP is certainly biologically plausible, review of the literature reveals mixed data regarding the clinical relationship of glaucoma and hypothyroidism [55–65]. Most of the published data essentially arises from case–control epidemiologic studies, consecutive case series, or a case report, which often demonstrate flaws in design and introduce bias. Unfortunately, as expected, the low annual incidence of glaucoma in the general population precludes the availability of prospective incidence data, and thus, scientific assessment of the relationship can only be practically made through case–control studies. Most of these studies, whether case–control or consecutive case series, however, identified fewer than 100 cases of hypothyroidism or glaucoma [55, 57–62]. There are only three published trials with greater than 100 patients and only two available in print [63–65]. The Blue Mountain Eye Study showed that of 147 patients on thyroxine replacement therapy, 6.8 % had glaucoma as compared to 2.8 % of age-matched controls [63]. The largest of the case–control epidemiologic studies in print was a nested case–control study conducted in men at a US Veterans Administration (VA) medical center by Girkin et al. [64]. In this study 590 newly diagnosed patients with glaucoma matched with 5,897 nonglaucoma controls were found to be more likely to have a prior diagnosis of hypothyroidism as compared to the control group: 6.4 % versus 4.0 %. The diagnosis of glaucoma was made between 1997 and 2001 and was based on identifying glaucoma cases from recorded ICD-9 diagnoses. Similarly hypothyroidism was based on ICD-9 diagnosis and or concurrent thyroxine use as determined by prescriptions filled. Although the study findings were intriguing and support the potential relationship between hypothyroidism and glaucoma, there are significant weaknesses in the study design. The study was done only in men and cannot be extrapolated to women who are significantly more likely to be diagnosed with hypothyroidism. The diagnosis was made by identifying an ICD-9 diagnosis recorded between a specified time interval, and perhaps with a longer follow-up interval, the incidence of glaucoma between the hypothyroid group and nonhypothyroid group may even out. There are many patients with significantly high TSH (biochemical hypothyroidism) but are clinically asymptomatic and not under treatment with thyroxine replacement, thus potentially underestimating the pool of patients that are hypothyroid and who may not have been coded as hypothyroid. Moreover, only a diagnosis of glaucoma made after the start of the observation period was considered. Since glaucoma does not start abruptly, it is possible that a “delayed” diagnosis of glaucoma resulted in a left bias as the authors conceded, thus questioning the veracity of the timing of the development of hypothyroidism preceding the diagnosis of glaucoma. The most recent and by far the largest epidemiologic study (EPUB publication), in contrast, failed to show an association between a prior diagnosis of hypothyroidism and development of glaucoma [65]. In that study Motsko and Jones, using a similar case–control study design as Girkin et al. identified 4,728 newly diagnosed patients and matched them with 14,184 controls. Prior hypothyroidism in open-angle glaucoma patients was found in 17.2 % versus 17.6 % in controls, which is significantly higher in both cases and controls as compared to the VA study by Girkin. This finding is as might be expected due to the inclusion of women in the study by Motsko and Jones. Although both studies shared similar weaknesses, the strengths of the Motsko and Jones study as compared to the Girkin et al. study were the larger number of cases of glaucoma and the demographically more diverse study group in the former, hence a better representation of the population.
At this juncture, a causal or permissive relationship between hypothyroidism and glaucoma is intriguing, but the available data have failed to prove this relationship. Even though elevated IOP has been demonstrated in patients with hypothyroidism, Bahçeci UA et al. have recently shown that there is a reversible decrease in central corneal thickness that may completely account for the observed improvement in IOP before and after hormone replacement therapy [54, 55]. Additional investigation, preferably prospective in nature, is therefore warranted to determine whether hypothyroidism potentially increases the risk of development of open-angle glaucoma.
Diabetes and Glaucoma
Diabetes affects 6–8 % of the population and 95 % of all diabetics have type 2 diabetes [66]. Beginning with the Framingham Heart Study, the relationship between diabetes and the development of cardiovascular, cerebrovascular, and peripheral vascular disease has been firmly corroborated by numerous studies that followed [67, 68]. Most diabetics will die a cardiac death, and the risk of an acute coronary event in a diabetic is high enough that the National Cholesterol Education Program – Adult Treatment Panel III (NCEP-ATPIII) guidelines on the treatment of hyperlipidemia recommend aggressive lowering of LDL cholesterol to the same target levels as in patients with manifest coronary artery disease [15, 69, 70]. The American Diabetes Association and the American Heart Association likewise categorize diabetes as a coronary artery disease risk equivalent [69, 70]. Furthermore, the metabolic syndrome, which includes insulin resistance as a feature, confers a significantly increased risk of developing a coronary event [69, 70]. Target organ damage due to diabetes is related to development of micro- and macrovascular disease with accelerated atherosclerosis.
While diabetes is an established risk factor for vascular disease and end-stage renal failure and low blood flow has been implicated as a potential risk factor in the pathophysiology of glaucoma, the relationship between diabetes and glaucoma has been subject to much debate and controversy. In the streptozotocin-induced diabetic mouse and rat model, there are experimental data that show retinal ganglion cell loss as well as abnormal morphology and increased numbers of dendritic terminals in the surviving retinal ganglion cells after 3 months [71, 72]. In addition, retrograde axoplasmic flow has also been shown to be reduced in large- and medium-type retinal ganglion cells [73]. Clinically, Soares et al. in a prospective cohort study of 137 patients with primary open-angle glaucoma, found that diabetes is significantly associated with development of optic disk hemorrhages, with a hazard ratio of 4.4 as compared to nondiabetics [74]. Elisaf et al. studied metabolic abnormalities among patients with known primary open-angle glaucoma and showed that elevated glucose as well as uric acid levels were significantly higher as compared to a matched control group [75]. Similarly, Oh et al. found that insulin resistance in patients with the metabolic syndrome was associated with elevated IOP and that mean IOP increased linearly with the presence of increasing numbers of components for the metabolic syndrome [76]. These and other experimental and clinical studies have provided evidence for diabetes as a causative or permissive factor in the development of glaucoma. Unfortunately, much of the controversy regarding the relationship between diabetes and glaucoma stems from the inconsistent results obtained from larger clinical and population-based epidemiologic studies [1, 77–89]. The OHTS initially showed that having diabetes, in fact, was surprisingly protective of the development of glaucoma [1]. In this study, however, the diagnosis of diabetes was identified by self-reporting without validation by record review, blood tests, or treatment history. The protocol also specifically excluded those with diabetic retinopathy since diabetic retinopathy could influence results of visual field testing and confound the specificity of visual field changes. Unfortunately, such exclusion creates an obvious selection bias in favor of diabetics with less severe disease and hence a prognostically different subgroup. Gordon et al. recognizing the methodological shortcomings of the study, reran the data using more detailed information regarding the diagnosis of diabetes as well as the details of their treatment [77]. During this follow-up study, 409 patients responded positively to the same question originally posed as compared to 191 in the original publication but were now divided into three sensitivity/specificity subgroups based on what type of treatment was recommended. Using the same Cox proportional hazard prediction model, this follow-up study determined that a history of diabetes mellitus was not statistically significantly predictive for the development of POAG and failed to support the original conclusion that diabetes was protective of glaucoma in patients with ocular hypertension. A smaller cohort of ocular hypertensives from the Diagnostic Innovations in Glaucoma Study yielded similar hazard ratios as in the OHTS analysis for all reported risk factors for progression, except that diabetics who progressed to glaucoma had an increased hazard ratio as compared to those that did not progress [90]. In the European Glaucoma Prevention Study (EGPS), only 4.7 % of 1,077 randomized participants with ocular hypertension reported diabetes, a number too small to determine prospectively the effect of diabetes on progression to glaucoma [91, 92].
The majority of the published data regarding the relationship between diabetes and glaucoma are derived from population-based cross-sectional studies, case–control studies, and cohort studies with fewer prospective population-based incidence studies such as the Barbados Eye Study [78, 80–89]. In aggregate, there was considerable heterogeneity with respect to race and ethnicities, though the individual studies came from predominantly homogeneous population groups. Vijaya et al. did not find diabetes to be associated with glaucoma in a South Indian population in Chennai [80]. Neither de Voogd in a prospective cohort study in Rotterdam nor Ellis in the DARTS/MEMO (Diabetes Audit and Research in Tayside Study/Medicines Monitoring Unit) in Scotland found an association between diabetes and glaucoma [81, 82]. The latter study population was comprised of 6,631 diabetics and 166,144 nondiabetic participants [82]. The Baltimore Eye Survey, a predominantly African-American population, failed to show that diabetes was associated in the development of glaucoma [78, 79]. In an 11 county area of southern Wisconsin, a predominantly Caucasian population with diabetes was compared to a smaller group of nondiabetics and was found to have a tendency toward a greater mean intraocular pressure than nondiabetics and higher rates of a positive history of glaucoma than in diabetic participants [83]. In a 10-year follow-up prospective study of the same cohort of diabetic participants, multivariate analysis of risk factors associated with increased self-reporting of glaucoma identified age in younger patients, age, baseline intraocular pressure, and insulin use in older diabetics as statistically significant risk factors [84]. The Los Angeles Latino Eye Study (LALES), a population-based cross-sectional study, recently showed that in Latinos over 40 years of age who were never on any glaucoma hypotensive therapy, diabetes was one of several systemic factors independently and positively correlated with elevated IOP on clinical examination. Furthermore, those with type 2 diabetes mellitus, defined as having diabetes after the age of 30, the prevalence of glaucoma was 40 % higher than those without type 2 diabetes [85, 86]. The patient was determined to be diabetic in the LALES if the participant had a history of being treated for diabetes, the glycosylated hemoglobin was measured at 7.0 % or higher, or a random blood glucose was 200 mg or higher. The Barbados Eye Study, a prospective population-based incidence study, described the temporal changes in intraocular pressure over a 9-year time period in a population of participants of African descent and identified a history of diabetes in a multivariate analysis as one of several systemic risk factors correlated with increasing intraocular pressure [87]. These data were consistent with a previously published report in the same study population that also identified diabetes as a significant risk factor in longitudinal increases in intraocular pressure after a 4-year follow-up [88]. In another recent prospective analysis of a cohort of women over 40 years of age from the Nurses’ Health Study observed between 1980 and 2000, Pasquale et al. found that type 2 diabetes mellitus was positively associated with development of primary open-angle glaucoma as confirmed by record review with a relative rate ratio of 1.82 [89]. In this study, a diagnosis of diabetes was validated on a supplemental questionnaire. Confounders such as age, race, hypertension, body mass index, family history, alcohol intake, smoking, and physical activity were controlled for including the potential for detection bias based on the number of eye exams (see Sidebar 24.2). The latter is a factor commonly held by many as the reason for finding a positive association between diabetes and glaucoma or ocular hypertension.
The data are conflicting, and the only meta-analysis published to date regarding the association between diabetes and glaucoma, prior to the availability of the results of the LALES and the Nurses’ Health Study, failed to identify diabetes as a potential risk factor [93]. Furthermore, interestingly, one study showed that changes in the biomechanical properties of the cornea due to increased glycosylated hemoglobin may artificially influence intraocular pressure measurements leading to a false-positive association between diabetes and elevated intraocular pressure [94]. Krueger and Ramos-Esteban proposed that corneal stiffening due to glucose-mediated collagen cross-linking may account for higher intraocular pressure readings in diabetics. Notwithstanding, more recent prospective well-designed studies such as the Barbados Eye Study and the Nurses’ Health Study are showing a positive association. The LALES, which also showed a positive association in a cross-sectional study of a predominantly Mexican Hispanic population and thus subject to inherent biases of similar prevalence-based studies, appeared to reduce patient recall bias as well as misclassification by validating a diabetes diagnosis through both medical history and blood testing. Selection bias, however, remains a potential confounder in a restricted population study set. Other reasons for the conflicting data regarding diabetes and glaucoma are the low prevalence of both diabetes and glaucoma as compared to cardiovascular diseases and the often equivocal nature of a glaucoma diagnosis leading to potential misclassification and underreporting, thus biasing against an association. This is unlike coronary artery or cerebrovascular disease where an ischemic event when it occurs is clearly evident. At the moment, the available evidence regarding the effect of diabetes on the development of glaucoma is equivocal. More prospective studies will be necessary to determine whether a causal or permissive relationship exists between diabetes and glaucoma.
Sidebar 24.2. Glaucoma, Diet, Exercise, and Lifestyle
Janet Betchkal3 and Rick E. Bendel4
(3)
J. Betchkal, MD Department of Ophthalmology, St. Vincent’s Medical Center, Jacksonville, FL, USA
(4)
R.E. Bendel, MD Department of Ophthalmology, Mayo School of Medicine, Mayo Clinic Jacksonville, Jacksonville, FL, USA
Anyone treating glaucoma patients is used to facing questions about how a patient’s lifestyle, habits, and/or emotional state may affect his or her intraocular pressure (IOP) or, more accurately, their risk of glaucomatous progression.
As with most chronic diseases, glaucoma risk factors can be divided into “nonmodifiable” and “modifiable” categories. The former would include family history, age, gender, underlying pathology, and genetics. The modifiable category includes the lifestyle issues over which we might be able to exert some level of “control.”
Through the years, there have been numerous studies that have demonstrated the relationship between IOP and the development of and/or progression of open-angle glaucoma [1–3]. Therefore, most research and review articles have focused on the affects of various lifestyle factors on IOP. Some of the factors examined include diet, exercise, systemic hypertension, cigarette smoking, alcohol consumption, caffeine ingestion, marijuana usage, and various types of stress [4].
There are a plethora of non-Food and Drug Administration (FDA)-approved “supplements” on the market that tout their effectiveness for various disease states of the body [5, 6], including the eye. However, these herbal or nutritional supplements are not regulated by the FDA as are prescription drugs, and as such, the makers of these products can make statements that are not based on evidence-based scientific data [7].
There are several well-designed studies indicating that the use of zinc and oral antioxidant food supplements may help prevent progression in patients with age-related macular degeneration. However, there is no similar evidence to indicate that nutritional supplemental therapy will help prevent the progression of damage to the optic nerve, visual field, or vision in patients with primary open-angle glaucoma [8, 9]. Nonetheless, there are several supplements that are specifically marketed to the glaucoma patient to help stabilize or improve their disease [10–12]. These include bilberry, lutein, and ginkgo [13]. In our opinion, there is no clear evidence that these help in the treatment of glaucoma; at this time, there is also no data to suggest that they are harmful to the glaucomatous eye. We recognize that there are different opinions about the validity of the literature.
Some studies have shown that obesity is a risk factor for elevated IOP [14, 15], while other studies have determined that caloric restriction may be neuroprotective in rats and other animal models [16–18].
While there is no denying the overall health benefits of a well-balanced diet and a regular exercise routine [19], the advantages, if any, to glaucoma patients are less clear. Aerobic exercise has been shown to reduce IOP more in glaucoma patients than in healthy individuals [20–22]. This effect is greatest in sedentary individuals starting an exercise program for the first time. The acute IOP reduction may be as great as 7–13 mmHg in primary open-angle glaucoma patients. However, the effect is short-lived and IOP has been shown to return to baseline in as little as 40 min [20, 21].
Interestingly, physically fit glaucoma patients and individuals participating in as little as 3 months of conditioning have been shown to have lower resting IOP levels; [23, 24] however, these same individuals show much less of an acute decrease in IOP after bouts of aerobic exercise than do their sedentary counterparts [25, 26]. The mechanism responsible for the decrease in IOP in these two subsets of glaucoma patients is not fully understood [27–29].
In contrast, any activities involving the Valsalva maneuver and/or anaerobic or isotonic exercise are associated with an acute increase in IOP [30]. One activity that should clearly be avoided in glaucoma patients would be any regimen that involves total-body inversion or head stands, as the immediate increase in IOP can be as high as 16 mmHg [31, 32].
There are many good reasons to stop smoking cigarettes that have nothing to do with glaucoma. And, while cigarette smoking has been shown to cause a mild and transient increase in IOP in some primary open-angle glaucoma patients [33, 34], several large studies have failed to demonstrate a link between cigarette smoking and increased incidence or prevalence of ocular hypertension or glaucoma [35, 36].
In 1965, Pecson and Grant [37] first reported that alcohol consumption caused a much greater decrease in the IOP of primary open-angle glaucoma patients than in the normal population. The effect is transient and tends to dissipate in 2–5 h [37, 38]. The clinician would be advised to exercise caution in relating this information to the patient, as he or she would not want to encourage binge drinking or, worse, create a situation whereby the patient is in a constant state of inebriation. In known alcoholics and/or in any patient who has alcohol on the breath, it is probably worth noting the time since ingestion of alcohol in relationship to the time of IOP measurement. A word of caution here: we have noted several incidences where the physician and staff have noted the typical aroma of alcohol (presumably on the patient’s breath), only to later realize that the odor is secondary to the use of hand sanitizers (an increasingly popular product on the market).
A majority of the population consumes caffeine in some form on a regular basis. In fact, it is estimated that in the United States, 80 % of people over the age of 50 consume caffeine on a daily basis [38]. More than two billion pounds of caffeine-containing beverages are consumed annually in the United States alone [39]. It is contained in numerous beverages such as coffee, tea, and soft drinks and in foods and various medications [40]. Caffeine’s effects on the human body are well known and include increased blood pressure, decreased heart rate, increased wakefulness, and reduced cerebral blood flow [41, 42].
Overall, most studies have shown that caffeine causes a transient increase in the IOP of normal, ocular hypertensive, and open-angle glaucoma subjects [43–48]. However, there are a few studies that did not reach this same conclusion [49–51]. Despite the fact that most studies demonstrated a statistically relevant elevation in IOP in response to caffeine ingestion, some investigators like Higginbotham et al. [44]. did not feel that this was “clinically relevant” and went so far as to recommend that glaucoma patients not be dissuaded from consuming caffeinated beverages, while others recommended that patients with glaucoma or ocular hypertension limit their daily caffeine consumption to a specified amount [47]. It may be that patients vary in response to caffeine and that it has more of an effect on select individuals than others based on a host of unstudied factors.
Just about everyone knows (or think they know) that marijuana (cannabis) is useful in the treatment of glaucoma. Ever since Hepler and Frank first reported the effects of marijuana smoking on IOP in 1971 [52], this has a been a hot topic that resurfaces every few years – usually when some celebrity is diagnosed with glaucoma or a law favoring legalization of marijuana is up for consideration.
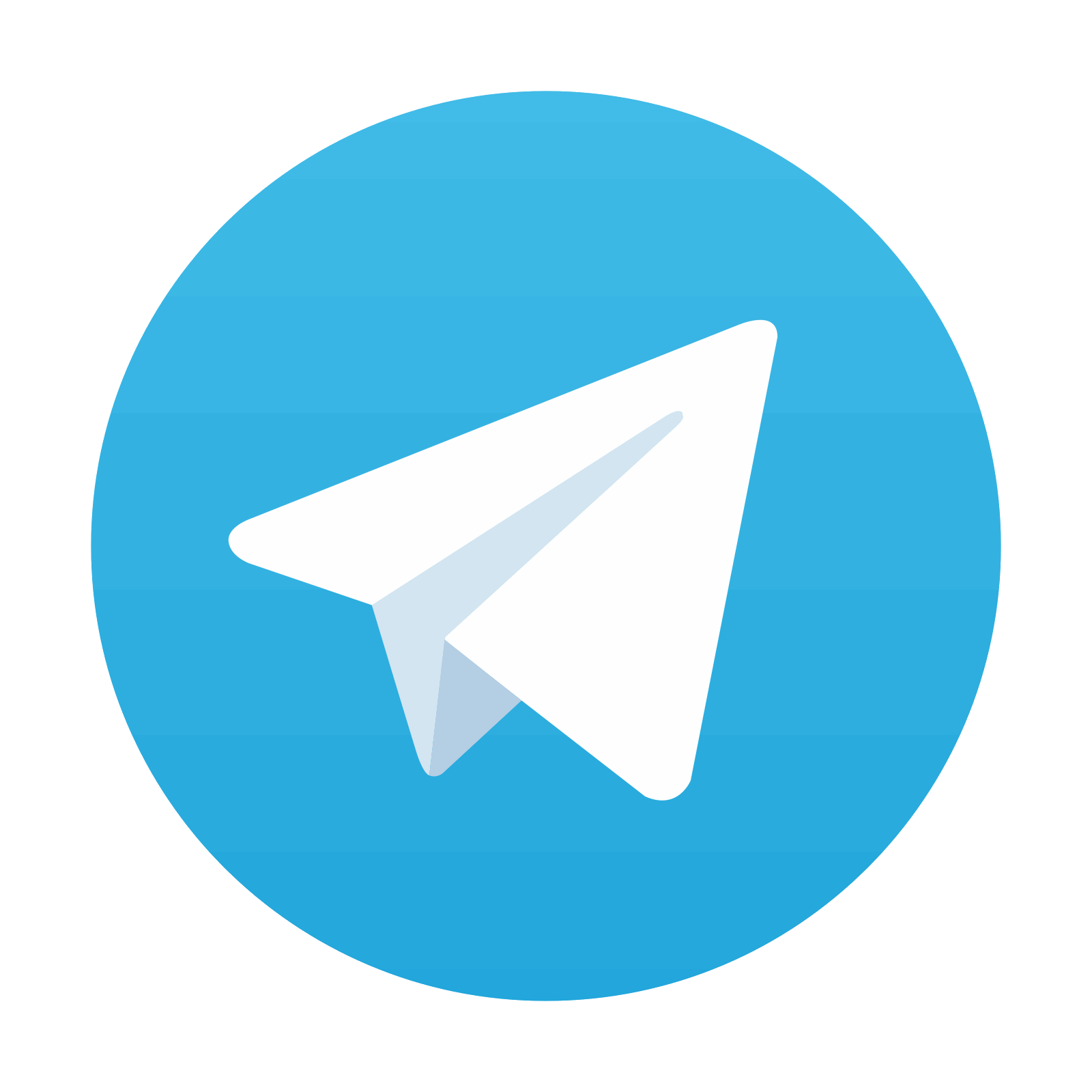
Stay updated, free articles. Join our Telegram channel

Full access? Get Clinical Tree
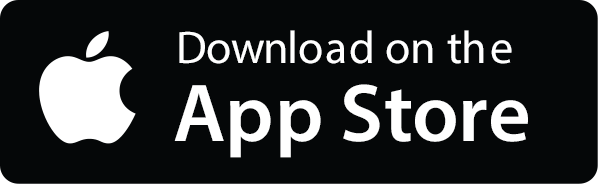
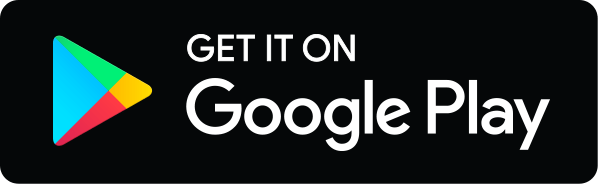