Fig. 1.1
Graph showing the incidence of open-angle glaucoma by intraocular pressure (IOP) (Reprinted from [7]. With permission from Elsevier)
IOP as a Function of Aqueous Humor Production and Outflow
Intraocular pressure can be expressed using the modified Goldmann equation [19], which consists of four elements:
where F is the aqueous humor formation rate in microliters per minute, C is the facility of outflow in microliters per minute per millimeter of mercury, Pv is the episcleral venous pressure in millimeters of mercury, and U is the rate of outflow of aqueous humor via all channels that are intraocular pressure independent.

Aqueous humor is produced by the ciliary body [20]. The ciliary processes contain a fibrovascular core and are covered by a bilayered epithelium. This double layer is the result of the optic cup invagination during embryogenesis. As it is joined apically, the basement membrane of the inner, nonpigmented epithelium is facing the posterior chamber. The vasculature is connected to the major circle of the iris [21]. Blood flows toward the network of choroidal veins and exits through the vortex veins. A sphincter-like system regulates blood flow that allows adjustment of filtration pressure and, consequently, aqueous humor production to some extent [22–24]. The capillaries from the anterior arteriole in the stromal core of the ciliary processes are lined by fenestrated endothelial cells, allowing the passage of macromolecules, ions, and water. The ciliary nonpigmented epithelium in contrast has zonulae occludentes, adherentes, and desmosomes [25, 26] constituting the blood–aqueous barrier of the ciliary body [27]. It is primarily the epithelium at the tip of the ciliary processes that contains carbonic anhydrase and the Na–K pump, an ATPase, to actively produce aqueous humor.
Aqueous humor leaves the eye via two routes: (1) the conventional outflow tract by passing through trabecular meshwork (TM) into Schlemm’s canal and subsequently following circuitous channels toward the surface of the sclera into the episcleral vasculature and (2) the uveoscleral outflow tract by absorption into uveal tissues [28, 29].
Most regard the juxtacanalicular tissue or the inner wall of Schlemm’s canal as the primary source of outflow resistance [30]. Past suggestions that increase in outflow resistance in primary open-angle glaucoma (POAG) derive from the accumulation of extracellular matrix material in the open spaces of the uveal and corneoscleral trabecular meshwork have recently been shown to be problematic as these decrease with age and in POAG [31, 32]. Aqueous humor passes through the juxtacanalicular trabecular meshwork and the inner wall of Schlemm’s canal through micropores and unique giant vacuoles that form in a pressure-dependent and energy-independent fashion [33, 34]. Around 30 external collector channels drain from the outer wall of Schlemm’s canal toward the scleral surface into a deep scleral plexus that leads to the deep scleral veins and eventually to the episcleral veins. Despite this small caliber vascular network and its ability to regulate flow [35], this effect is relatively insignificant. Several unique vessels, termed the aqueous veins of Ascher, bypass the deep scleral plexus directly into the episcleral veins [36].
Under physiological conditions, 70–95 % of outflow occurs through the trabecular meshwork and is pressure dependent [37–40]. The pressure-independent uveoscleral outflow can increase significantly during inflammation to 60 % [28, 29]. Pharmacological enhancement of uveoscleral outflow into the supraciliary space was first demonstrated in humans with prostaglandin acid prodrugs [40, 41]. Despite increased leakiness of the ciliary body vasculature, intraocular pressure is usually decreased during intraocular inflammation because of increased uveoscleral outflow and disappearance of gap junctions that serve intercellular metabolic and electronic coupling of ciliary epithelial, resulting in decreased aqueous humor production [42, 43].
IOP can be decreased through the suppression of aqueous production with carbonic anhydrase inhibitors [44], beta-adrenergic antagonists [45], and alpha-adrenergic agonists [46]. The only medication class in clinical use that increases conventional outflow, the parasympathomimetics [47], has been all but abandoned because of its side effects and potential to reduce uveoscleral outflow [28]. Trabeculoplasty has been employed to lower intraocular pressure by inducing remodeling of the TM and extracellular matrix [48]. However, the extent of pressure reduction is much smaller than can be achieved with filtering procedures. Complete disruption of the TM and wall of Schlemm’s canal has recently been achieved with the trabectome [49], but this disruption is irreversible, creating a permanently open connection to the downstream drainage system. Therapies for advanced glaucoma traditionally attempt to lower intraocular pressure surgically by circumventing the outflow resistance of the TM by shunting aqueous humor to the sub-Tenon space (e.g., trabeculectomy, aqueous drainage device) [50]. These filtering procedures have a high rate of complication and failure [51] despite incremental advances in surgical technique, including the introduction of antifibrotics and improvement of drainage device design, respectively.
Important Randomized Clinical Trials
Collaborative Normal Tension Glaucoma Study
Before the Collaborative Normal Tension Glaucoma Study (CNTGS) [8], it was not known whether IOP that was in the normal statistical range was at all involved in glaucomatous optic nerve damage and visual field loss. The study demonstrated the risk of progression was significantly reduced in eyes randomized to treatment that achieved a ≥30 % reduction IOP compared to control, untreated eyes. The most treatment benefits were observed in patients of female gender, with family history of glaucoma, without family history of stroke, without personal history of cardiovascular disease, and with mild disc excavation [11]. Risk factors for progression were female gender, migraine headaches, and optic disc hemorrhages [8, 11]. The natural course as well as the response to treatment can be highly variable, and treatment has to be individualized according to the stage of disease and rate of progression if the mentioned risk factors are not present [11, 52].
Advanced Glaucoma Intervention Study
The Advanced Glaucoma Intervention Study (AGIS) [53] has to be seen in its historical context. The goal of AGIS was to compare trabeculectomy with argon laser trabeculoplasty (ALT) in eyes that had failed medical management at a time when it was not established that ALT was less effective than trabeculectomy. Before 1980, trabeculectomy was the usual intervention for medically failing advanced glaucoma. AGIS established a benefit from lowering IOP in glaucoma by trabeculectomy [54]: Eyes with early average intraocular pressure greater than 17.5 mmHg had more progression than eyes with average intraocular pressure less than 14 mmHg. When IOP was less than 18 mmHg during all visits over 6 years in a separate analysis, mean changes from baseline in visual field defect scores were close to zero. A shortcoming is that AGIS was not originally designed to detect the benefits of different degrees of IOP reduction in the prevention of glaucoma progression. These effects were detected in a post hoc analysis.
Collaborative Initial Glaucoma Treatment Study
The Collaborative Initial Glaucoma Treatment Study (CIGTS) [55] compared treatment of newly diagnosed POAG with standard medical treatment (typically initial beta-blocker) versus filtration surgery. In the medication treatment arm, additional topical medications could be added, followed by ALT and filtration surgery if needed. In the surgical treatment arm, failed trabeculectomy could be followed by ALT. The surgical group had IOPs that were 2–3 points lower than the medical group. Surprisingly, despite the lower IOP, the surgical group had more visual field and more visual acuity loss than the medical group in the first 3 years, but this difference disappeared in the follow-up [56]. There were 3.8-fold more cataract extractions in the surgical group [57] in the initial 5 years after trabeculectomy but not thereafter. While the surgical group complained about foreign body sensation at the bleb site, the medical group experienced more systemic symptoms. Results from CIGTS did initially not support altering treatment practices of initial medical management of patients with primary open-angle glaucoma [58], but the most recent follow-up showed better outcomes in the trabeculectomy group among patients with more advanced visual field loss [59].
Ocular Hypertension Treatment Study and the European Glaucoma Prevention Study
The purpose of the Ocular Hypertension Treatment Study (OHTS) [9] was to determine whether the pharmacological reduction of elevated IOP can prevent glaucoma and to define risk factors for glaucoma development. Topical ocular hypotensive medication was effective in delaying or preventing onset of POAG in individuals with elevated IOP by about 50 %. Results to date have shown an approximate 50 % reduction in conversion from OHT to POAG, with a 20 % reduction in intraocular pressure [60]. OHTS demonstrated that medical treatment of people with intraocular pressure of ≥24 mmHg reduces the risk of the development of primary open-angle glaucoma (POAG) by 60 % [61].
Factors that predicted the development of POAG included older age, race (African American), sex (male), larger vertical cup–disc ratio, larger horizontal cup–disc ratio, higher intraocular pressure, greater Humphrey visual field (VF) pattern standard deviation, heart disease, and thin central corneal thickness [62]. Reduction in IOP in the medication group was 22.5 ± 9.9 % versus 4.0 ± 11.6 % in the observation group. At 60 months, the overall probability of developing POAG was 4.4 % in the medication group and 9.5 % in the observation group [60]. Of African-American participants, 8.4 % developed POAG in the medication group when compared with 16.1 % in the observation group [63]. The occurrence of an optic disc hemorrhage was associated with an increased risk of developing a POAG end point in participants in the OHTS. However, most eyes (86.7 %) in which a disc hemorrhage developed have not developed POAG to date. The 96-month cumulative incidence of POAG in the eyes without optic disc hemorrhage was 5.2 %, when compared with 13.6 % in the eyes with optic disc hemorrhage [64].
The same predictors for the development of POAG were identified independently in both the OHTS observation group and the European Glaucoma Prevention Study (EGPS) [65]: placebo group-baseline age, intraocular pressure, central corneal thickness, vertical cup-to-disc ratio, and Humphrey VF pattern standard deviation [66]. There was no evidence for a general effect of topical ocular hypotensive medication on lens opacification or visual function [67]. Results of EGPS have to be interpreted with caution as several design problems might have caused the unexpected finding that IOP was lowered in the placebo control group as well: (1) a high patient dropout rate, especially in the dorzolamide arm; (2) commitment to dorzolamide treatment regardless of therapeutic effect; and (3) determination of baseline pressure with just two readings that could have been merely 2 h apart, increasing the confounding impact of regression to the mean IOP in the placebo arm [68].
Early Manifest Glaucoma Trial
The Early Manifest Glaucoma Trial (EMGT) [10] compared how glaucoma progression was affected by immediate combined medical (betaxolol) and laser therapy for newly diagnosed OAG with normal or moderately elevated IOP versus late or no treatment. Treatment caused an average reduction of IOP of about 5 mmHg (25 %), which reduced glaucoma progression to 45 % when compared with 62 % in the control group and occurred later. These benefits were preserved after stratifying for age, ethnicity, and glaucoma stage and type. The percent of patient follow-up visits with disc hemorrhages was also related to progression (hazard ratio = 1.02 per percent higher) [69]. Progression risk decreased by about 10 % with each millimeter of mercury of IOP reduction from baseline to the first follow-up visit (HR = 0.90 per millimeter of mercury decrease) [69, 70]. Elevated IOP was a strong factor for glaucoma progression, but intraocular pressure fluctuation was not [70]. Higher IOP, exfoliation, bilateral disease, and older age were previously identified risk factors for progression [71]. New baseline predictors were lower ocular systolic perfusion pressure (≤160 mmHg; HR 1.42), cardiovascular disease history (HR 2.75) in patients with higher baseline IOP, and lower systolic blood pressure (BP) (≤125 mmHg; HR 0.46; 95 % CI 0.21–1.02) in patients with lower baseline IOP. Lower central corneal thickness (CCT) (HR 1.25 per 40 μm lower) was a new significant factor, a result observed in patients with higher baseline IOP.
Conclusion from Randomized Trials
Therapeutic IOP reduction does delay or prevent progression to glaucoma among patients with ocular hypertension and reduces the risk of progression among patients with glaucoma. Another conclusion, however, is that despite good IOP control in many actively treated patients, POAG can still progress and can result in blindness in an unacceptably large number of patients [72, 73].
IOP as Risk Factor for Glaucoma Development
The role of IOP in the diagnosis of glaucoma is different from its role in treatment. Because of the wide range of IOP that can be found interindividually as well as intraindividually (as will be discussed), a single IOP measurement above the expected average has little merit. Glaucoma risk calculators reflect the complexity of multiple other glaucoma risk factors that bear equal or greater significance. At least one variable – CCT – influences IOP measurements directly and must be taken into account when judging IOP that was measured clinically with the most common technique: Goldmann applanation tonometry. In addition, CCT may be an independent risk factor reflecting ocular biomechanical characteristics, including that of the lamina cribrosa. A recent histological study questioned this theory when no correlation was found in non-glaucomatous human globes between CCT and lamina cribrosa and peripapillary scleral thickness [74] a conclusion similar to that of a study performed with ocular coherence tomography (OCT) [75] that also found no correlation between CCT and axial length in healthy eyes. Histological artifact and sectioning methods could partially account for the lack of a predicted association. CCT instead inversely correlates to optic disc size in Caucasians [76] suggesting that – following Laplace’s law – a disc with a larger radius would be more deformable and vulnerable than a small one. Measurement of CCT is also considerably more reliable in one sitting [77] and stable over the lifetime of an adult.
Clinical Pearl: Predicting Glaucoma Risk
There is a high degree of variability in ophthalmologists’ skills at estimating an individual’s risk of conversion from OHT to OAG based on knowledge of important risk factors. In comparison to OHTS glaucoma risk calculations, treating physicians tend to underestimate the risk of conversion to glaucoma by almost twofold on average [78]. This can lead to either under- or overtreatment of patients.
Clinicians need a more exact method to determine the probability of conversion to glaucoma from ocular hypertension. Gordon et al. have recently developed a point system (Table 1.1) that can be more easily used than risk calculators for estimating the 5-year risk of conversion from OHT to POAG [66].
Table 1.1
Glaucoma risk calculator
Points for baseline predictor | |||||
---|---|---|---|---|---|
Baseline predictor | 0 | 1 | 2 | 3 | 4 |
Age (years) | <45 | 45 to <55 | 55 to <65 | 65 to <75 | ≥75 |
Mean IOP (mmHg) | <22 | 22 to <24 | 24 to <26 | 26 to <28 | ≥28 |
Mean CCT (μm) | ≥600 | 576–600 | 551–575 | 526–550 | ≤525 |
Mean vertical cup-to-disc ratio by contour | <0.3 | 0.3 to <0.4 | 0.4 to <0.5 | 0.5 to <0.6 | ≥0.6 |
Mean PSD (dB) | <1.8 | 1.8 to <2.0 | 2.0 to <2.4 | 2.4 to <2.8 | ≥2.8 |
Sum of points | 0–6 | 7–8 | 9–10 | 11–12 | >12 |
Estimated 5-year risk of POAG | ≤4.0 % | 10 % | 15 % | 20 % | ≥33 % |
How to Measure Intraocular Pressure
Clinical Pearl: Instruments to Measure IOP
Goldmann applanation tonometry (GAT) is the standard for daily clinical use because it is easy to perform and inexpensive and allows comparison to other glaucoma clinics and trials.
The pneumatonometer is a standard for clinical research because of high precision and retest reliability but lacks widespread deployment largely due to device size and cost.
The latest generation Tono-Pen is nearly as precise as GAT and can easily be integrated in the workup by support staff prior to patient–physician contact.
Dynamic contour tonometry makes measurements less dependent on CCT. Its use remains limited because of costs and need for longer measurements. Recording of the pulse amplitude can be useful in select cases, but its significance in daily practice is marginal.
The latest noncontact tonometers are highly accurate, provide information about additional biomechanical properties that are directly related to glaucoma, and can be used with little training. Measurements create potentially infectious aerosol. The anticipation of the puff is uncomfortable to most patients and can produce lid artifacts from squeezing.
Schiotz tonometry is best employed as a reserve technique when other instruments are not available or in the OR when the entire device has to be sterile.
Central corneal thickness (CCT) should be determined at one of the first visits but considered an independent risk factor.
Transpalpebral scleral IOP palpation is a valuable skill for difficult eyes. This can be learned by performing digital estimation after IOP was measured with an instrument.
No direct correction of GAT by central corneal thickness is possible. GAT IOP and CCT can be integrated using a pressure-to-cornea index (IOP/(CCT^3); normal PCI = 120–140. CCT can also be classified as thin, normal, or thick.
Goldmann Applanation Tonometry
Goldmann applanation tonometry (GAT, Haag-Streit AG, Bern, Switzerland) is the standard method of measuring IOP [79, 80] to which all other ones are compared. GAT was introduced by Goldmann in 1954 [81, 82] and applies the Imbert-Fick law that describes the applanation force of an ideal sphere to applanation of the cornea – a method that is more accurate than older indentation systems. Applanation occurs to an area of 7.35 mm2 so that tear capillary action counteracts the “rigidity” of the cornea. GAT produces precise and repeatable IOP readings with an interobserver variation of 11 % and an intra-observer variation of 9 % when compared with a single measurement [83]. It has remained the standard because it is simple and widely available with a popular slit-lamp system that allows comparison of historical measurements including ones obtained by others. GAT can be integrated well into the exam algorithm, as the fluorescein that is used is also helpful in evaluating the ocular surface. GAT displaces only about 0.5 mL of fluid, while Schiotz and other indentation techniques displace about 15–20 mL, depending on ocular rigidity. GAT is influenced by central corneal thickness, curvature, structure, and ocular axial length [84].
No reliable nomogram exists that converts GAT and CCT into true IOP, although attempts have been made to develop a conversion factor [85–90]. Doughty et al. found in a meta-analysis that a 10 % difference in CCT would result in a 3.4 ± 0.9 mmHg difference in IOP in 133 data sets of all eyes but only 1.1 ± 0.6 mmHg in healthy eyes [88]. Others could not confirm a systematic error that would allow direct conversion of applanation tonometry readings with increasing CCT when compared to intracameral measurements indicating that the relationship is parametric and nonlinear [91]. In fact, the predictive accuracy of the prediction model of glaucoma development in the OHTS/EGPS model could not be improved when IOP was corrected for CCT with any of the above formulas [92]. GAT IOP and CCT (thin, normal, and thick) are best treated as two independent risk factors or integrated without calculation of “true” IOP. This can be done, for instance, by using a recently described pressure-to-cornea index (PCI) [93]. PCI can be calculated as IOP/CCT^3. A PCI range of 120–140 is proposed as the upper normal limit.
The influence of CCT on GAT can be especially challenging after refractive surgery. Other disadvantages of GAT are that it requires more user skill than other methods, it can be falsely high in anxious or obese patients second to Valsalva maneuver, and the device must be sent in for calibration when a preset weight indicates inaccurate readings. GAT readout is also user dependent: Most examiners will use the IOP during the ocular pulse diastole, while others choose to estimate IOP between systole and diastole.
Tono-Pen Tonometry
A small handheld device, the Tono-Pen AVIA (Mentor, Santa Barbara, California, USA) uses the same principle as GAT, and its development has a comparably long history, dating back to the Mackay-Marg tonometer in 1960 [94, 95]. While prior studies comparing Tono-Pen tonometry with other methods produced mixed results [96], measurements with the later generation of Tono-Pen correlate better with GAT [97], but may overestimate IOP compared to GAT when CCT is greater than 520 μm and underestimate IOP when CCT is less than 510 μm [98].
In addition to IOP, the Tono-Pen determines the confidence interval of several measurements. Because of a smaller area of applanation (2.36 mm2) than GAT (7.35 mm2), the Tono-Pen is less dependent on CCT in theory [87], allows valid intraocular pressure measurements in the presence of corneal edema and irregularities [99], and has even been used to measure through soft contact lenses [100, 101]. Measurements can be performed while the patient rests back in the chair, avoiding false high readings from increased intra-abdominal pressure, e.g., in obese patients when leaning forward. In contrast to GAT, which cannot be adjusted by the user, the Tono-Pen self-calibrates. Support staff can obtain accurate readings with ease prior to physician encounter.
Dynamic Contour Tonometry
In dynamic contour tonometry (DCT) (Pascal dynamic contour tonometer; Swiss Microtechnology AG, Port, Switzerland), both IOP and ocular pulse amplitude can be read on a display. It uses a solid-state pressure sensor built into the center of a contoured, concave tip surface. The contour surface is designed to minimize distortion and to direct all forces acting within the cornea to the pressure sensor surface. The tonometer head allows the cornea to assume the shape it naturally adopts when pressure on both sides of the cornea is equal, which constitutes the measurement end point. A microprocessor determines the intraocular pressure and the ocular pulse amplitude. DCT is very similar to GAT, but does not vary with decreased CCT and has a lower intra-and interobserver variability [102]. This is of particular interest after LASIK when GAT, but not DCT, produces lower IOP readings due to a thinner and more oblate cornea [103]. Both DCT and the ocular response analyzer (ORA) can be used well in keratoconus, where corneal hysteresis and corneal resistance factor are of more importance than CCT as biomechanical properties that cause inaccuracies in applanation tonometry techniques. DCT does not have any benefit over GAT in thick corneas, however [104], and only between 540 and 545 μm there is good agreement [105]. Measurements take approximately three times longer, require two measurements to succeed, and are less comfortable for patients [106].
Transpalpebral Pressure Estimation
Transpalpebral intraocular pressure estimation by palpation of the sclera in down gaze is an invaluable tool that has many advantages: It requires little patient cooperation, circumvents corneal pathology, is fast, and can be used without any equipment. In the operating room, direct corneal palpation might be the only available form of tonometry. Experienced observers are able to estimate IOP surprisingly accurately within only 2 mmHg [107] to 5 mmHg [108] median deviation from GAT, although IOPs below 14 mmHg may be overestimated [107]. In other hands, palpation may only be helpful in identifying IOPs above 30 mmHg [109]. It is best to practice and correlate palpation and GAT in daily practice.
The most common transpalpebral tonometer is the Diaton (JSC GRPZ, Ryazan, Russia). As prior models, the current version has the same shortcoming that biomechanical properties of the eyelid and tarsus are part of the IOP measurement. The Diaton is based on rebound speeds of a falling rod [110].
Rebound Tonometer
Rebound tonometry utilizes an accelerated lightweight probe to make momentary contact with non-anesthetized cornea. An induction-based coil system then measures motion parameters of the probe and its deceleration and contact time which change as a function of IOP and corneal biomechanical properties. Similar to air puff-based tonometers, the advantage of this technology is that it can be performed rapidly without anesthetic and before the blink reflex is triggered [111]. It is also easy to use and portable [112] and performs reliably while producing measurements similar to GAT [113, 114]. This has led to adoption not only in pediatric ophthalmology [111, 115, 116] but also for home use [117].
Noncontact Tonometry
A cited advantage of air puff tonometry is the absence of contact with the measured eye, which in theory makes the exam more comfortable as no anesthetic drops are needed, and there is no risk of a corneal abrasion by untrained personnel. In reality, many patients find the anticipation of multiple puffs uncomfortable, which can lead to false high-pressure measurements because of lid pressure. The air puff generates aerosol that potentially distributes infectious agents [118]. While earlier noncontact tonometry was inaccurate when compared with Goldmann tonometry, earning the method a bad reputation, recent devices have a high accuracy that approaches GAT [119–121]. Noncontact tonometry is more influenced by biomechanical properties such as corneal hysteresis, resistance factor, and CCT than other methods [122]. It has been reported to be performed with sufficient accuracy over soft contact lenses of less than 0.30 mm thickness and less than +3D power [123].
A newer generation noncontact tonometer, the ocular response analyzer (ORA, Reichert, Depew, NY) provides IOP values that are independent of corneal properties [124, 125] and – due to a 10 microsecond rather than 1–3 microsecond air puff – can provide additional information about corneal properties that may help to measure IOP in keratoconus [126]. Low corneal hysteresis is a biomechanical parameter that the ORA can measure which is associated with more rapid glaucomatous visual field progression [127] and increased deformation of the lamina cribrosa during transient elevations of IOP [128].
Pneumatonometry
The pneumatonometer (Model 30 Classic Pneumatonometer, Reichert Ophthalmic Instruments, Depew, New York, USA) is a high-displacement tonometer [129] that can also be used to measure outflow facility [130]. It must not be confused with the noncontact air puff tonometer. Its accuracy is exceeded by that of the Goldmann tonometer [131]. IOP measurements have only half the standard deviation of the Perkins handheld applanation tonometer [132] and are better than the Schiotz tonometer [133]. Another advantage is that – similar to an electrocardiogram strip – a live printout of IOP over time can be obtained that allows measuring and visualizing IOP fluctuations. The pneumatonometer tends to underestimate IOP above 40 mmHg [134]. IOP measurements are independent of the central corneal thickness after LASIK [135] and not significantly affected by bandage contact lenses [136]. Both pneumatonometer and Tono-Pen measurements are not affected by whether the orientation is vertical or horizontal [137].
Schiotz Tonometry
The Schiotz tonometer [138] is another displacement tonometer that is less accurate and influenced by the curvature and thickness of the cornea as well as by overall ocular rigidity. Because it is a small handheld, sterilizable full metal instrument that requires no batteries, it may be useful in the operating room or as a backup instrument.
Distribution of IOP and Factors Influencing IOP
Distribution of IOP Within the Population
IOP has a non-Gaussian distribution and is skewed to higher pressures (especially among subjects over 45 years of age [139] and more markedly so in women [140].) In a primarily Caucasian population in the USA, mean IOP was 16 mmHg (SD ±3 mmHg) [139]. The Baltimore Eye Survey found no difference in IOP among normal African Americans when compared with normal Caucasian subjects [3]. In the Barbados Eye Study, IOP was found to be higher among African Caribbeans [18 mmHg (SD 4 mmHg)] [141] when compared with Caucasians, 14.5 mmHg (SD 2.6 mmHg) in Iran [142], 16.11 mmHg (SD 3.39 mmHg) in Northern China [143], 11.7 mmHg (SD 2.5 mmHg) in Japan [144], and 15.5 mmHg [145] in West Africa. Myopic and more darkly pigmented subjects also have a higher average IOP [142]. There is no upper IOP that can be defined as safe for the entire population below which no glaucomatous optic neuropathy occurs. This is exemplified by an average IOP of 15.4 mmHg (SD 2.8 mmHg) of Japanese POAG subjects in the Tajimi study [146] that is below or similar to the previously mentioned groups. There was a surprisingly high prevalence of POAG (3.9 %) among participants, 92 % of whom had an IOP ≤21 mmHg.
Factors Influencing Intraocular Pressure
IOP is influenced by various external and internal factors that include time of the day and year, heartbeat, respiration, exercises, and fluid intake. Systemic medications and topically applied drugs can also have an impact. Measurement of IOP by the widespread Goldmann applanation tonometry is dependent on the central corneal thickness as detailed previously.
Clinical Pearl: Factors Influencing Intraocular Pressure
IOP measurements are significantly dependent on central corneal thickness in Goldmann applanation tonometry: A thick cornea will lead to overestimation and a thin cornea to underestimation.
Variations of IOP by season, cardiac pulse wave, or respiration are of no practical clinical significance to the practitioner. A diurnal pressure curve can be utilized to get a better idea of the range of IOP an eye is subjected to. Always note the time of the day with each IOP measurement.
Be aware that you are measuring office IOP, which may be lower than IOP at home as a result of increased compliance before the visit.
Optimization of exercise, cessation of recreational drugs, and improvement of nutrition should always be encouraged, but must never be falsely prioritized.
The possibility of progressive visual field defects secondary to Valsalva maneuvers when playing high-resistance wind instruments or during extreme postural changes such as in yoga should be pointed out clearly to glaucoma patients. IOP measured in the supine position allows estimation of nocturnal IOP in untreated glaucoma patients.
The water-drinking test can be used to estimate the highest naturally occurring IOP but must be used with caution in cardiac or renal patients as fluid overload and electrolyte shifts can occur rapidly.
Nightly systemic hypotension may result in cerebral and optic nerve hypoperfusion. Consider using topical beta-blockers only in the morning in such individuals and discuss the option of using systemic beta-blockers only in the morning in individuals who take them not primarily for blood pressure control (congestive heart failure, migraine prophylaxis).
In the absence of any available medication, a good Scotch or other high-percentage alcoholic beverage can be consumed to emergently treat acutely vision-threatening high IOP.
Circadian and Seasonal Variation
The diurnal (daytime) IOP varies by 2–6 mmHg in normal individuals. Pressure peaks most commonly occur in the morning hours but may be seen in the afternoon, evening, or sleep, especially if one measures IOP in the habitual position (sitting during the day, supine at night). Some individuals have no reproducible pattern. Generally, higher amplitude fluctuations are seen in higher mean IOP and in individuals with POAG [147] and greater in progressive visual field loss than in the presence of stable fields [148]. The reason for higher IOP during the day is that the rate of aqueous humor formation has a circadian variation as well and is twice as high during the day as at night [149] as a result of endogenous catecholamines [150, 151]. Measurements of fluctuations to create a diurnal curve can be used to detect normal tension glaucoma, in which pressure peaks may contribute to progressive glaucomatous optic neuropathy, despite well-controlled average pressures at prior office visits. However, fluctuation itself is not significantly associated with a higher risk of progression to glaucoma in untreated ocular hypertensives [152]. In contrast to this study, Lee et al. found in a cohort study that each unit increase in IOP standard deviation resulted in an approximately five times higher risk of glaucoma progression [153]. Although variations of IOP in the left and right eye are similar, the strength of association is only moderate, which makes one-eye therapeutic trials difficult to assess by single pairs of right and left IOP measurements [154]. IOP is higher in winter months by about 3 mmHg and within the range of the diurnal variations [155].
Cardiac Pulse Wave
As the cardiac pulse wave is transmitted by the carotids to the ocular circulation, it manifests in the choriocapillaris as pulse-synchronous IOP oscillations. The relationship between ocular perfusion pressure and intraocular pressure is more complicated because of autoregulation within a physiological range of optic nerve head circulation, central retinal artery, and choroidal perfusion. This dynamic autoregulation is impaired in glaucoma [156]. Counterintuitively, IOP might decrease rather than increase with increased perfusion pressure [157]. This is different from other tissues (e.g., the kidney) because active secretion vastly exceeds passive filtration in aqueous humor production. It is difficult to study this relationship because ocular perfusion pressure cannot be changed selectively without affecting ocular blood return, ciliary body tension, etc. in vivo by pharmacological or physical means.
Respiration and Valsalva Maneuver
Short-term IOP changes occur with breathing as the result of acute changes of perfusion pressure [158].With higher pressure than normal breathing – e.g., from high-resistance wind instruments (trumpet, oboe) – uveal engorgement becomes the major factor [159]. This increase is not trivial: Professional high-resistance musicians who are exposed to frequent and longer-lasting, high-amplitude IOP spikes have a significantly greater incidence of visual field loss with characteristic glaucomatous damage [159]. During only 12 s, IOP can rise from 24 to 46 mmHg [159]. In contrast, decreased venous drainage from increased episcleral venous pressure as a result of high intra-abdominal pressure would be driven by aqueous humor production and would take 10–16 min in theory [159].
Postural Changes
IOP also varies with postural changes as a result of increased episcleral venous pressure [160] as well as increased choroidal volume by vascular engorgement [161]. The relationship between the head-down body position and increased IOP is well known [162–166]. Nocturnal IOP in untreated glaucoma patients can be estimated during routine office visits by measuring the supine position [167]. Upside-down body changes such as in certain yoga poses can cause extreme IOPs and can result in visual field progression [160, 168].
Physical Exercise
The IOP decreases significantly after dynamic exercise, although heart rate and systolic artery pressure increase [169]. Different from the sustained high intrathoracic pressure when playing wind instruments, IOP increases only by about 4 mmHg with breath holding in resistance training (e.g., bench press) compared to about 2 mmHg with normal breathing [170]. While there is an immediate IOP spike after exercise, decrease is seen 30 min later and lasts for 2 h after exercise [171]. During aerobic exercise, duration of the systolic and diastolic phases of the intraocular pulse shortens, and the pulse amplitude and volume decrease, and total ocular blood flow increases by 18 % [157].
Fluid Intake
IOP and hydration status are related. While low IOP in hypovolemia can be used to estimate extent of dehydration [172]. a fluid bolus intake can increase IOP. The water-drinking test has been used for a long time to detect peak IOPs that can be elusive during the day in the diagnosis of glaucoma [173].
Because recent studies have demonstrated that the amplitude of fluctuation correlates to the severity of glaucoma [174] and to visual field progression [175], this test has regained interest. Water-drinking test peak pressures and peak pressures seen in a normal diurnal pressure curve correlate [176, 177]. Change of IOP after the water-drinking test is one identified significant risk factor as are outflow facility, age, IOP, and cup-to-disc ratio. Yoshikawa et al. [176]. found that the water-drinking test could predict the progression of visual field loss also in normal tension glaucoma.
Nutrition and Recreational Substances
Patients frequently ask about the influence of nutrition, recreational substances (caffeine, alcohol, nicotine, marijuana) or blood pressure on IOP. Although there is evidence for a minimal but statistically significant increase of IOP after high amounts of caffeine [178] and smoking [179], this has not been proven to be a significant factor in the context of glaucoma. There is a large school of thinking focused on improving optic nerve head perfusion with smoking cessation, aspirin, calcium antagonist, ginkgo extracts, or magnesium supplements, but this is not supported by convincing data. Alcoholic beverages can lower IOP and have been used to break acute pressure spikes, e.g., in angle closure [180, 181]. Cannabinoids also have a moderate effect and lower IOP by about 5 mmHg for 4–6 h [182–184].
Medications Influencing Intraocular Pressure
Clinical Pearl: Drug-Induced Intraocular Hypertension and Glaucoma
Corticosteroids increase IOP in up to 36 % of healthy and up to 92 % of POAG patients. This may not be reversible after cessation.
This can occur via all routes of exposure, not just ocular or oral.
More potent steroids cause a faster and higher IOP increase.
From low to high 163: medrysone 1.0 % = 1.0 mmHg, tetrahydrotriamcinolone 0.25 % = 1.8 mmHg, hydrocortisone 0.5 % = 3.2 mmHg, fluorometholone 0.1 % = 6.1 mmHg, dexamethasone 0.005 % = 8.2 mmHg, prednisolone 1.0 % = 10.0 mmHg, and dexamethasone 0.1 % = 22 mmHg.
Corticosteroids
The list of topical and systemic drugs that can increase IOP and cause glaucoma is long. The most notorious ones are 43 corticosteroids (glucocorticosteroids more than mineralocorticosteroids) no matter their route of application: Steroid-induced ocular hypertension has been reported following topical application to the eyelids [185], chronic nasal or inhaled steroid [186], subconjunctival [187], and sub-Tenon’s injection of steroid [188–191]. Endogenous corticosteroids can have the same effect [191]. Corticosteroid-induced glaucoma was first described in 1950 after administration of adrenocorticotropic hormone and cortisone [192].Eighteen to 36 % of healthy individuals and 46–92 % of patients with POAG will respond with an elevation of IOP within 2–4 weeks [193]. Relatives of patients with POAG, angle recession, patients with diabetes mellitus, high myopia, and connective tissue disease (rheumatoid arthritis in particular) have a higher risk of steroid-induced intraocular hypertension. Thirty-one percent of children who receive prednisone for inflammatory bowel disease have a significant increase of IOP but respond well to dose reduction [193].
Chances of steroid-induced ocular hypertension increase with steroid potency. It is very low with rimexolone, loteprednol etabonate, fluorometholone, and medrysone, but this risk and extent of IOP rise increase with steroid potency 163 (medrysone 1.0 % = 1.0 ± 1.3 mmHg, tetrahydrotriamcinolone 0.25 % = 1.8 ± 1.3 mmHg, hydrocortisone 0.5 % = 3.2 ± 1.0 mmHg, fluorometholone 0.1 % = 6.1 ± 1.4 mmHg, dexamethasone 0.005 % = 8.2 ± 1.7 mmHg, prednisolone 1.0 % = 10.0 ± 1.7 mmHg, and dexamethasone 0.1 % = 22.0 ± 2.9 mmHg). Physicians must be aware that steroid-induced ocular hypertension might not be reversible after discontinuation of steroid therapy.
The pathophysiology of steroid-induced ocular hypertension is localized in the trabecular meshwork, but the mechanism is incompletely understood. More than 1, 000-fold upregulation of myocilin (MYOC – originally termed trabecular meshwork inducible glucocorticoid response [TIGR]) can be observed in trabecular meshwork with accompanying accumulation of polymerized glycosaminoglycans in the extracellular matrix [194]. It has been hypothesized but not proven that the primary means of action is a decreased funneling of aqueous humor toward the juxtacanalicular trabecular meshwork [195]. This mechanism is debated because myocilin mutants of some glaucomas act intracellularly and not primarily extracellularly [196, 197]. Other proposed mechanisms include direct swelling of TM cells from inhibition of Na-K-Cl cotransporter that is regulated in TM cells [198], decreased production of tissue plasminogen activator, collagenase IV, and stromelysin [199–203].
Medications Influencing Intraocular Pressure
Clinical Pearl: Drug-Induced Intraocular Hypertension and Glaucoma
Corticosteroids increase IOP in up to 36 % of healthy and up to 92 % of POAG patients. This may not be reversible after cessation.
This can occur via all routes of exposure, not just ocular or oral.
More potent steroids cause a faster and higher IOP increase. From low to high [204]: medrysone 1.0 % = 1.0 mmHg, tetrahydrotriamcinolone 0.25 % = 1.8 mmHg, hydrocortisone 0.5 % = 3.2 mmHg, fluorometholone 0.1 % = 6.1 mmHg, dexamethasone 0.005 % = 8.2mmHg, prednisolone 1.0 % = 10.0 mmHg, and dexamethasone 0.1 % = 22 mmHg.
Pupillary Block from Anticholinergics
Antipsychotics, antidepressants, antihistamines, antiparkinson medications, antispasmolytic agents, mydriatics, sympathomimetics, antiasthma medications (ipratropium) [205], and botulinum toxin (botulinum toxin can produce mydriasis if the ciliary ganglion is affected [206]) are drug classes that have the potential to increase IOP by angle closure secondary to their anticholinergic properties (except botulinum toxin). The prevalence of occludable angles in Caucasians 55 years of age or older in the Framingham study is 3.8 % [207], but the risk of this in a Vietnamese population was estimated to be as high as 47.8 % [208].
Pupillary Block from Phacomorphic Changes or Ciliary Body Rotation
Promethazine HCl, an H1 antihistamine, has been documented to also produce swelling of the lens [209] in addition to mydriasis. Salbutamol, an inhalation agent used in asthma, can raise IOP by increased production of aqueous humor by about 38 % [210]. Pupillary dilation may trigger the release of pigment and trabecular obstruction [211]. Other drugs that can induce phacomorphic changes or anterior rotation of the ciliary body are sulfa drugs such as acetazolamide [212–214], sulfamethoxazole/trimethoprim [213], indapamide, promethazine, spironolactone, isosorbide dinitrate, and bromocriptine [214]; tetracycline [213, 214]; corticosteroids; hydrochlorothiazide; penicillamine; quinine; metronidazole; isotretinoin; and aspirin [214].
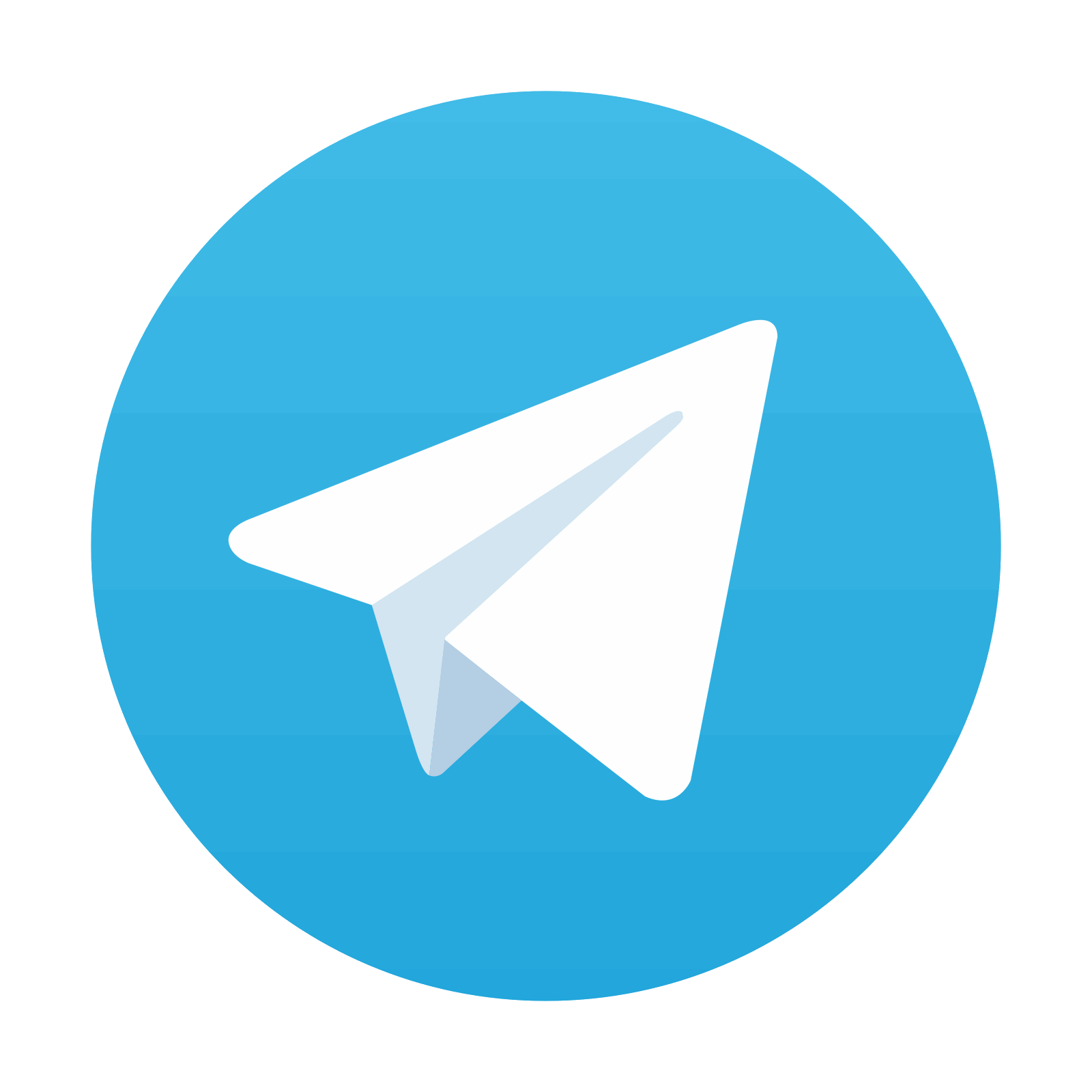
Stay updated, free articles. Join our Telegram channel

Full access? Get Clinical Tree
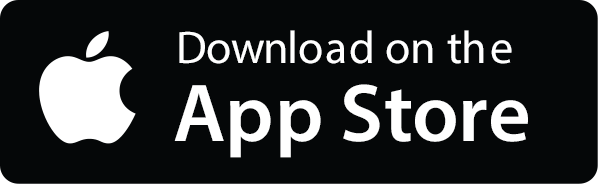
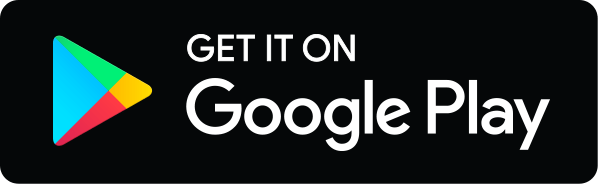