12
Speech Perception in Children with Cochlear Implants
Susan B. Waltzman
Multichannel cochlear implants were first used in children in the United States in 1987. Early results mainly focused on the ability of children with implants to obtain benefit when the prosthesis was used as an adjunct to speech reading or in situations where a finite number of alternatives were available as response choices (closed set). Postimplantation improvement was observed for suprasegmental features, consonant and vowel recognition, and word and sentence perception on closed-set tests (Osberger et al, 1991; Staller et al, 1991). Subsequently, numerous investigators examined the results over time in children who were both younger at time of implantation and congenitally/ prelingually hearing impaired. Concurrently, the focus shifted from postoperative performance on closed-set tests to the ability to perform on open-set measures where no alternative choices are provided and the results, therefore, are more closely aligned to unrestricted environmental listening conditions. Over the last several years, however, improvements in diagnosis of hearing loss, implant technology, processing strategies, surgical technique, programming options, and intervention approaches have proven that cochlear implants are a safe and effective treatment for children with sensorineural hearing loss leading to expanded criteria for implantation.
Children 2 years of age and older have been receiving implants since the late 1980s. The outcome of numerous investigations documented that pre-and postlinguistically deaf children of all ages could achieve significant open-set phoneme, word, and sentence recognition following cochlear implantation with a multichannel cochlear prosthesis beyond what could be achieved with conventional amplification. These results, coupled with advancements in cochlear implant technology and postoperative care, led to the implantation of children below 2 years of age. Again, performance in this age group revealed an increase in access to auditory stimuli leading to enhanced speech perception and oral language development. Despite the positive outcomes reported, the studies also revealed a wide range of performance. Postoperative auditory comprehension extended from the capability of using audition as the sole means of receptive communication to an ability to understand speech under restricted conditions to the need to augment auditory cues with sign language (Gantz et al, 1994; Waltzman et al, 1997). Indeed, monosyllabic word scores on tests designed for the pediatric population showed scores ranging from 0% to 100% at 1 year postimplantation, with pediatric cochlear implant recipients continuing to outperform children without implants using hearing aids on measures of speech perception (Meyer et al, 1998). Nevertheless, despite the use of less sophisticated speech processing schemes as compared with the current strategies, recent studies on long-term outcomes in the pediatric population have been surprisingly good. Waltzman et al (2002a) and Haensel et al (2005) reported continued improvement in speech perception over time with no deleterious effects in children implanted for 5 to 13 years.
♦ Factors Affecting Speech Perception in Children with Cochlear Implants
Despite the numerous positive results in pediatric cochlear implantation, published data still indicated a wide range of performance among implant recipients. Because the first question of parents of pediatric implant candidates often is “Which device is best for my child?,” there remains a need to study the possible variables that could account for the diversity of performance and affect ultimate outcome. An appreciation of the possible factors that influence results is critical to both the professionals dealing with the parents and to the parents/guardians themselves. It is not the purpose of this chapter to extensively review previously published results but rather to present for consideration a variety of factors, some of which have already been shown to influence outcome and others that warrant attention. Categorizing these determinants increases the ability of clinicians to offer educated preoperative prognoses to the families and might potentially allow for manipulation of variables in an attempt to achieve the best possible outcome. (Table 12–1) is a list of possibilities. The task of considering 22 potential intertwining variables becomes less daunting when one realizes that many of them are closely aligned and can be grouped together as units. The first variable, however, stands alone as to significance and influence.
1. Implant technology |
2. Surviving neural population |
3.Auditory (sensory) deprivation |
4. Auditory pathway development |
5. Plasticity of the auditory system |
6. Length of deafness |
7. Age at time of implantation |
8. Etiology of deafness |
9. Preoperative selection criteria |
10. Preoperative hearing level |
11. Preoperative auditory speech perception |
12. Measures of speech perception (preoperative and postoperative) |
13. Preoperative linguistic level: spoken language/manual language |
14. Other handicaps |
15. Surgical issues |
16. Device programming |
17. Device/equipment malfunctions |
18. Mode of communication |
19. Auditory input |
20. Frequency/type of training |
21. (Pre) school environment/education setting |
22. Parental/family motivation, social issues |
Implant Technology
Since initial research began on the development of cochlear implants, numerous designs and strategies have emerged. In the past, the prostheses had either single or multiple electrodes and channels. The electrode array was either long or short (6–30 mm) and could be positioned either in or out of the cochlea. Although there are design differences among devices, the basic implant system consists of an implanted electrode array and receiver-stimulator, and an externally worn microphone, transmitter, and processor. Currently, the most widely used devices are multielectrode/ multichannel and use a straight or precoiled electrode array and transcutaneous transmission. The single most affecting difference, however, is the processing strategy, which ultimately determines the nature of the stimulation of the electrodes. The variable factors include rate of stimulation, simultaneous or sequential stimulation, analog or pulsatile stimulation, fewer or more channels, and variations of waveform or speech feature extraction of the incoming signal.
A review of coding strategies and resultant performance used in early versions of cochlear implants can be found in a variety of publications. The most obvious result of advances in coding methodology can be found in the studies that have compared postoperative performance following speech processor upgrades using updated approaches to signal processing. The investigations showed markedly improved adult subject performance on open-set stimuli when continuous interleaved sampling (CIS) replaced simultaneous analog, when Spectral Peak (SPEAK) supplanted its predecessor MPEAK, when Multi Peak (MPEAK) succeeded its antecedent, and so forth. Those improvements in children that can be attributed to technology are somewhat more difficult to determine. The assessment of speech perception in very young children is particularly difficult because the measures are language based and therefore not appropriate for young congenitally/prelinguistically deaf children. The same group of children who are 4 to 5 years old may still not be linguistically competent to perform a perception task where the language has been standardized on hearing children. Despite the obvious evaluation dilemmas, children of all ages with implants have shown increased speech recognition skills with each successive technological evolution. It is not, then, unreasonable to assume that future alterations in processing regimens would further enhance subject outcome in both adult and child implantees.
Concurrently, research related to electrode design and its ultimate impact on performance is being conducted. The goal of electrode research is to design an array that (1) is the least traumatic to the region; (2) may be sufficiently atraumatic as to preserve residual hearing; (3) can get close to the neural elements to reduce the power needed for stimulation; (4) can stimulate a more selective cell subpopulation; and (5) can deliver lubricants, antiinflammatory agents, and neurotrophic growth factors should they be proven beneficial. Manufacturers have also provided various types of arrays so that patients with a Mondini deformity common cavity and other anatomic deformities can be successfully implanted.
In summary, dynamic technological advancements in implant design and processing not only alter absolute postoperative scores but also influence the interactions and correlations among all of the variables that affect outcome with a cochlear implant.
Surviving Neural Population, Auditory Deprivation, Auditory Pathway Development, Plasticity of the Auditory System, Length of Deafness, and Age at Time of Implantation
The second variable subset involves the physiologic manifestation of ganglion cell survival and the effects of the lack of auditory input to the system over a period of time. Investigators have demonstrated that chronic electrical stimulation in neonatally deafened kittens resulted in the preservation of spiral ganglion cells that, without stimulation, would follow a pattern of continuous neuronal degeneration (see Chapter 3). Although numerous studies have shown that early sound deprivation does result in negative effects on the central auditory system, a finite critical period has not yet been defined; however, deprivation at later times during development does not have the same severe consequences as early deprivation, confirming the existence of an as yet unspecified critical period. Similar neutralizing effects of electrical stimulation have been found on neurons in kittens that were deafened as adults.
Ponton et al (1996) and Sharma et al (2002) provided further support for the detrimental effects of deprivation with concomitant positive effects of the introduction of stimulation, although the effects may be limited (Ponton et al, 1999, 2001). In the Ponton study, evoked cortical responses were obtained from groups of normal hearing and deaf children. Although the rate of development of P1 was similar in the deaf and normal-hearing groups, the development of P1 was delayed by the length of deafness prior to implantation of the deaf children. Latencies of the P2 component reached adult levels at a very young age in the normal-hearing group, but the P2 component did not appear to develop at all in the deaf group despite implantation. On the basis of these results, it is possible to postulate that the auditory pathways do not evolve without stimulation. Nevertheless, maturation of P1 does occur following the introduction of sound. The lack of development of P2, however, might indicate some selective plasticity, confined to certain portions of the pathways. The data from the Sharma et al study, which examined P1 latencies in congenitally deaf children implanted at ages ranging from 1 to 17 years, suggested that there is a period of ˜7years in which the human auditory system is plastic to different degrees. Beyond age 7, the plasticity of the system is reportedly greatly reduced.
We can hypothesize that the clinical correlates of deprivation and plasticity and their consequences are related to length of deafness and age at implantation and their effects on performance with a cochlear implant. Fryauf-Bertschy et al (1997) showed that children who received implants before age 5 performed better on open-set recognition tasks than did those who received implants after 5 years of age. Additionally, the above age 5 group had a greater number of inconsistent implant users than the younger group, presumably due, at least in part, to their reduced benefit. More compelling was the fact that of five children whose length of deafness was at least 10 years, only one child uses the device consistently.
In several studies, Waltzman and colleagues examined the effects of age and length of deafness on implant performance. In the initial study in 1994, results on open-set tests on children who received implants before the age of 3 were found to be better than for results reported by Miyamoto et al (1993) on children whose mean age at implantation was 6.1 years. Although the majority of children in both studies were congenitally deaf, the mean length of deafness for those with acquired prelingual hearing loss was 4.8 years in the Miyamoto study and 1.5 years in Waltzman’s New York University (NYU) study. Another NYU study by Waltzman and Cohen (1998) compared the development of auditory skills in children who received implants before the age of 2 to those who received implants between 3 and 5 years of age. The results suggested that the younger children achieved levels of auditory performance equal to or greater than the older group with comparable duration of usage. In fact, recently it has been shown that children implanted at a young age achieve auditory skills that can allow them to develop oral fluency in several languages (McConkey Robbins et al, 2004b; Waltzman et al, 2003).
Universal newborn hearing screening, however, has enabled the early detection of significant hearing loss worldwide and consequently an interest in implanting children below the age of 2 and most recently below 12 months of age. The rationale for earlier implantation is rooted in the fact that these children have significant delays in oral language skills relative to their normal-hearing peers despite the use of hearing aids and appropriate intervention. Results in these children have been very encouraging. Waltzman and Cohen (1998), Govaerts et al (2002), Kirk et al (2002), Geers et al (2003a,b), McConkey Robbins et al (2004a), Svirsky et al (2004), and Zwolan et al (2004) found that children implanted between the ages of 12 and 24 months attained better speech perception scores and oral language capability than those who received implants above the age of 2. (This chapter is focused on speech perception; for a more in-depth discussion of language development, see Chapter 13.) Although these and other studies too numerous to mention have discussed the relative benefits of implantation below the age of 2, data regarding the safety and efficacy of implantation in children below the age of 12 months are just beginning to emerge. Although James and Papsin (2004) and Waltzman and Roland (2005) reported that implant surgery was shown to be safe in children under 12 months, the authors caution that special attention needs to be paid to issues related to anesthesia, blood loss, flap breakdown, etc. The potential speech perception and linguistic advantages of implantation below 12 months of age versus implantation between 12 and 24 months, however, are still in the early stages of exploration. Lesinski-Schiedat et al (2004) reported on a small number of children implanted under 12 months of age and followed for 2 years and found better performance on the Infant Toddler Meaningful Auditory Integration Scale (IT-MAIS) in this group than for children implanted between 12 and 24 months. Similar results on a slightly larger group of children were described by Waltzman and Roland in the 2005 study. In that report, results on the IT-MAIS for young implanted children followed for 1 year were found to be in the same range as for normal-hearing children. Svirsky (2005), however, in a presentation at the CI2005 pediatric meeting in Dallas, found no difference in language development for the short term in a small number of subjects implanted below 12 months of age versus implantation in the 12-to 24-month age group, although both groups performed better than those implanted above the age of 2. These data are surely preliminary and it remains to be seen whether implantation below 12 months of age is truly more beneficial for oral language development in the long term compared with implantation at 12 to 24 months of age.
With so much focus on younger children, the inference should not be made that later-implanted children do not derive considerable benefit from devices. As technology evolves, outcomes for the congenitally deaf later-implanted group have shown substantial improvement. Dowell et al (2002a,b), Schramm et al (2002), and Waltzman et al (2002b) found significant improvement in open-set speech understanding in congenitally deaf children implanted between the ages of 8 and 18, and similar results have been shown with long-term deafened adults. Because research in the early 1990s did not reveal improvement in auditory performance following implantation in the long-term deafened population, it is not unreasonable to assume a connection between newer processing strategies and improved functioning, nor is it unreasonable to assume that future coding strategies might allow for greater achievements.
The effects of these results on our understanding of the plasticity of the auditory system are as yet unclear but would indicate a more complex interaction between physiologic plasticity and electrical stimulation.
Preoperative Hearing Level and Preoperative Auditory Speech Perception
As Chapter 6 indicated, criteria for implantation have expanded to include those with greater amounts of residual hearing. Several investigators have noted that children with higher (better) hearing levels preoperatively obtain better results postimplantation when compared with children with greater amounts of preoperative hearing loss (Dowell et al, 2004; Gantz et al, 2000; Mondain et al, 2002). Although preoperative hearing levels and speech perception skills appear to contribute to postoperative performance, the effects of technology upgrades and length of usage, which permit a global improvement in scores, may reduce the influence of preoperative open-set speech recognition. More in-depth studies over a protracted period of time are needed to define the contribution of baseline hearing.
Additional Handicaps
Hearing impairment in a child is sometimes one of several sequelae associated with a particular syndrome or etiology, the presence of which can affect the outcome with a cochlear implant. Moreover, a child with no other apparent physical or cognitive issues can have other soft sign disabilities manifest themselves after they start school similar to their normal-hearing peers. For instance, children without hearing loss are often diagnosed with a variety of conditions including learning disabilities or attention deficit disorders only after they have entered elementary school. The same is certainly true of hearing-impaired children. The preoperative implant counseling sessions should alert the family to this possibility and to the adverse effects these and other issues, related to the hearing loss or not, can have on the ability of a child to make use of the signal provided by the implant. Reports on handicapped children using cochlear implants have confirmed that many show substantial improvement in auditory, linguistic, and communication skills following implantation, although the degree of gain is largely based on the extent of the disability not related to the hearing loss (Donaldson et al, 2004; Fukuda et al, 2003; Hamzavi et al, 2000; Knutson et al, 2000; Waltzman et al, 2000). Disabilities include blindness, CHARGE syndrome (coloboma of the eye, heart anomaly, atresia choanae, retardation, and genital and ear anomalies), attention deficit/hyperactivity disorder (ADHD), cerebral palsy, pervasive developmental disorder (PDD), autism, and many others. The parents of children with other issues often want to believe that the presence of the implant will “cure” the child. It is important to gently reduce their expectations without completely discouraging them.
There are two conditions that warrant special comments: deafness from meningitis and auditory neuropathy. The results associated with postmeningitic hearing loss are often dependent on the amount of ossification in the cochlea and the additional sequelae associated with meningitis. If bony growth is in place in the cochlea, a partial insertion of the electrode array or drill-out procedure (see Chapter 10) is not unusual. In these cases, prognosis should be guarded. Moreover, if the child had additional problems resulting from the meningitis, the outcomes can also be compromised (Francis et al, 2004).
Auditory neuropathy/dyssynchrony is a type of hearing impairment in which the outer hair cells are functional but neural transmission is impaired and the hearing loss can vary from near normal to severe. A few of the defining characteristics are the presence/absence of a significant hearing deficit, presence of otoacoustic emissions, but abnormal auditory brainstem response (ABR) recordings. The speech perception ability of these children varies from expected performance levels commensurate with the level of sensorineural hearing loss to little or no speech understanding despite good auditory thresholds. Results with cochlear implants, however, have been promising. Shallop et al (2001) matched a group of children diagnosed with auditory neuropathy to an implanted group with other etiologies and found no difference in benefit between the two groups; similar results are being reported by other clinicians (Peterson et al, 2003; Rance et al, 2002, 2004). Although preliminary, these data suggest that cochlear implantation is a viable treatment option for children diagnosed with auditory neuropathy.
Surgical Issues
The goal of cochlear implant surgery is to insert the entire electrode array into the scala tympani with as little damage as possible to the structure of the inner ear. Various factors can affect the ability of the surgeon to fully insert the electrode array: severe Mondini dysplasia or common cavity bone growth (labyrinthitis ossificans) leading to obstruction within the cochlea, properties of the electrode array itself, and surgical experience. Additional surgical issues include kinking or other surgical trauma to the electrode, facial nerve injury, and postoperative flap problems, including breakdown or infection that might lead to extrusion. For a complete review of surgical issues and complications, see Chapter 9.
Device Programming
A very important influence on performance is the manner in which the speech processor is programmed. Behavioral responses in adult implant recipients were sufficient to obtain accurate electrical threshold and comfort levels for the majority of patients. Although presumed reasonably accurate at the time of programming, thresholds and comfortable loudness levels have been shown to vary over time both in adults and children (Henkin et al, 2003; Lee et al, 2000; Shapiro & Waltzman, 1995; Skinner et al, 1995; Waltzman et al, 1986). These shifts can be indicative of measurement technique, physiologic changes brought about by electrical stimulation, or greater familiarity with the stimulus on the part of the patient. The need for accurate psychophysical measures is simple: If electrical thresholds and comfort levels are incorrect, the access to sound is flawed, leading to both poorer postoperative performance and possible nonuse by the patient. Skinner et al (1997) advocated specific subjective procedures and periodic programming sessions in an attempt to decrease the effects of programming issues on outcome, while over the years numerous investigators have advocated objective techniques including evoked stapedius reflex (ESR) to establish device thresholds. Although this and other objective methods did not supersede behavioral approaches for the adult population, implanting devices in very young children provided a more pressing need to examine the applicability of objective measures in implant programming. Loudness scaling techniques and averaged electrical voltages, in addition to ESR, have been used in an attempt to obtain valid and reliable electrical threshold and comfort levels suitable for use in a processor program. Most recently, neural response telemetry (NRT) in the Nucleus device (Cochlear Corporation, Sydney, Australia) and neural response imaging (NRI) in the Clarion device (Advanced Bionics, Valencia, CA) have been implemented to assist with device programming. These bidirectional systems enable the measurement of electrical action potentials from within the cochlea. Information is gathered about the response of the auditory nerve to both electrical stimulation and the functioning of the prosthesis (see Chapter 8). These techniques are becoming increasingly more popular as the age of implantation of children decreases and as the capabilities of the systems within each device increase in both reliability and efficiency and are being advocated by numerous clinicians. Nevertheless, behavioral programming techniques are still commonly and successfully used to program children of all ages. When used appropriately, both methods employed in tandem can be useful and time saving, especially with multiply-handicapped or difficult-to-test children.
Programming, however, is not simply the determination of thresholds and comfort levels; programming dilemmas often arise. Labyrinthitis ossificans, Mondini deformities, and other inner ear abnormalities often result in a partial insertion of the electrode array, thereby limiting the number of electrodes available for programming. Pseudomonopolar, variable-mode, and double-electrode mappings are some of the special condition strategies used in challenging programming situations. For a complete discussion of behavioral and objective programming techniques, see Chapters 8 and 11.
To a much smaller extent, device reliability plays a role in outcome. Although current implants are reliable and device failures infrequent, there are cases of internal receiver/ stimulator or external equipment failure that remain undetected due to either intermittence or an inability to diagnose the defect. These problems, while discomfiting and distressing, do not usually have a long-term effect on performance. Temporary decreases in speech perception and production may be observed until the equipment is replaced, and recent studies have shown that reimplantation of an electrode array does not have an adverse affect on performance; the same or better results can be achieved following reimplantation (Alexiades et al, 2001; Ray et al, 2004; Waltzman et al, 2004).
Mode of Communication, Auditory Input, Frequency/Type of Training, Educational Setting, and Goals and Expectations
The trend is clear: implanted children obtain speech perception benefit no matter what type of communication mode is used; however, the ability to understand open-set speech stimuli and develop competence in oral language is far greater when oral communication (OC) is the primary mode of communication. Several studies have shown that when oral-only intervention and oral education were employed, children achieved higher levels of speech perception and oral language skills (Geers et al, 2002, 2003a,b; Hodges et al, 1999). It is, however, important to remember that children who use total communication (TC) do benefit from implants and, in fact, many have become oral communicators following implantation. For in-depth information on the effects of mode of communication, see Chapters 15 and 16.
It is unquestionably challenging to assimilate all of these confounding factors and their impact on outcome. However, any prognosis related to postoperative speech perception should include a discussion of the possible effects of all of the variables, including age at implantation, length of deafness, communication mode, and so forth. Although the interactions are not fully known, the preliminary evidence is clear: the wide range of performance reported among implanted children is associated in part with components external to the implant and not solely the implant as an independent entity. This fact becomes crucial during the preoperative counseling of parents regarding expectations. Parents, siblings, and extended family members are central to the process. Much depends on the knowledge they have accumulated prior to surgery and their ability to be flexible as the child’s auditory and linguistic skills begin to emerge. A key factor in this process is the availability of the information necessary for them to make educated decisions. Preoperatively, they need information regarding the available devices so that a choice can be made based on fact rather than hearsay. They need to be apprised of all aspects of implantation, not just device choice, and its ramifications so that they can be certain about the decision to implant. Concurrently, choices need to be made regarding training and educational placement. Flexibility is key: the preoperative auditory and linguistic levels usually dictate the initial communication and school settings, but the course of emerging skills postoperatively often requires a change in the type of training the child is receiving. Depending on the progress, a child who began in a total communication setting may switch to an oral/aural setting or vice versa. It is important that the family not expect immediate or rapid changes in the communication skills of the children. They need to be patient and consistent about follow-up regarding programming and training to maximize the potential for long-term success.
It is hoped that this chapter has inspired contemplation about the complicated ongoing process of pediatric cochlear implantation. The intermeshing of all the variables that might affect outcome is complex, symbiotic, and, to some degree, obscure. But as clinicians and investigators, we would be remiss if we did not attempt to study and clarify the nature of these interactions: undoubtedly, they are dynamic and will change based on technological advancements and expanding criteria.
References
Alexiades G, Roland JT, Fishman AJ, et al. (2001). Cochlear reimplantation: surgical techniques, and functional results. Laryngoscope 111:1608–1613
Donaldson A, Heavner K, Zwolan T. (2004). Measuring progress in children with autism spectrum disorder who have cochlear implants. Arch Otolaryngol Head Neck Surg 130:666–675
Dowell R, Dettman S, Blamey P, Barker E, Clark G. (2002a). Speech perception in children using implants: prediction of long-term outcomes. Cochlear Implants International 3:1–18
Dowell R, Dettman S, Hill K, Winton E, Barker E, Clark G. (2002b). Speech perception outcomes in older children who use multichannel cochlear implants: older is not always poorer. Ann Otol Rhinol Laryngol Suppl 189:97–101
Dowell R, Hollow R, Winton E. (2004). Outcomes for cochlear implant users with significant residual hearing. Arch Otolaryngol Head Neck Surg 130:575–586
Francis H, Pulsifier M, Chinnici J, et al. (2004). Effects of central nervous system residua on cochlear implant results in children deafened by meningitis. Arch Otolaryngol Head Neck Surg 130:604–611
Fryauf-Bertschy H, Tyler RS, Kelsay DM, Gantz BJ, Woodworth GG. (1997). Cochlear implant use by prelingually deafened children: the influences of age at implant and length of device use. J Speech Hear Res 40:183–199
Fukuda S, Fukushima K, Maeda Y, et al. (2003). Language development of a multiply handicapped child after cochlear implantation. Int J Pediatr Otorhinolaryngol 67:627–633
Gantz BJ, Rubinstein JT, Tyler RS, et al. (2000). Long-term results of cochlear implants in children with residual hearing. Ann Otol Laryngol Suppl 2000;185:33–36
Gantz B, Tyler R, Woodworth G. (1994). Results of multichannel cochlear implants in congenital and acquired prelingual deafness in children: five year follow-up. Am J Otol 15:1–7
Geers A, Brenner C, Davidson L. (2003a). Factors associated with development of speech perception skills in children implanted by age 5. Ear Hear 24:24S–35S
Geers A, Brenner C, Nicholas J, Uchanski R, Tye-Murray N, Tobey E. (2002). Rehabilitation factors contributing to implant benefit in children. Ann Otol Rhinol Laryngol Suppl 189:127–130
Geers A, Nicholas J, Sedey A. (2003b). Language skills of children with early cochlear implantation. Ear Hear 24:46S–58S
Govaerts P, De Beukelaer C, Daemers K, et al. (2002). Outcome of cochlear implantation at different ages from 0 to 6 years. Otol Neurotol 23:885–890
Haensel J, Engelke J, Ottenjann W, Westhofen M. (2005). Long-term results of cochlear implantation in children. Otolaryngol Head Neck Surg 132:456–458
Hamzavi J, Baumgartner WD, Egelierier B, Franz P, Schenk B, Gstoettner W. (2000). Follow-up of cochlear implanted handicapped children. Int J Pediatr Otorhinolaryngol 56:169–174
Henkin Y, Kaplan-Neeman R, Muchnik C, Kronenberg J, Hildesheimer M. (2003). Changes over time in electrical stimulation levels and electrode impedance values in children using the Nucleus 24M cochlear implant. Int J Pediatr Otorhinolaryngol 67:873–880
Hodges AV, Dolan Ash M, Balkany TJ, Schloffman JJ, Butts SL. (1999). Speech perception results in children with cochlear implants: contributing factors. Otolaryngol Head Neck Surg 12:31–34
James A, Papsin B. (2004). Cochlear implant surgery at 12 months of age or younger. Laryngoscope 114:2191–2195
Kirk KL, Miyamoto RT, Lento CL, Ying EE, O’Neill T, Fears B. (2002). Effects of age at implantation in young children. Ann Otol Rhinol Laryngol Suppl 189:69–73
Knutson JF, Ehlers SL, Wald RL, Tyler RS. (2000). Psychological predictors of pediatric cochlear implant use and benefit. Ann Otol Rhinol Laryngol Suppl 185:100–103
Lee M, Kim S, Huh M, Kim L. (2000). Changes in electrical thresholds and dynamic range over time in children with cochlear implants. Adv Otorhinolaryngol 57:339–342
Lesinski-Schiedat A, Illg A, Heermann R, Bertram B, Lenarz T. (2004). Paediatric cochlear implantation in the first and second year of life: a comparative study. Cochlear Implants Int 5:146–159
McConkey Robbins A, Green JE, Waltzman SB. (2004a). Bilingual oral language proficiency in children with cochlear implants. Arch Otolaryngol Head Neck Surg 130:644–647
McConkey Robbins A, Koch D, Osberger M, Zimmerman-Phillips S, Kishon-Rabin L. (2004b). Effect of age at implantation on auditory skill development in infants and toddlers. Arch Otolaryngol Head Neck Surg 130:570–574
Meyer TA, Svirsky MA, Kirk KI, Miyamoto RT. (1998). Improvements in speech perception by children with profound prelingual hearing loss: effects of device, communication mode and chronological age. J Speech Lang Hear Res 41:846–858
Miyamoto R, Osberger M, Robbins A, Myres W, Kessler K. (1993). Prelingually deafened children’s performance with the Nucleus multichannel cochlear implant. Am J Otol 14:437–445
Mondain M, Sillon M, Vieu A, et al. (2002). Cochlear implantation in prelingually deafened children with residual hearing. Int J Pediatr Otorhinolaryngol 63:91–97
Osberger M, Miyamoto R, Zimmerman-Phillips S, et al. (1991). Independent evaluation of the speech perception abilities of children with the Nucleus 22 channel cochlear implant system. Ear Hear 12:66S–80S
Peterson A, Shallop J, Driscoll C, et al. (2003). Outcomes of cochlear implantation in children with auditory neuropathy. J Am Acad Audiol 14:188–201
Ponton CW, Eggermont JJ. (2001). Of kittens and kids: altered cortical maturation following profound deafness and cochlear implant use. Audiol Neurootol 6:363–380
Ponton C, Eggermont J, Waring M, Kwong B, Masuda A. (1996). Auditory system plasticity in children after long periods of complete deafness. Neuroreport 8:61–65
Ponton CW, Moore JK, Eggermont JJ. (1999). Prolonged deafness limits auditory system developmental plasticity: evidence from an evoked potentials study in children with cochlear implants. Scand Audiol Suppl 51:13–22
Rance G, Cone-Wesson B, Wunderlich J, Dowell R. (2002). Speech perception and cortical event related potentials in children with auditory neuropathy. Ear Hear 23:239–253
Rance G, McCay C, Grayden D. (2004). Perceptual characterization of children with auditory neuropathy. Ear Hear 25:34–46
Ray J, Proops D, Donaldson I, Fielden C, Cooper H. (2004). Explantation and reimplantation of cochlear implants. Cochlear Implants Int 5:160–167
Schramm D, Fitzpatrick E, Seguin C. (2002). Cochlear implantation for adolescents and adults with prelinguistic deafness. Otol Neurotol 23:698–703
Shallop JK, Peterson A, Facer GW, Fabry LB, Driscoll CL. (2001). Cochlear implants in five cases of auditory neuropathy: postoperative findings and progress. Laryngoscope 111:555–562
Shapiro W, Waltzman S. (1995). Changes in electrical thresholds over time in young children implanted with the Nucleus cochlear prosthesis. Ann Otol Rhinol Laryngol Suppl 166:177–178
Sharma A, Dorman M, Spahr A. (2002). A sensitive period for the development of the central auditory system in children with cochlear implants: implications for age of implantation. Ear Hear 23:532–539
Skinner M, Holden L, Demorest M, Holden T. (1995). Use of test-retest measures to evaluate performance stability in adult cochlear implant users. Ear Hear 16:187–197
Skinner M, Holden L, Holden T. (1997). Parameter selection to optimize speech recognition with the Nucleus implant. Otolaryngol Head Neck Surg 117:188–195
Staller S, Dowell R, Beiter A, Brimacombe J. (1991). Perceptual abilities with the Nucleus 22 channel cochlear implant system. Ear Hear 12:34S–47S
Svirsky M, Holt R. (2005). Speech perception and language in congenitally deaf children implanted in the first year of life. Paper presented at the Tenth Symposium on Cochlear Implants in Children, March 2005, Dallas, Tx
Svirsky M, Teoh S, Neuberger H. (2004). Development of language and speech perception in congenitally, profoundly deaf children as a function of age at implantation. Audiol Neurootol 9:224–233
Waltzman S, Cohen N. (1998). Cochlear implantation in children younger than 2 years of age. Am J Otol 19:158–162
Waltzman S, Cohen N, Gomolin R, et al. (1997). Open-set speech perception in congenitally deaf children using cochlear implants. Am J Otol 18:342–349
Waltzman S, Cohen N, Green J, Roland JT. (2002a). Long-term effects of cochlear implants in children. Otolaryngol Head Neck Surg 126:505–511
Waltzman S, Cohen N, Shapiro W. (1986). Long-term effects of multichannel cochlear implant usage. Laryngoscope 96:1083–1087
Waltzman SB, Cohen NL, Shapiro WS. (2004). Use of a multichannel cochlear implant in the congenitally and prelingually deaf population. Laryngoscope 102:395–399
Waltzman S, Robbins AM, Green J, Cohen N. (2003). Second oral language capabilities in children with cochlear implants. Otol Neurotol 24: 757–763
Waltzman S, Roland JT, Cohen N. (2002b). Delayed implantation in congenitally deaf children and adults. Otol Neurotol 2002;23:333–340
Waltzman SB, Roland JT. (2005). Cochlear implantation in children younger than 12 months. Pediatrics 116:e487–e493
Waltzman S, Roland JT, Waltzman M, Shapiro W, Lalwani A, Cohen N. (2004). Cochlear reimplantation in children: soft sign, symptoms and results. Cochlear Implants International 5:138–145
Waltzman S, Scalchunes V, Cohen N. (2000). Performance of multiply handicapped children using cochlear implants. Am J Otol 21:329–335
Zwolan T, Ashbaugh C, Alarfaj A, et al. (2004). Pediatric cochlear implant patient performance as a function of age at implantation. Otol Neurotol 25:112–120
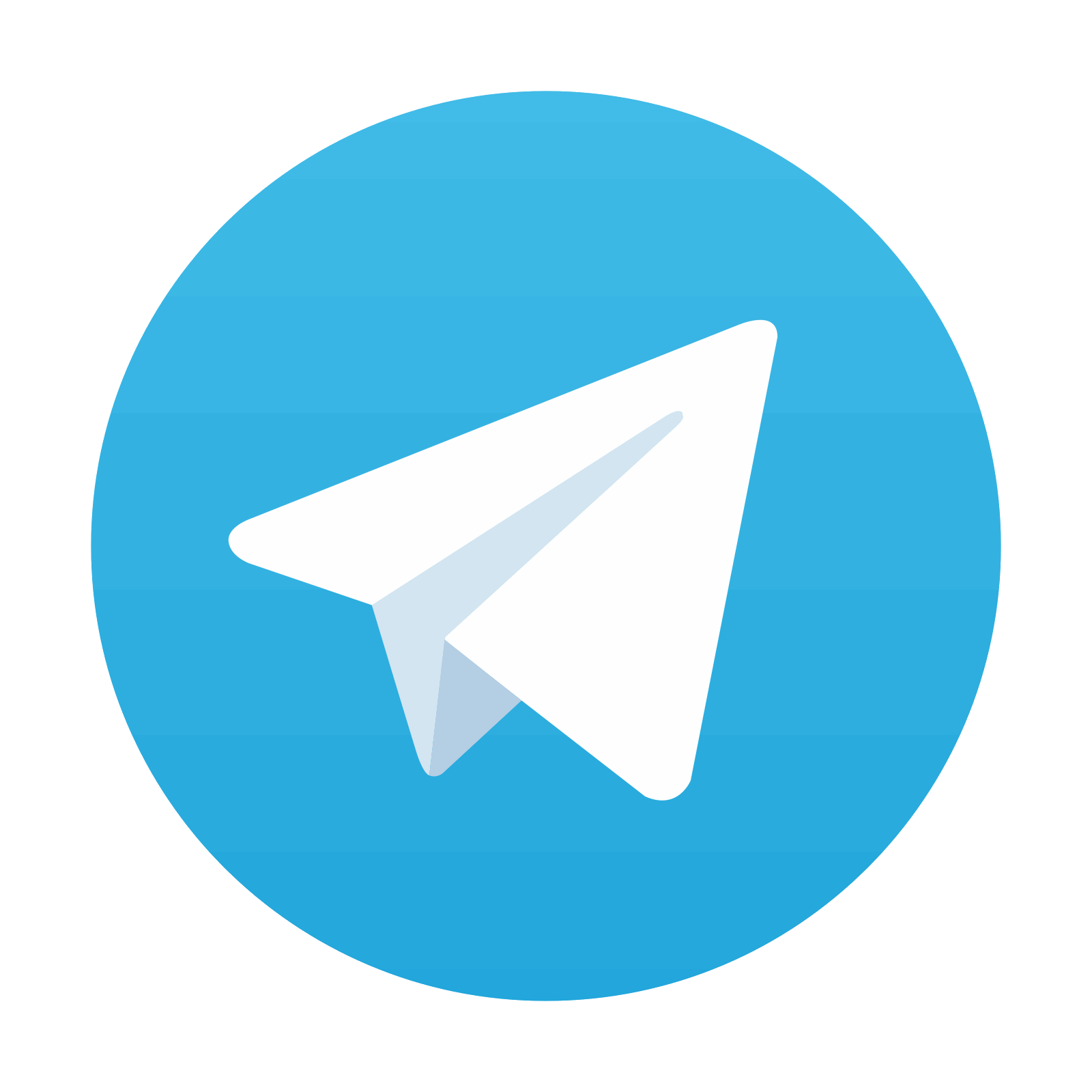
Stay updated, free articles. Join our Telegram channel

Full access? Get Clinical Tree
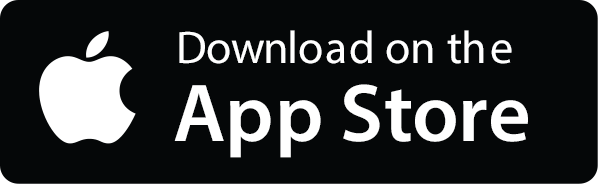
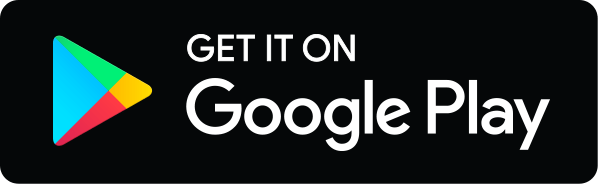