Fig. 26.1
Schematic representation of the developing SGNs in mice, modified Coate and Kelley [3]. (a) Neuroblasts delaminate from the otocyst and form the CVG. (b) The last wave of neuroblasts populates the SGNs. (c) SGNs coil along the lengthening cochlear duct. Type II SGNs extend into the OHC region at the base. CVG cochleovestibular ganglion, SGNs spiral ganglion neurons, CN cochlear nucleus, CE cochlear epithelium
26.2 Neurogenesis
Neurogenin1 (Neurog1) and Neurod1 are members of the basic helix-loop-helix (bHLH) family of transcription factors. Neurog1 is necessary for SGN formation; deletion of Neurog1 in mouse causes complete loss of SGNs [2]. Neurod1 is necessary for SGN differentiation and survival; in mice that lack Neurod1, neurons are lost just after delamination and have completely disappeared by E13.5 [6]. Although either Neurog1 and Neurod1 is sufficient to induce neuronal identity in cochlear non-sensory epithelial cells at embryonic stages in vitro, ectopic expression of Neurog1 fails to induce Neurod1 expression. Therefore, Neurog1 and Neurod1 might function in different signaling cascades or Neurog1 requires specific cofactors to induce Neurod1 expression [7].
bHLH transcription factors such as Neurog1 and Neurod1 interact with LIM-HD proteins to synergistically regulate gene expression [8, 9]. Specifically, Islet1 is an LIM-HD gene that plays a role in development and differentiation of the proneurosensory domain at E12 in mouse. As the sensory epithelium starts to differentiate, Islet1 is downregulated in the entire cochlear epithelium but is maintained in cells following a neuronal lineage. Therefore, Islet1 is an early marker for the proneurosensory domain and a later marker for SGNs and plays a potential role in the inner ear-specific sensory and neuronal cell development [10]. The expression of these proneural factors such as Neurog1, Neurod1, and Islet1 is maintained by the ATP-dependent chromatin remodeling enzyme CHD7, which is mutated in human CHARGE syndrome [11].
Sox2 is not only a universal stem cell marker but also one of the earliest definitive markers of the proneurosensory domain within the developing inner ear [12]. Sox2 plays an important role in maintaining a pool of multipotent progenitors and controlling differentiations of neurons and sensory epithelial cells [7, 12, 13]. Sox2 is downregulated in nascent hair cells by E16 in mouse, but remains expressed in supporting cells and spiral ganglion cells [7, 13]. Mutations in SOX2 lead to sensorineural hearing loss in humans [14]. Light coat and circling (Lcc) mutant mice that do not express Sox2 in the inner ear exhibit a loss of neurons [7] and a loss of hair cells and supporting cells [12], suggesting Sox2 is necessary for SGN and sensory cell formation. Sox2 is also sufficient to induce neurons in non-sensory regions of the cochlea at embryonic stages [7].
The murine eyes absent (Eya) and homeobox Six gene family are essential for inner ear development, which is arrested at the otocyst stage in either Eya1- or Six1-null mutants [15–17]. Haploinsufficiency for human EYA1 and SIX1 causes branchio-oto-renal syndrome [18, 19]. Specifically, in Eya1- or Six1-null mutants, neurogenesis is initiated, but neuroblasts do not form the CVG, suggesting both genes are required for differentiation and maintenance of sensory neurons [20]. More recently, it was shown that Eya1 and Six1 together with the SWI/SNF ATP-dependent chromatin remodeling protein complex activate Neurog1 and Neurod1, and Sox2 cooperates with these factors to mediate neuronal differentiation [21].
26.3 A Potential Role of Insulin-Like Growth Factor-1 (IGF-1) in SGN Survival and Maturation
IGF-1 plays an important role in murine inner ear morphogenesis [22] and postnatal cochlear maturation [23]. Igf1r is expressed in SGNs at E13.5 and E16.5 demonstrated by in situ hybridization [22]. Igf1r is also predominantly expressed in SGNs compared to VGNs both at embryonic and postnatal stages according to Goodrich’s lab microarray database [24]. The number of SGNs in Igf1−/− mutants decreases as a result of apoptotic cell death beginning after P20 [23], implying that IGF-1 plays a role in SGN survival and maturation. However, SGN loss in Igf1−/− mutants might be secondary due to insufficient production of neurotrophins by cochlear hair cells. Additionally, the number of SGNs in Igf1r−/− mutants at embryonic stages does not appear to be different from that of heterozygotes (Takayuki Okano, personal communication). Conditional deletion of Igf1r using an inner ear-specific driver is necessary to elucidate a potential role of IGF-1 in SGN survival and maturation, because Igf1r−/− mutants die at birth, preventing a subsequent analysis of inner ear [22].
26.4 Spiral vs. Vestibular Ganglion Neuron Specification
SGNs and VGNs are generated during two overlapping but distinct waves of neurogenesis; SGNs derived from a Neurog1 lineage are born later than VGNs [1], in line with the previous birth-dating studies that VGNs are born before SGNs [25]. Additionally, neuroblasts giving rise to SGNs and VGNs delaminate from distinct regions of the otocyst [26]. The zinc finger transcription factor Gata3 is initially expressed at the otocyst, but its expression is progressively restricted to SGNs and the organ of Corti compared to VGNs [26, 27]. Haploinsufficiency of GATA3 causes human hypoparathyroidism, sensorineural deafness, and renal dysphasia (HDR) [28, 29], indicating GATA3 has a profound role in cochlear neurosensory development. Conditional deletion of Gata3 in SGNs leads to precocious extension of their peripheral axons and aberrant path finding to cochlear epithelium [27]. Expression profiling of Gata3-null SGNs revealed a broad shift in gene expression toward a more differentiated state, implying Gata3 serves as an intermediate regulator that guides SGNs through differentiation and preserves the auditory fate [27]. At present, upstream factors that restrict Gata3 to the auditory division in the CVG remain to be seen.
26.5 Type I and Type II Spiral Ganglion Fate Specification
The type I neurons, which comprise 90–95 % of the SGNs, terminate on inner hair cells. Each afferent dendrite contacts only a single inner hair cell, but each hair cell directs its output to multiple nerve fibers [30]. Type II neurons comprise 5–10 % of the SGNs and a single type II neuron contacts 10–100 different outer hair cells [31, 32]. Type I and type II SGNs are different even at embryonic stages demonstrated by the following observations: a small number of type II SGNs in the base of the cochlea at E16.5 extend past the row of inner hair cells and innervate directly to outer hair cells [1] (Fig. 26.1). A subset of SGNs at E18.5 is labeled peripherin [33], which marks type II SGNs in the adult [34]. On the other hand, SGNs develop through a process of superfluous outgrowth of afferent dendrites followed by pruning at postnatal stages [35]. Therefore, although type I and type II SGNs are specified at embryonic stages, refinement of projections occurs during later stages of circuit assembly.
26.6 Tonotopy in the SGNs
In SGNs, there is tonotopic distribution of firing features, voltage-gated ion channels, and synaptic proteins: SGNs isolated from apical, low-frequency regions showed greater levels of slow accommodation compared to those isolated from basal, high-frequency regions [36]; large conductance calcium-activated potassium channels, Kv1.1 and Kv3.2, are enriched in basal neurons, contributing to abbreviated firing features [36]; the presynaptic protein synaptophysin is enriched in the apical neurons while the postsynaptic AMPA receptor subunits GluR2 and GluR3 are abundant in the basal neurons [37]. Neurotrophin-3 (Ntf3) is expressed preferentially in the apical SGNs, whereas brain-derived neurotrophic factor (Bdnf) is expressed predominantly in the basal SGNs [38]. Regionalized concentrations of NTF3 and BDNF partly determine tonotopic features that characterize SGNs in the fully developed ear [39]. Characteristics of basal SGNs is enhanced by exposure to BDNF and reduced by NTF3, whereas that of apical SGNs is enhanced by NTF3 and reduced by BDNF [39]. “Yin-yang” regulatory effects of Ntf3 and Bdnf on voltage-gated ion channels and synaptic protein composition in SGNs indicate that Bdnf and Ntf3 activate distinctly different elements of trk-based signaling pathways [39].
References
1.
Koundakjian EJ, Appler JL, Goodrich LV. Auditory neurons make stereotyped wiring decisions before maturation of their targets. J Neurosci. 2007;27(51):14078–88. doi:10.1523/JNEUROSCI.3765-07.2007.PubMedCrossRef
2.
Ma Q, Chen Z, del Barco Barrantes I, de la Pompa JL, Anderson DJ. Neurogenin1 is essential for the determination of neuronal precursors for proximal cranial sensory ganglia. Neuron. 1998;20(3):469–82.
3.
Coate TM, Kelley MW. Making connections in the inner ear: recent insights into the development of spiral ganglion neurons and their connectivity with sensory hair cells. Semin Cell Dev Biol. 2013;24(5):460–9. doi:10.1016/j.semcdb.2013.04.003.PubMedCentralPubMedCrossRef
4.
Carney PR, Silver J. Studies on cell migration and axon guidance in the developing distal auditory system of the mouse. J Comp Neurol. 1983;215(4):359–69. doi:10.1002/cne.902150402.PubMedCrossRef
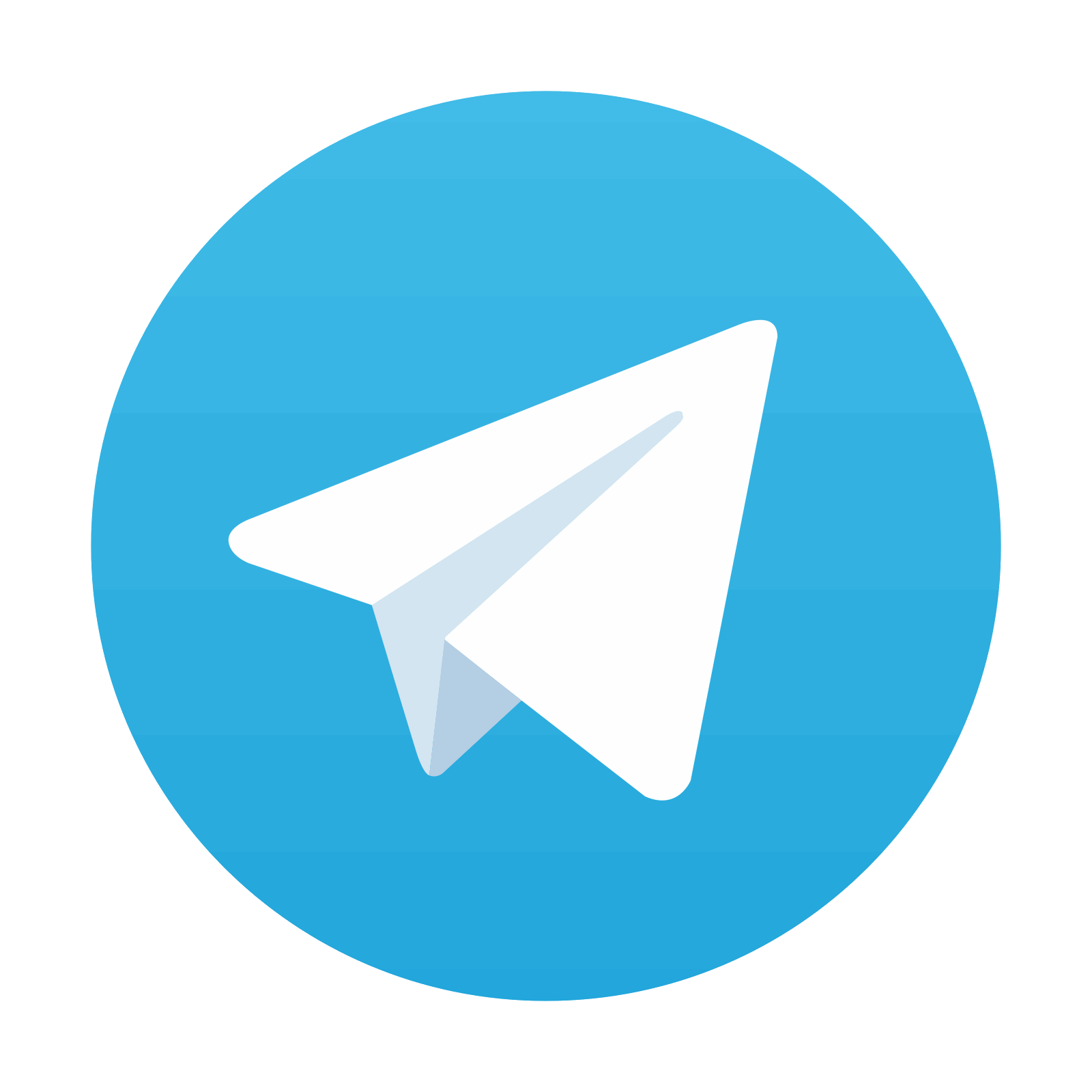
Stay updated, free articles. Join our Telegram channel

Full access? Get Clinical Tree
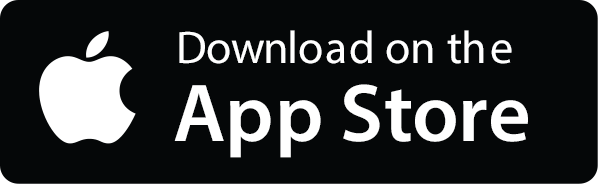
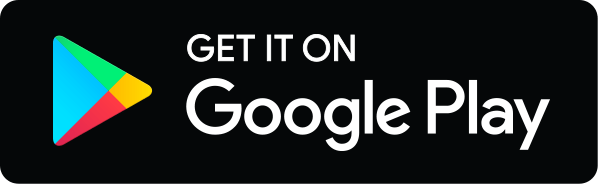