Retinoscopy
Jonathan D. Wirtschafter
Gary S. Schwartz
Retinoscopy is the most practical, manual method of objective refraction. Classic textbooks of ophthalmology1 and visual optics2,3 have stressed that the practice of retinoscopy4,5 follows easily from an understanding of the optics of the retinoscope, whereas monographs on practical retinoscopy concentrate on the technique in simplified ways that may offend the textbook authors but still represent a level of sophistication beyond the observed behavior of most practitioners. Most practitioners relegate retinoscopy to others, and if asked about it, would merely restate what they remember about neutralization: “With reflex, add plus; against reflex, add minus.” The goal of this chapter is to increase the usefulness of the retinoscope to the practitioner by encouraging the use of ophthalmoscopic retinoscopy while reviewing the basis for the more commonly used neutralization retinoscopy.
The goal of objective refraction is to locate the far point of the spherical eye or the two far-point planes of an astigmatic one. Although the retinoscope offers more than two techniques with which to locate the far point, the most important are ophthalmoscopic retinoscopy and neutralization retinoscopy. Ophthalmoscopic retinoscopy locates the far point in space by varying the illumination system and observing the image of the retinoscope filament on the retina. Neutralization retinoscopy is a technique that brings the patient’s far point to a location that is a predetermined distance from the patient, the working distance, usually 66 cm (1.50 diopters [D]) in front of the eye, through the use of lenses. Neutralization retinoscopy has a well-defined and rather accurate end point, and, in contrast to ophthalmoscopic retinoscopy, varies the observation system to observe the image from the illuminated retina. Although ophthalmoscopic retinoscopy is faster than neutralization retinoscopy, use of the two techniques in conjunction often requires less time than neutralization alone, especially in difficult cases. There is simply no reason to spend more than 5 minutes neutralizing an eye when ophthalmoscopic retinoscopy can quickly reveal the source of the difficulty.
Ophthalmoscopic retinoscopy is often referred to as “estimation,” and, in fact, works well to estimate a patient’s refractive error. However, certain modifications of the neutralization retinoscopy technique can also enable the retinoscopist to estimate the patient’s refractive error. These techniques depend primarily on magnification and enhancement of the observed image, and to a lesser extent on observation of the “with and against” or “scissors” reflexes. Our hope is that in studying this chapter, the reader will enhance his or her skills in using the retinoscope, thus increasing both speed and accuracy.
We would like this chapter to be read at two levels. First, we hope it will be useful for the clinician merely hoping to improve his or her clinical skills in using the retinoscope, and in this regard, this chapter should serve as a how-to guide. Second, we would like this text to serve as an initial reference for those wishing to understand the optics and retinoscope in greater detail. In this respect, we have provided information on topics that go beyond the minimum information required for performing ophthalmoscopic and neutralization retinoscopy.
HISTORY OF THE RETINOSCOPE
The observations that led to clinical retinoscopy were made in 1859 with a plane mirror ophthalmoscope lighted by a candle, when Sir William Bowman noted a linear shadow seen when examining an astigmatic eye. By 1875, the optics were explained and the procedure was described as a “shadow test,” an allusion to neutralization. H. Parent coined the term retinoscopy in 1881, but the term is somewhat of a misnomer because the technique actually provides an ophthalmoscopic view of the light reflected from a patch of retina. The earliest retinoscopes used a mirror to reflect a candle, which produced a “spot” of light. It was soon discovered that a linear streak of light could be produced with slit-shaped mirrors. Early electric retinoscopes used spiral filament bulbs and a rotating slit. Jacob Copeland introduced a linear filament bulb that produced a sharp, bright line of light.4,6,7,8 The Copeland streak retinoscope set the standard for future retinoscopic developments.9,10 As with any instrument, many advances have been incorporated over the years, and innovations continue to this day, incrementally improving the viewing system, control of vergence and filament meridians, handle and sleeve design, and battery power.
OPTICS OF THE RETINOSCOPE
The streak retinoscope has supplanted the spot retinoscope in the modern eye clinic, and only the streak retinoscope is discussed in this chapter. Although the various brands of streak retinoscopes differ in design, they all work similarly. Light is produced by a luminous filament within the base of the handle and emanates from a mirror in the head as a linear streak, with both orientation and vergence controlled by the retinoscopist. The streak of light passes through the patient’s tear film, cornea, anterior chamber, lens, vitreous chamber, and retina. It is then reflected from the choroid and retinal pigment epithelium as a linear red reflex that passes back through the sensory retina, vitreous, lens, aqueous, cornea, and tear film, through the air between the patient and the examiner, and into the head of the retinoscope, through an aperture in the mirror, finally exiting through the back of the retinoscope into the retinoscopist’s own eye. By observing qualities of the reflected light (the reflex) after it leaves the patient’s eye, the retinoscopist can make determinations about the patient’s refractive state.
Explaining the optics and proper usage of the retinoscope can be a confusing business. To help simplify the text, we have chosen to use the feminine pronouns (e.g., “she” and “her”) when referring to the retinoscopist, and the masculine ones (e.g., “he,” “him,” and “his”) when referring to the patient.
All streak retinoscopes are made of the same fundamental components: light source, condensing lens, mirror, and sleeve (Fig. 1). The light source is a light bulb with a fine, linear filament, which projects a fine, linear streak of light with the passage of an electric current. The filament (which should be considered a linear point source of light), and therefore the streak, can be rotated 360 degrees by rotating the sleeve of the retinoscope. Currently, most retinoscopes use a halogen bulb, which projects a very bright streak. The condensing lens is a plus lens, which exerts positive vergence on the streak, which is emitted from the point-source filament in a highly diverging manner. The position of the lens in relation to the light filament can be altered by raising or lowering the sleeve. In this way, the vergence of the streak that is emitted from the retinoscope can be controlled by the retinoscopist, as described subsequently.
The mirror bends light that originates in the handle and is initially projected upward toward the ceiling, to instead exit the retinoscope along an axis parallel to the floor so that it can be projected into the patient’s eye. The mirror should not reflect 100% of visible light; rather, it must allow some light to pass through it. Only in this way can the retinoscopist have a view into the patient’s pupil that is coaxial to the path of the streak.
OPTICS OF THE PEEPHOLE
The true peephole of the retinoscope is not the hole on the examiner’s side of the instrument; rather, it is a “hole” in the reflecting mirror. The peephole may be manufactured in two basic ways. One is to leave a round area of the mirror unsilvered, whereas the other is to partially silver the mirror so that it acts as a beam splitter. The size of the hole is also a consideration in the design of the instrument. A large hole decreases the useful light reflected into the subject’s eye. At the expense of internal reflections, the beam-splitter helps provide light needed by the retinoscopist to find the subject’s pupil and to maintain a coaxial position. To decrease glare and polarization, some retinoscopes introduce filters between the true peephole and the back of the retinoscope peephole.
The peephole allows the retinoscopist’s view into the patient’s eye to be coaxial to light emitted from the retinoscope. If this coaxial relationship between the path of the retinoscopist’s line of sight and the emitted streak is not established, or she will not see a red-reflex image of the streak. Instead, only the patient’s black pupil will be seen, and the retinoscopist cannot obtain any information regarding the patient’s refractive status. Tilting the retinoscope allows some areas of the retinal reflex to be seen through the peephole while other portions are cut off and are perceived as a dark shadow in the subject’s pupil.
CONTROLLING THE PROPERTIES OF THE RETINOSCOPE
The basic idea behind the retinoscope is that the retinoscopist creates a streak of light, projects it into a patient’s eye, bounces it off his retina, and makes deductions concerning the patient’s refractive status based on what the image of that streak looks like when it reaches the retinoscopist’s eye. To aid her in this task, the retinoscopist has control over, and can easily vary, certain aspects of the system. Two things she can control have nothing to do with the intrinsic properties of the retinoscope she is holding—the distance between the retinoscopist’s eye and the patient’s, and which lenses she may be holding between the patient’s eye and her own; thus, these are not discussed in this section.
However, two properties over which the retinoscopist has total control are completely intrinsic to the retinoscope she is holding. The first is the orientation of the streak as it leaves the retinoscope. Because the light source for the retinoscope is a fine filament, the light emanates from the retinoscope as a fine streak. By rotating the light source, the retinoscopist can easily alter the orientation of the streak by more than 360 degrees. Merely by rotating the sleeve on the handle of the retinoscope, she can project a streak whose orientation is parallel to the floor, or perpendicular to it, or any meridian in between. This feature of the retinoscope proves invaluable when examining patients with astigmatism.
The second property that can be controlled easily by the retinoscopist is the vergence of the incident streak. With the touch of a finger (or thumb), the retinoscopist can alter the streak so that it leaves the retinoscope as converging, diverging, or even parallel light. This feature gives the retinoscopist an incredible amount of power in evaluating a patient’s refractive state. Unfortunately, it is probably the most underused feature of the retinoscope. The average retinoscopist uses only diverging light (plane mirror) when performing an objective refraction, and therefore limits what she can truly accomplish with the apparatus.
Changing the distance between the light filament and the condensing lens alters the vergence of the emitted streak. This can be accomplished by raising or lowering the sleeve in the handle of the retinoscope. This is the most fundamental way in which different models of retinoscope will contrast, and it is obviously important for the retinoscopist to be familiar with the type of retinoscope with which she is working. In the earliest models of retinoscopes (e.g., Bausch and Lomb Copeland [1928] and Copeland-Optec 360 [1958]), the condensing lens is fixed, and the light source can be raised or lowered by moving the sleeve up or down (Fig. 2). When the sleeve is raised in these retinoscopes, the streak emanates as a diverging beam; when the sleeve is lowered, the streak emanates in a converging nature. Most current textbooks on retinoscopy techniques use this type of retinoscope in their discussions4,5 and therefore use the term “sleeve up” when the retinoscope emits diverging light and “sleeve down” when it emits converging light.
In the U.S. market, retinoscopes that emit a converging beam with the sleeve down have largely been supplanted with retinoscopes in which the light source is fixed and the condensing lens can be raised or lowered by moving the sleeve up or down (Fig. 3). Examples of this type of retinoscope include those made by Welch Allyn, Heine, Neitz, and Keeler. In these retinoscopes, when the sleeve is raised, the streak emanates as a converging beam, and when the sleeve is lowered, the streak emanates as a diverging beam. These retinoscopes have a more complex linkage than the retinoscope described previously because the sleeve has two different control functions: to raise or lower the lens and to rotate the bulb filament.
The Copeland streak retinoscope traditionally has been the standard against which others have been compared. However, our informal survey of optical instrument distribution reveals that retinoscopes that converge with the sleeve down are being used in fewer and fewer ophthalmology offices because more clinicians prefer the Welch Allyn, Heine, Neitz, or Keeler models. The reasons for this are a mix of technical (i.e., compatibility of instrument design with rechargeable battery handles) and marketing aspects. Regardless, the market currently is dominated by retinoscopes that converge when the sleeve is up. Therefore, this chapter takes a departure from prior retinoscopy textbooks and use the nomenclature sleeve down to mean that the streak is emitted as diverging light and sleeve up to mean that the streak is emitted as converging light. The reader must be familiar with her own retinoscope. When using a Bausch and Lomb Copeland, Copeland-Optec 360, or other type with a movable light source and fixed condensing lens, she must be aware that the sleeve position should be in the down position when we use the term sleeve up and should be in the up position when we use the term sleeve down.
By altering the vergence of the emitted streak, the retinoscopist is actually manipulating its focal point, the point where the emitted light comes to focus in a point in real or virtual space. When in maximum convergence (sleeve up), that focal point is a real image located 33 cm in front of the retinoscope. (You can test this by moving the palm of your hand in front of the retinoscope at a distance of 33 cm, then turning it on with the sleeve raised all the way up. You should see the image of the filament in perfect focus on the palm of your hand.) As the sleeve is lowered, the focal point moves farther from the retinoscope (aim the retinoscope at a wall and recede) until a point where the sleeve is roughly two thirds of the way down, when the streak is emitted as parallel light and the focal point is at infinity. If the retinoscopist continues to lower the sleeve of the retinoscope, the focal point is created in virtual space far behind her. As she lowers the sleeve more, the focal point moves closer to her until the sleeve is lowered all the way, and the focal point is 40 cm behind her. At no time can the focal point of the streak emanating from the retinoscope be located between 33 cm in front of and 40 cm behind the retinoscope. In other words, the retinoscope cannot project the streak to the region in space that the retinoscope itself occupies. Thus, the retinoscopist must know the location of the converging and diverging focal points of her retinoscope to use it to its full potential.
DETERMINING THE VERGENCE OF THE RETINOSCOPE BEAM
To determine the vergence of a retinoscope at any sleeve adjustment, a simple trick called Foucault’s Method (Fig. 4) can be used. The most instructive part of this exercise is shown in Figure 4A. Note that when a card is introduced at the edge of a converging beam, an opposite movement is produced on a screen located beyond the focal point.
CALIBRATING THE RETINOSCOPE SLEEVE
Perform calibration in a semidarkened examining room with a 20-foot distance from the phoropter to the distant wall. Turn on the retinoscope.
Calibration of the Converging Beam
Bring the sleeve all the way up and place it against a reflecting surface such as the wall. Move away from the wall and observe from the side (not through the peephole) until the streak is in sharp focus on the wall. You should note that when the retinoscope is moved beyond that distance, the streak will go out of focus because the filament light has converged and then diverged (see Fig. 4A). Return to the point of sharp focus and measure to determine the focal point of the retinoscope: it should be about 33 cm, which corresponds to + 3.00 D.
Calibration of the Parallel Beam
Sit in the patient’s examination chair and aim the retinoscope toward the distant wall while moving the sleeve up and down. Watch where the finest focused image of the filament is observed. Note the relative position of the bottom of the sleeve with regard to the range of sleeve movement. In that position, the retinoscope beam is as parallel as possible and it has no vergence and thus is focused at infinity. The Heine retinoscope has a mechanical stop (Para Stop) at the parallel beam position that can be engaged to prevent the vergence control from being adjusted to a convergent beam. This position can be used as the plano calibration.
Calibration of the Diverging Beam
Sit in the patient’s examination chair and place the retinoscope immediately adjacent to the patient’s side of the phoropter. Aim the retinoscope through the phoropter eyehole and onto the distant wall. Do not look through the retinoscope or the phoropter. Move the sleeve all the way down and select the trial lens that allows for the sharpest focus. The divergent beam of the retinoscope will be brought to convergence at infinity when you neutralize it with some lens between + 1.50 to + 2.25 D. Different models of retinoscope vary as to where in space light can be focused behind them. For example, the Heine retinoscope focuses light to 44 cm behind it (-2.25 D), the Welch Allyn focuses light 50 cm behind it (-2.00 D), and the Copeland-Optek cannot focus light to any closer than 66 cm behind it (-1.50 D).
Although the retinoscopist should be aware of the convergence and divergence focal points of the retinoscope that she uses, in truth, very few retinoscopists actually go through the small amount of trouble to measure them.
You have now calibrated the sleeve of your retinoscope and determined that it has an ophthalmoscopic retinoscopy focusing range of 4.00 D of hyperopia to 2.25 D (or 2.00 D or 1.50 D, depending on the model) of myopia. You can record these measurements on a label affixed to the side of the retinoscope (Fig. 5) or simply remember them because you are using the scale only for the estimate. The most useful aspect of the label is the position of plano.
OPHTHALMOSCOPIC RETINOSCOPY
Copeland taught methods for refracting an individual with a retinoscope that relied on neither lenses nor verbal responses from the patient. His technique relied on the recognition of a focused “ophthalmoscopic” image of the retinoscope. Although Dr Copeland’s skills at ophthalmoscopic retinoscopy were such that he could write a spectacle prescription based on information garnered from this technique alone, most of us use it only as a technique of estimating the refractive error, and few of us feel comfortable enough with our skills to rely solely on this method. However, ophthalmoscopic retinoscopy is a valuable technique to use as a prelude to neutralization, especially in a patient with an unknown refraction. It is quick, easy, and accurate. It was especially useful when practitioners refracted with loose lenses, where they could benefit from any technique that would decrease the number of “trips” they had to make to the trial lens case. However, now that most ophthalmic examination rooms are equipped with phoropters rather than trial lenses, estimation techniques tend to be underused, and most retinoscopists skip ophthalmoscopic retinoscopy altogether, and merely dive headlong into neutralization. We have chosen to replace terms such as “estimation techniques” with “ophthalmoscopic retinoscopy” to indicate a coherent optical basis rather than describe a collection of estimating “tricks.”
Ophthalmoscopic retinoscopy is quite helpful for the cycloplegic refraction of uncooperative infants and children, for patients being examined under anesthesia, or in any circumstance when the first attempt at neutralization does not yield a useful reflex. Most retinoscopists who only use the retinoscope for neutralization retinoscopy may be unfamiliar with the technique of estimation by ophthalmoscopic retinoscopy (Table 1).
TABLE 1. Differences Between Ophthalmoscopic Retinoscopy and Neutralization Retinoscopy | ||||||||||||||||||||||||||||||||||||
---|---|---|---|---|---|---|---|---|---|---|---|---|---|---|---|---|---|---|---|---|---|---|---|---|---|---|---|---|---|---|---|---|---|---|---|---|
|
The reader must understand four specific differences between the performance of ophthalmoscopic retinoscopy and neutralization retinoscopy. First, during ophthalmoscopic retinoscopy, the working distance starts at 5 cm from the patient and ends at 66 cm, whereas the working distance remains at 66 cm for neutralization retinoscopy (Fig. 6). Second, the sleeve is “spiraled” up during ophthalmoscopic retinoscopy—it is rotated while it is raised from the sleeve down to the sleeve up position, whereas for neutralization retinoscopy, it is left in the sleeve down position and swept from side to side across the pupil. Third, no lenses are used during initial ophthalmoscopic retinoscopy, but they are almost always used during neutralization retinoscopy. Fourth, the end point for ophthalmoscopic retinoscopy is a clear, sharp “ophthalmoscopic” image of the retinoscope streak, whereas for neutralization retinoscopy the end point is the neutralization reflex where the pupil immediately fills with an unfocused image of the streak.
The basic idea behind ophthalmoscopic retinoscopy is that the retinoscopist can determine when the focal point of the retinoscope falls on the focal point of the patient’s eye. When this occurs, the retinoscopist can see the image of the retinoscope streak as a sharply focused line in the patient’s pupil. By altering both the distance of the retinoscope to the patient’s eye and position of the focal point of the emitted streak from the retinoscope head (which is another way of saying the “vergence” of the emitted streak), the retinoscopist can superimpose the focal point of the retinoscope onto the focal point of the patient.
OPHTHALMOSCOPIC RETINOSCOPY OF SPHERICAL EYES
When performing ophthalmoscopic retinoscopy, the retinoscopist begins with the retinoscope 5 cm (about 2 inches) away from the patient’s eye with the sleeve all the way down (Fig. 7). The streak exits the retinoscope head as diverging light that comes to focus at a distance 40 cm behind it (behind the retinoscopist’s head) in virtual space. If the patient is a -2.25 D myope, the focal point of the retinoscope lies in the same point in space as the focal point of the patient, and the retinoscopist sees a finely focused streak of light in the patient’s pupil. It does not matter that the focal point of the retinoscope is in virtual space; it only matters that the focal point of the retinoscope is coincident with the focal point of the patient. At this point, the patient’s and retinoscope’s focal points are conjugate. The retinoscopist now rotates the streak 360 degrees by rotating the sleeve in the retinoscope handle. If the streak image is in focus in all meridians, the patient has no astigmatism, and ophthalmoscopic retinoscopy is complete at this stage. However, if the streak is in sharp focus in one orientation, but out of focus in another, the patient has astigmatism. Estimation of the refractive errors of astigmatic individuals is discussed toward the end of this section. For now, let us concentrate on eyes with spherical refractive errors.
Emmetropia and Mild Ametropia
If the patient’s refractive error is anything other than -2.50 D, the image of the streak in his pupil as seen by the retinoscopist will be out of focus—the farther the patient is from -2.50 D, the more out of focus the image will be. At this point, the retinoscopist slowly raises the retinoscope sleeve while rotating the streak 360 degrees (a technique known as “spiraling”). She keeps the retinoscope head 5 cm from the patient’s eye throughout this step. As the sleeve is raised the first one third of its range, the focal point of the retinoscope moves farther and farther from the back of the retinoscopist’s head until it lies on the horizon when the sleeve is approaching one third of the way up. If the image of the streak is in focus at this point, the patient’s focal point must lie at infinity, and he must therefore be emmetropic (Fig. 8). All patients with refractive errors between emmetropia and 2.25 D of myopia should be discovered up to this point with the sleeve between all the way down and one third of the way up, with the retinoscope positioned 5 cm from the patient.
The retinoscopist can easily learn to calibrate the sleeve position for the vergence and refraction at the 5-cm distance and depend on proprioception and a memorized calibration scale for this information. The mechanics of most retinoscopes make it difficult to permanently display this information on the handle. Fortunately, it is possible to place a written calibration on the handle of the Heine ophthalmoscope so that the 5-cm ophthalmic retinoscopy can be read with precision (see Fig. 5). The text in the preceding section explains how to calibrate any retinoscope, and the exercise can help you understand the optics.
If the retinoscopist gets much above the midsleeve position with the streak not yet coming into focus, she continues to spiral the sleeve up. She is now in the area where the image of the streak of patients with low hyperopic refractive errors will appear in focus. This occurs because the streak is emitted in a converging nature when the sleeve is raised above the one-third mark. The focal point of the streak is now located in space behind the patient’s head. When the sleeve is just barely above one third of the way up, the focal point of the streak is far behind the patient’s head, near the horizon, and will be conjugate with the patient’s focal point only if he is a weak hyperope (Fig. 9). As the sleeve is raised farther, the focal point of the retinoscope moves closer to the back of the patient’s head. When the sleeve is raised all the way up, the focal point of the retinoscope lies 33 cm in front of it—roughly 25 cm behind the patient’s cornea. The focal point of the retinoscope will be conjugate with the focal point of the patient if he is a + 4 hyperope. All hyperopes with refractive errors lower than + 4 should be discovered in this way with the sleeve between one third and all the way up and the retinoscope positioned 5 cm from the patient. Thus, the retinoscopist can estimate or confirm the refraction of all patients between -2.25 and + 4 by merely spiraling the sleeve upward while holding the retinoscope 5 cm from the patient’s eye (Fig. 10).
HIGH HYPEROPIA AND HIGH MYOPIA
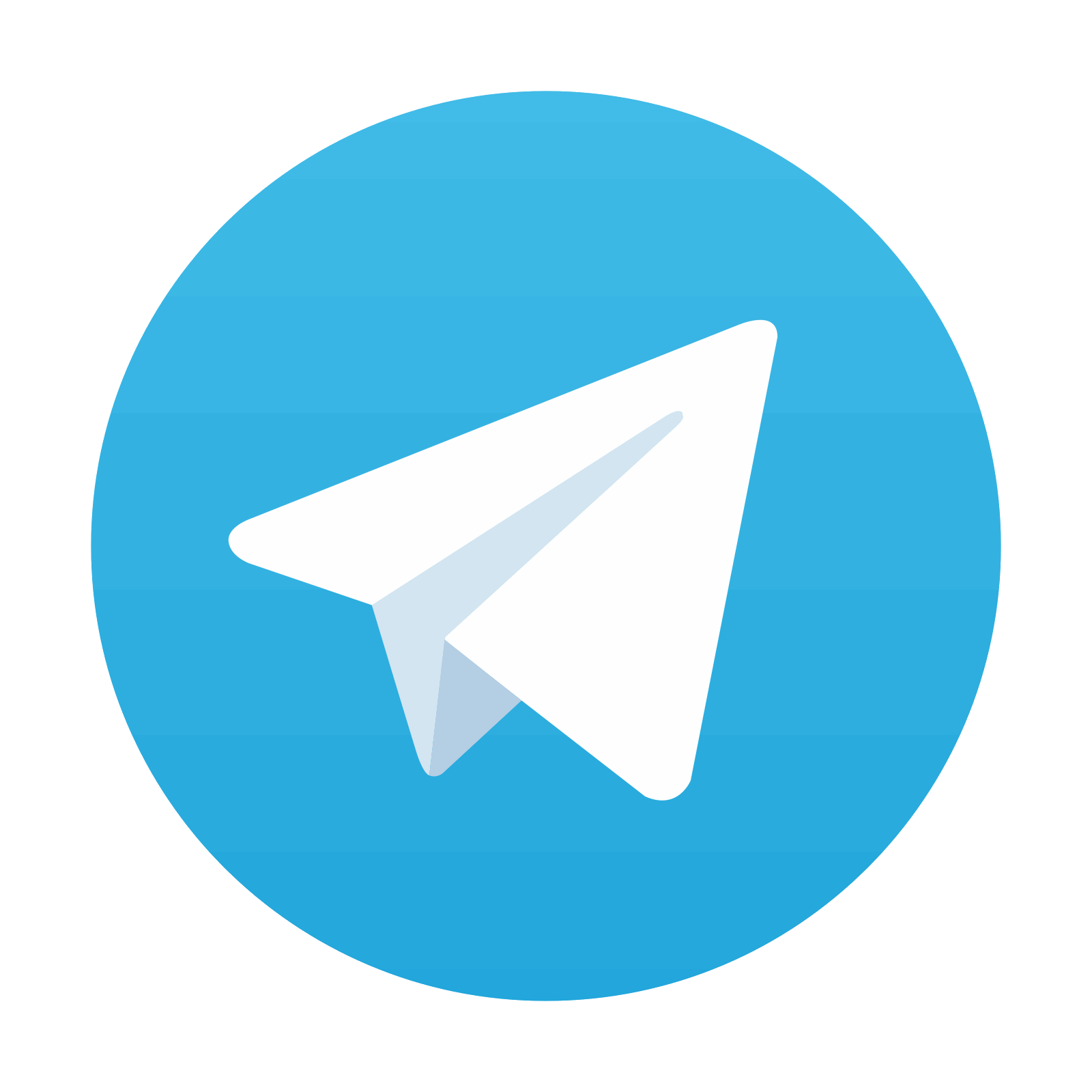
Stay updated, free articles. Join our Telegram channel

Full access? Get Clinical Tree
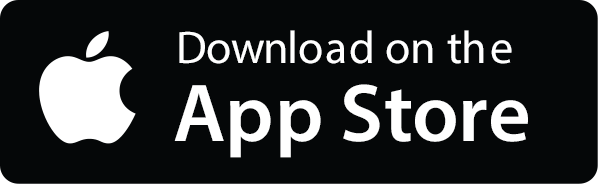
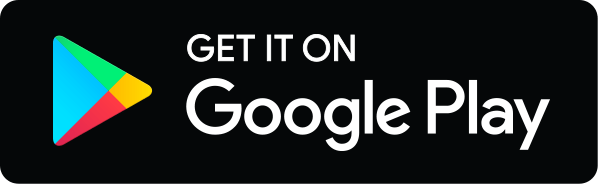