Country
GA (weeks) and BW (g)
Notes
Argentina [74]
≤32 weeks and/or 1500 g
Include also 1500–2000 g with unstable clinical course, predisposing factors or prolonged oxygen therapy
Brazil [16]
≤32 weeks and/or ≤1500 g
Include also larger and more mature babies with illnesses and other risk factors, e.g. sepsis, respiratory problems, multiple births
Canada [73]
<31 weeks and <1251 g
Include also babies 1251–2000 g if at high risk due to complex clinical course
Chile [75]
<33 weeks and <1500 g
Include also babies between 1500 and 2000 g with unstable clinical course, predisposing risk factor or prolonged oxygen therapy
Colombia [77]
≤32 weeks and/or ≤1800 g
Include also >1800 g with unstable clinical course, predisposing factors or prolonged oxygen therapy
Mexico [76]
≤34 weeks and/or ≤1750 g
Sweden [23]
<31 weeks
Include also larger babies severely ill
India [17]
<34 weeks and/or <1750 g
Include also babies 34–36 weeks or 1750–2000 g if there are risk factors
UK [7]
<31 weeks or <1251 g one criterion to be met
Must be screened. No additional sickness criteria
UK [7]
31 to <32 weeks or 1251–1501 g one criterion to be met
Should be screened
USA [42]
≤30 weeks or <1500 g
Include also “selected” 1500–2000 g or >30 weeks if unstable clinical course with cardiorespiratory support and at high risk
South Africa [78]
<30 weeks and <1500 g
Also selected 1500–2000 g if risk factors or oxygen monitoring suboptimal—includes guidance on oxygen monitoring
Which Infants Need to be Screened?
High-Income Countries
In high-income countries, with a relatively uniform high level of neonatal care, most have devised inclusion criteria, based on gestational age, birth weight alone or with sickness criteria. There is very little data on the specificity of illness factors which are risk factors for ROP [7]. It is pertinent to note that in high-income countries with excellent neonatal care, inappropriately excessive oxygen administration is rare (not denying the importance of oxygen in ROP pathogenesis) and the additional risk factors are likely to be sepsis, transfusions, general sickness, and prolonged cardiorespiratory support—which are most probably nonspecific indicators of illness. Almost all ROP requiring treatment affects babies <31 weeks GA or <1250 g BW, but it does occasionally occur in larger and more mature babies [7–9]. More data is required to determine whether the safest, most effective, and efficient method to include the outliers is by raising the GA and BW bar and disregarding a sickness score, or by setting lower level limits and combining GA and BW with a “sickness” criterion which will robustly identify the larger infant.
Low- and Middle-Income Countries
In low- and middle-income countries, with variations in the standard of neonatal care, larger and more mature infants are at risk of developing sight-threatening ROP [1, 10–13] compared with those in high-income countries. Recent publications from India illustrate the issue with infants <36 weeks GA and >2500 g BW requiring treatment [13–15]. Clearly basing inclusion criteria on GA and BW alone under these circumstances would be quite inappropriate as many babies would be unnecessarily screened and the manpower demand for screening simply could not be met. Thus in these communities a sickness criterion needs to be included for those larger and more mature babies without the GA and BW but who are at risk of sight-threatening ROP as screened.
As well, in many instances, the GA may not be known. As indicated in Table 3.1 the GA and BW inclusion criteria are wider in those middle-income countries which have guidelines such as Brazil [16] and India [17]. Inappropriate excessive oxygen administration—sometimes unblended—has long been suspected to be a risk factor. Shah et al. reported a high incidence of aggressive posterior ROP (APROP) in babies <35 weeks GA who had received unblended oxygen and that following crude oxygen blending this incidence fell dramatically [14].
Clinical management within the NICU’s is continually changing, so reevaluation of evidence-based inclusion criteria for infants requiring a screening eye exam will be necessary from time to time.
When to Screen?
ROP Natural History
An effective and efficient screening program requires understanding of the natural history of ROP. This has been studied in population-based [18–20] and treatment studies [ 21–23]. Findings have been very consistent over the 20-year period in high-income countries, showing that the onset and progression of ROP are governed predominantly by postmenstrual and not postnatal age, although there is a slight acceleration of the process in the most immature infants [20]. The rate of progress through the stages is approximately one stage per 7–10 days: thus in the Early Treatment of Retinopathy of Prematurity Study (ETROP) the median age for stages 1, 2, and 3 and plus disease was 34.1, 35.1, 36.6, and 36.0 weeks post menstrual age (PMA), respectively [24]. ROP very rarely requires treatment before 31 weeks PMA and the mean age for treatment of Type I (prethreshold) and threshold disease in ETROP was 35.2 weeks PMA (range 30.6–42.1 weeks) and 37.0 weeks PMA (range 31.9–46.6 weeks) essentially identical to previous studies [25].
With respect to middle- and low-income countries and larger infants, Carden et al. reported on 100 preterm infants <1900 g BW and <35 weeks GA in Vietnam who required treatment for threshold ROP at 36.25 weeks PMA (range 31.4–44.3 weeks) [11]. From India, Shah et al. reported on laser treatment to 93 babies (<2250 g BW and <35 weeks GA) at mean age of 37 weeks PMA (range 30–40 weeks), while Jalali et al. (2011) treated 115 babies with APROP at a mean age of 34.6 weeks PMA (range 31–51 weeks PMA) [14, 15]. Thus, information to date confirms that the natural history of ROP is governed by postmenstrual age so that the mean age of infants requiring treatment at threshold ROP is approximately 37 weeks and at Type I prethreshold around 35 weeks even in larger infants. Progression through the stages of disease will be compressed in the larger infants and the National Neonatology Foundation of India guideline recommends screening by 2–3 weeks of age [17].
When to Start Screening?
Screening should commence before ROP requires treatment (Table 3.2). Several ROP guidelines have given a single postnatal age for the commencement of screening examinations, e.g., 6–7 weeks for the 1995 UK guideline [3] and 4–6 weeks in the Vision 2020 Action Plan [4]. While this had the merit of simplicity, it did not take into consideration the natural history of ROP and consequently required excessive examinations of the most immature infants and yet would have missed the opportunity for treatment in the largest and most mature infant. Several national guidelines have now followed the approach suggested by Reynolds et al. in which the commencement of screening is tailored to the infant’s GA [26]. This is shown in Table 3.2 and has been extended by authors beyond 32 weeks for the purpose of this article. It is important to note that larger birth weight infants should have earlier screening exams.
Table 3.2
Suggested age for first screening examination
Age at first ROP screening examination | ||
---|---|---|
Gestational age (weeks) | Postnatal Age (weeks) | Postmenstrual age (weeks) |
22 | 8 | 30 |
23 | 7 | 30 |
24 | 6 | 30 |
25 | 5 | 30 |
26 | 4 | 30 |
27 | 4 | 31 |
28 | 4 | 32 |
29 | 4 | 33 |
30 | 4 | 34 |
31 | 4 | 35 |
32 | 3 | 35 |
33 | 2 | 35 |
34 | 2 | 36 |
35 | 2 | 37 |
When Screening Can Be Safely Terminated
The decision to terminate screening safely is critical and depends on whether or not the infant had any ROP. In those infants not ever having had any ROP, most guidelines recommend that eye exams should continue until there is adequate retinal vascularization to zone III and not to stop screening before 37 weeks of PMA. Infants that have had ROP throughout their clinical course require at least two examinations with signs of regression of ROP.
Telemedicine Approach to ROP
Telemedicine refers to the use of information technologies to support health care between participants who are separated from each other. It may be a possible solution to some of the screening challenges in the management of ROP.
Over the last 15 years, research studies using a telemedicine approach to ROP screening have consistently demonstrated the ability to identify infants at risk for severe ROP needing treatment. There are over 35 peer-reviewed published studies that document feasibility and accuracy of this approach [ 27–31]. Advances in imaging techniques have enabled the manufacture of digital cameras specifically for imaging the posterior segment and retina of the premature infants. The RetCam digital camera system (Clarity Medical Systems, Pleasanton, California, USA) is the most commonly used camera for neonatal ophthalmic imaging. The wide-angle lens gives a panoramic view of the retina, and the capture and save feature enables photographic documentation of retinal pathology which may become part of the permanent medical record in addition to its use in telemedicine. Retinal photographic documentation of infants at risk for ROP using the RetCam camera with remote interpretation of digital images has been favorably assessed in many studies. Recently, a large multicenter study published results that support the validity of remote evaluation by trained nonphysician readers of digital retinal images taken by trained nonphysician imagers from infants at risk for severe ROP [32–34].
Several telemedicine studies have documented identification of severe ROP disease one to two weeks earlier than the clinical binocular indirect ophthalmoscopy (BIO) standard [35, 36]. Earlier identification of disease leads to earlier treatment of severe ROP and early treatment results in better visual outcomes [37–39]. This is consistent with major clinical trials in diabetic retinopathy that document the efficacy of digital imaging with remote evaluation as better than ophthalmologists’ exams in detecting the features of diabetic retinopathy [40, 41]. The use of telemedicine to manage infants with ROP offers the potential to address the shortage of physician expertise, standardize, and improve the quality of ROP evaluations and optimize the timing of treatment of premature infants.
An Ophthalmic Technology Assessment report commissioned by the American Academy of Ophthalmology has evaluated the accuracy of detecting clinically significant ROP using wide-angle digital retinal photography from the literature currently available [42]. It reviewed seven level I papers and three level III papers. Of these ten papers, seven of them reported a high sensitivity and specificity for detecting ROP needing treatment using digital imaging.
Chiang et al. reported on multiple “Real-World ROP Management Programs” [43]. These programs have successfully set up ROP telemedicine programs and have published data supporting their validity. The largest published dataset of infants screened for ROP using telemedicine is from Bangalore, India. This telemedicine program, KIDROP (Karnataka Internet Assisted Diagnosis of Retinopathy of Prematurity), has been screening in remote neonatal intensive care units (NICU’s) in rural regions where ROP screening does not exist [44–47]. Trained and certified technicians visit multiple hospitals on a fixed schedule in many districts of Karnataka state (inhabited by 64 million people), and using the Retcam Shuttle, image infants who are either resident to that NICU or referred to the program. Images are stored, analyzed, and uploaded on a secure Tele-ROP platform (i2i Tele-solutions Ltd, Bangalore, India) for the expert to view and report on a smart phone, tablet or computer, in real-time, using a standardized template [17, 45]. In Germany, Lorenz et al. showed 100% detection of suspected ROP treatment warranted cases in a prospective study [48]. In the USA, the Stanford University Network for Diagnosis of ROP has reported a 100% sensitivity and 90% specificity at detecting treatment warranted cases of ROP [49]. The Joint Technical Report on “Telemedicine for Evaluation of Retinopathy of Prematurity” recently published by the American Academy of Pediatrics Section on Ophthalmology, American Academy of Ophthalmology, and American Association of Certified Orthoptists also reinforces the important impact of telemedicine in the future management of ROP [50].
Future Technology of ROP Screening
Less than 10% of neonates screened for ROP will require treatment, so the vast majority either do not develop ROP or it is mild and self-limited. This offers two options for screening: identifying the eye not at risk of developing sight-threatening ROP or predicting reliably the eye which is likely to progress. Predictive factors for likelihood of disease progression can be reliably isolated. Predictive factors for ROP progression studied thus far include post natal weight gain, serum IGF-1 levels and quantifiable vessel changes in the retina. The dichotomous distinction between disease presence and disease absence may be a warranted first step in assessing these infants. There is also growing evidence that genetic factors are linked with disease severity, and in the more distant future, genetic screening may also add to the decision-making process of which babies are more likely to benefit from eye screening examinations.
An alternative and complementary approach to identifying either the eye with no/mild or severe ROP is through retinal vessel analysis. Image analysis techniques have progressed rapidly, and over the last decade retinal vessel analysis tools have been developed specifically to assess the vascular changes in the posterior pole associated with plus disease of ROP. Image segmentation isolates areas of interest within an image. There are many different image segmentation techniques and these can broadly be divided into ridge-based and region-based techniques. These techniques have been applied to retinal images by many teams globally to identify blood vessels in images. Locating the tiniest blood vessels in preterm neonates has been challenging for software, but progress is being made and the accuracy of these systems may consequently equip them to be the most sensitive and specific tools for finding features in adult images. The first reports of semi-automated image analysis techniques for identifying and then quantifying retinal vessels from RetCam images were from Imperial College London where Retinal Image multiScale Analysis (RISA) had been developed [51].
RISA has been used to quantify arterial tortuosity in ROP and subsequently showed that in plus disease arteriolar and venous curvature, diameter and tortuosity index are all increased compared to those without plus disease [52]. The rate of change in venous parameters has been correlated with plus disease development using RISA, with the highest area under the curve reported in venous tortuosity index for weekly rate of change [53]. Additional computer-assisted vessel detection software has been developed, including, ROPtool, developed at Duke University, USA [54, 55] and Computer-Aided Image Analysis of the Retina (CAIAR), developed from RISA to enable quicker image analysis due to more accurate image segmentation techniques [56–58].
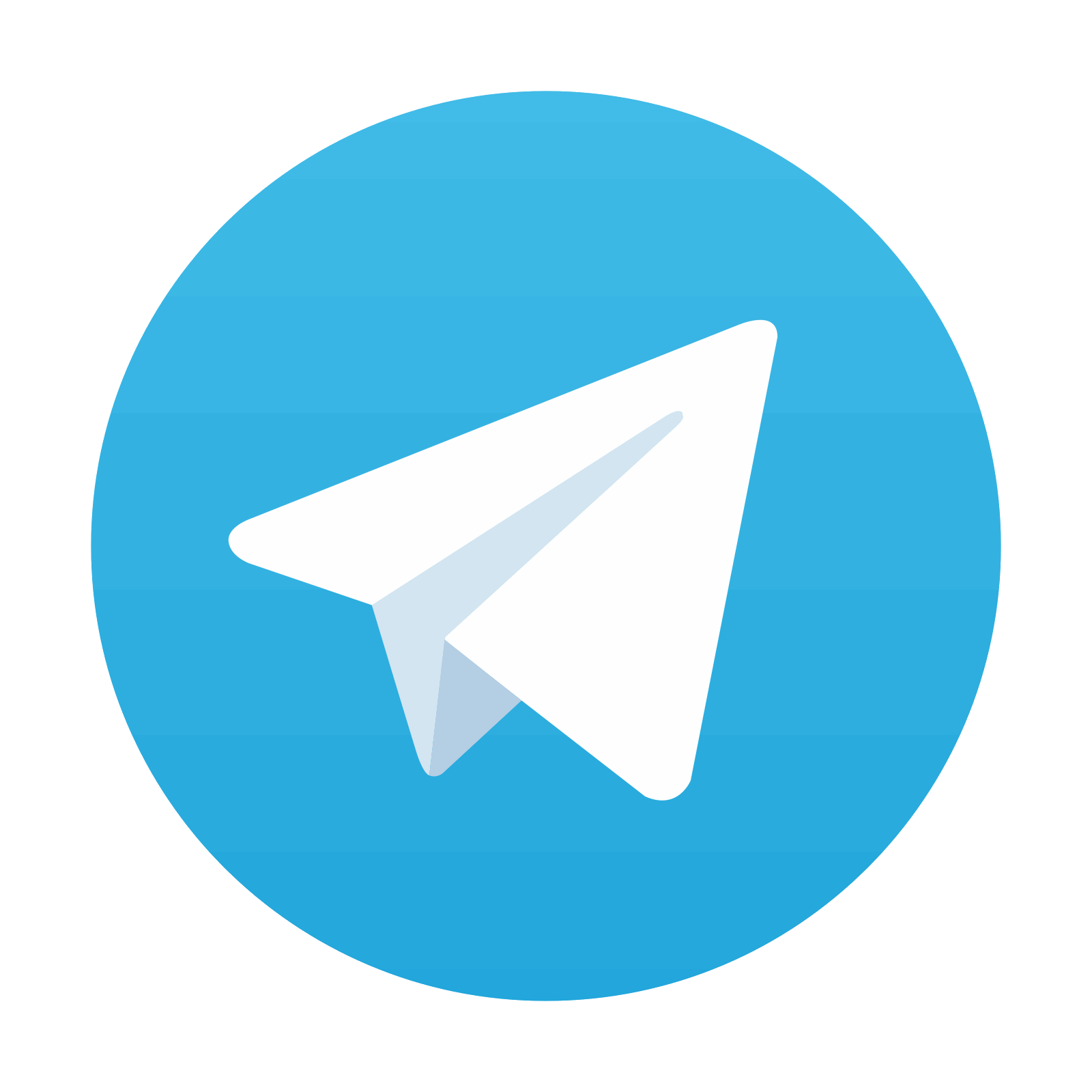
Stay updated, free articles. Join our Telegram channel

Full access? Get Clinical Tree
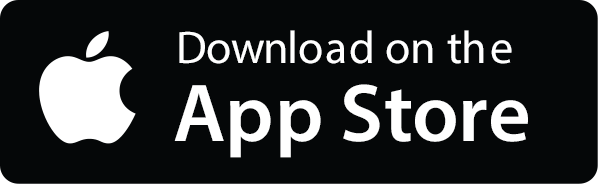
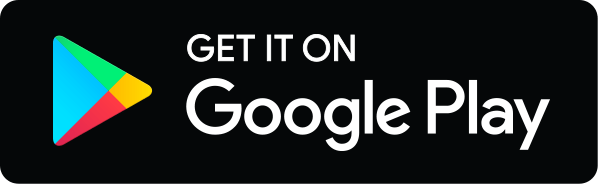