Clinical background
Retinopathy of prematurity (ROP) is a leading cause of childhood blindness worldwide and develops after birth in preterm infants, especially those born at less than 1500 g birth weight or younger than 32 weeks’ gestational age. There are no symptoms or obvious signs of ROP. Therefore, longitudinal dilated examinations of the retinas of infants at risk are necessary to determine when treatment is needed, or that retinal vascular development is complete and the risk of ROP is no longer present. The goal of examinations is to detect and treat severe ROP in order to prevent progressive retinal detachment, in which poor vision occurs even when successful retinal surgery can be performed.
Based on the International Classification of ROP (ICROP), ROP is characterized by several parameters: zone, stage, extent of stage, and the presence or absence of plus disease. At any examination, the risk of a bad outcome depends on the presence of plus disease or stage 3 ROP (both defined below and important features of severe ROP) as well as the postgestational age of the infant (i.e., the gestational age + chronologic age from birth in weeks).
The zone of ROP is the retinal area supplied by retinal vessels and is an indicator of the extent of retinal vascular development ( Figure 72.1 ). Zone I is the smallest, having the largest area of avascular retina. Zone III is the most vascularized (except for complete vascularization) and has a very low risk of a poor outcome. There are five stages of ROP. Stages 1 through 3 are the acute forms of ROP and are characterized by the appearance of the retina at the junction of vascular and avascular retina. A line (stage 1) or ridge (stage 2 ( Figure 72.2A ) can often regress without developing into severe ROP. Stage 3 ROP has intravitreous neovascularization, a feature of severe ROP ( Figure 72.2B ). Stages 4 ( Figure 72.2C ) and 5 ( Figure 72.2D ) describe partial or complete retinal detachment, respectively, and are associated with vitreous and fibrovascular changes. The extent of ROP indicates the number of clock hours of a stage. Plus disease is the presence of dilated and tortuous retinal vessels and is a feature of severe ROP ( Figure 72.3 ).



One point of clarification is the difference between retinal drawings and images of dissected retinal flat mounts. The retina covers the inner sphere of the eyeball, and when dissected, must be cut with relaxing incisions in order to flatten it on to a microscope slide. The result is a clover-leaf appearance ( Figure 72.4 ). However, clinicians and surgeons represent the retina as a round clock face and use clock hours to describe the location of pathologic features on the retina.

Severe ROP portends an increased risk of developing retinal detachment and poor vision ( Box 72.1 ). Therefore treatment, preferably with laser, is strongly considered at certain levels of severe ROP, threshold disease and type 1 prethreshold ROP ( Table 72.1 ). A form of severe ROP is aggressive posterior ROP (APROP), which appears at young postgestational ages in preterm infants, who have immature retinal vascular development and large avascular retinal areas ( Box 72.2 ). Neovascularization in stage 3 appears broad and flat in APROP ( Figure 72.5 ). Despite standard of care treatment, these eyes often have poor outcomes.
- •
Definitions of severe ROP have changed from the Multicenter Clinical Trial for Cryotherapy for Retinopathy of Prematurity (CRYO ROP) study to the Early Treatment for ROP (ETROP) study
- •
CRYO ROP study termed severe ROP as threshold disease: the presence of zone I or II, stage 3 (5 contiguous or 8 total clock hours with plus disease)
- •
ETROP defined type 1 prethreshold ROP as zone I, any stage with plus disease, or stage 3, without plus disease, or zone II, stage 2 or 3 with plus disease
- •
Treatment, preferably with laser, is now considered when the risk of a poor outcome approaches 15% (ETROP) whereas with threshold ROP the risk is 50%
- •
Cryotherapy has been reported to be associated with more inflammation and with subretinal plaques. Laser is currently preferred
- •
Consideration of other pharmacologic therapies is under way
Threshold ROP (CRYO-ROP) (risk of unfavorable outcome approaches 50%) | Type 1 prethreshold ROP (ETROP) (risk of unfavorable outcome is ≥ 15%) |
---|---|
Zone I or II: stage 3 (5 contiguous or 8 total clock hours with plus disease) | Zone I: any stage with plus disease |
Zone I: stage 3 without plus disease | |
Zone II: stage 2 or 3 with plus disease |
- •
APROP presents early with plus disease and often zone I vascularization
- •
Outcomes have been poor both based on laser treatment for acute early ROP and later treatment for stage 4 fibrovascular ROP
- •
Stage 3 ROP has a different appearance than in most severe ROP. The neovascularization is flat and can be easily misidentified as intraretinal vessels at the junction with the avascular zone. Therefore, it is important to follow carefully after laser. Skip lesions will seem to appear when flat neovascularization regresses. More laser should be applied

Historical development
In the 1940s, ROP manifested as a white pupil and was termed retrolental fibroplasia (RLF). Most RLF was stage 5 ROP with a retrolental membrane and occurred in older and larger infants. Michaelson, Ashton and Cook, and Patz described the hypothesis of hyperoxia-induced vaso-obliteration followed by relative hypoxia and the release of an “angiogenesis” or “vasoproliferative” factor. Further validation from studies with animal models (see below) led to improved monitoring of oxygen to preterm infants in neonatal intensive care units (NICUs). ROP virtually disappeared, because hyperoxia at birth was avoided. (Standard of care today is maintenance of infant oxygen saturation in the mid 80 and low 90 percentages.) But as infants of younger gestational ages and of lower birth weights have survived, ROP has re-emerged. Besides absolute oxygen level, now fluctuations in oxygen also are recognized as important in the pathogenesis of severe ROP ( Box 72.3 ).
- •
ROP described in the 1940s resulted from high inspired oxygen at birth, likely causing capillary obliteration and subsequent relative hypoxia
- •
Most severe ROP in the USA today is not from high unregulated oxygen at birth but a result of oxygen fluctuations
- •
The effect of supplemental oxygen is complex and may depend on the age of the infant and when the hyaloidal circulation regresses, photoreceptors mature, and the choroid is unable to support supplemental inspired oxygen
- •
Long-term effects of lower inspired oxygen are concerning until more knowledge is available of the effect on infant brain development
- •
Also important are the changes in infant growth factors, such as insulin-like growth factor 1 and binding proteins
Xenon photocoagulation was reported as a treatment for ROP in 1976 by Nagata. The initial observations of Folkman et al of a tumor angiogenesis factor were described in the Friedenwald lecture presented by Arnall Patz in 1980. In 1984, ICROP described and characterized stages of ROP earlier than stage 5. The Multicenter Clinical Trial for Cryotherapy for Retinopathy of Prematurity (CRYO-ROP) found that obliteration of the avascular retina in ROP with cryotherapy at a certain threshold of severity ( Table 72.1 ) significantly reduced blindness and retinal detachment. The CRYO-ROP study group reported on the natural history of ROP (for review, see McColm and Hartnett ). Since the development of laser via indirect delivery, treatment of acute ROP has been preferentially performed using laser ( Figure 72.6 ), which is less destructive and causes less inflammation than cryotherapy ( Box 72.4 ). The Early Treatment for ROP Study (ETROP) found that treatment of eyes with type 1 prethreshold ROP ( Table 72.1 ) further reduced the risk of a bad outcome. ETROP also emphasized plus disease and reduced the importance of extent of stage in defining high-risk eyes.

- •
Consider laser for type 1 prethreshold ROP and for all threshold ROP urgently
- •
Treat avascular retina 360°. Space laser
–1 spot size apart. Too much laser will increase inflammation. Too little will result in skip lesions
- •
If hemorrhage precludes treatment in an area, monitor and add laser as blood clears. Consider referral to pediatric retinal surgeon for consultation and possible lens-sparing vitrectomy if clearing does not occur within 2 weeks
- •
After laser, monitor weekly
- •
For persistent vascular activity (plus disease, neovascularization), add laser to skip lesions or in between laser spots
- •
Add laser if flat neovascularization in aggressive posterior ROP regresses, leaving a skip lesion
- •
For persistent or new ridge thickening or fibrovascular contraction (junction of vascular and avascular retina), vitreous haze, or recurrent plus disease, consider lens-sparing vitrectomy to remove core vitreous and lyse adhesions between anterior eye and junction, optic nerve, and circumferential traction
Epidemiology
The Institute of Medicine reported preterm births up 30% from 1981 ( www.iom.edu/Object.File/Master/35/975/pretermbirth.pdf ), now accounting for 12.5% of all births in the USA. With increases in prematurity, ROP has also become a leading cause of childhood blindness worldwide. A report from a national registry of children in the USA (Babies Count) indicated that ROP was the earliest cause of visual impairment and one of the three most prevalent visual conditions along with cortical visual impairment and optic nerve hypoplasia. ROP of any stage affects approximately 16 000 infants each year in the USA. Most early stages of ROP resolve and about 1100 infants require treatment. Even with treatment, blindness occurs in 550 infants/year (National Eye Institute statement on ROP, October 2007: http://www.nei.nih.gov.easyaccess2.lib.cuhk.edu.hk/health/rop/ ). In the USA, ROP is seen more commonly in Caucasians than African Americans but once severe ROP occurs, the outcomes appear similar. Asians also have an increased risk of ROP. ROP in developing nations is seen in infants of larger birth weight and older gestational ages than in the USA, possibly because of variations in ethnic groups, regulation and monitoring of oxygen delivery, and the availability of prenatal care.
Genetics
A genetic component to ROP appears to be present, although the understanding of the contribution of genetic polymorphisms to the risk of ROP is incomplete. Based on a retrospective analysis in monozygotic and dizygotic twins, a 70% variance in the susceptibility of ROP was found to be from genetic factors.
The Norrie disease gene produces the gene product, norrin, which is also a downstream ligand for receptors in the Wnt pathway. The Wnt pathway and norrin are important in retinal and vascular development and abnormalities are present in patients with Norrie disease. Genetic mutations in the Norrie disease gene [Xp11.2-11.3] were reported to account for 3% of cases of advanced ROP, but another study having racially diverse populations reported no significant increase in the prevalence of polymorphisms in infants with severe ROP compared to control premature infants with no or minimal ROP. One study reported on samples from 109 patients with diverse pediatric vitreoretinopathies including ROP and 54 controls. Mutations within the cysteine knot configuration of the Norrie disease gene were associated with severe retinal dysplasia whereas other polymorphisms within the gene had less severe vitreoretinopathies. This study suggested that, within severe ROP, polymorphisms in the Norrie disease gene may account for a subgroup with severe retinal dysplasia. Controversy in the association of mutations in the Norrie disease gene may also reflect differences based on ethnic variability.
One study found an association of severe ROP with certain polymorphisms in the gene of vascular endothelial growth factor (VEGF), whereas another study found different associations. Overexpression of VEGF was reported in an unusual case of ROP. Some polymorphisms in VEGF may be linked differentially to others and the effects of these may differ among ethnic groups. Despite the finding that low serum systemic insulin-like growth factor-1 (IGF-1) was associated with more severe ROP, one study failed to show a relationship between a prevalent polymorphism in the IGF-1 receptor and the presence of ROP. The role of genetics requires greater study and will continue to be elucidated along with the effect of environmental factors on gene function.
Diagnostic workup and treatment
The published guidelines from the American Academy of Pediatrics, American Academy of Ophthalmology, and American Association for Pediatric Ophthalmology and Strabismus are:
infants with a birth weight of less than 1500 g or gestational age of 30 weeks or less and selected infants between 1500 and 2000 g or gestational age of more than 30 weeks with an unstable clinical course
should have retinal screening with pupil dilation and indirect ophthalmoscopy. The first examination is recommended at 4–6 weeks after birth or at 31 weeks’ postgestational age (whichever is later) and prior to discharge from the hospital. Examinations are performed approximately every 2 weeks if there is incomplete retinal vascular development without ROP or weekly with evidence of ROP until treatment is recommended ( Table 72.1 ) or until regression of ROP occurs and retinal vascular development is completed.
The use of wide-angle photography or quantification of plus disease to detect high-risk ROP is being studied. Images would be obtained at a remote facility and transmitted for review to a reading center. The center would detect “clinically significant ROP” and notify the remote facility that the infant requires an examination and possible treatment by a qualified ophthalmologist. A successful system requires careful coordination to avoid missing any infant with severe ROP.
For eyes that progress to stage 4 ROP (partial retinal detachment), the vitreous appears to play an important role. Both changes at the junction and fibrovascular organization within the vitreous have been associated with and are predictive of progressive stage 4 ROP. The severity of the retinal detachment depends on the total area of the fibrovascular proliferation that grows and interacts with the vitreous collagen. The vitreous provides a scaffold for invading cells. Cell contraction on the vitreous collagen is believed to lead to tractional retinal detachments. Whereas most stage 4 ROP begins as a retinal detachment at the junction of vascular and avascular retina, APROP may produce more severe detachments because of extensive cell invasion into broad sheets of vitreous in the posterior retina, i.e. usually within zone I. From optical coherence tomography (OCT), changes at the vitreoretinal interface of the posterior retina are recognized ( Figure 72.7 ). The role of transforming growth factor-β (TGF-β) in wound healing and the changes in concentrations of TGF-β and VEGF in the developing preterm infant have been proposed. Study of the mechanisms of stages 4 and 5 ROP is difficult because of lack of ideal animal models and the difficulty in studying human infants. Once progressive stage 4 ROP is diagnosed, surgery is performed to release vitreous tractional forces that detach the retina.

Differential diagnosis
Several conditions can manifest as either early or late stages of ROP based on the progression of the disease. Examples include familial exudative vitreoretinopathy (FEVR), Norrie disease, and incontinentia pigmenti. Other conditions cause media opacity from diseased cornea, lens, vitreous, or retina and produce a white pupil or leukocoria mimicking stage 5 ROP. Notable examples include persistent fetal vasculature, a typically unilateral developmental abnormality; chronic vitreous hemorrhage; retinoblastoma, a childhood cancer; Coats disease, a retinovascular condition that can lead to exudative retinal detachment; and infectious endophthalmitis, that can occur from systemic infection or conjunctivitis, as in the case of Pseudomonas , or after surgery. It should be noted that endophthalmitis can occur in preterm infants, whereas other conditions usually occur in full-term infants or children.
Pathology
In ROP, the peripheral avascular retina lacks retinal vascularization. At the junction of vascular and avascular retina, aberrant angiogenesis (stage 3 ROP) grows into the vitreous. The avascular retina that forms after hyperoxia-induced vaso-obliteration in the cat or mouse or after fluctuations in oxygen in the rat (see below) is hypoxic. The hypoxia is believed to occur because of reduced oxygen supply from an incompletely developed retinal vasculature in the face of a regressing hyaloid vasculature, increased metabolic and oxygen demand of maturing photoreceptors, and the possible inability of the choroid to adjust its oxygen concentration in the young animal even with increases in inspired oxygen level. Although several growth factors, including erythropoietin, IGF-1, and hypoxia-inducible factor-1α (HIF-1α), have been reported to be involved in the pathomechanisms of ROP models, VEGF has also been shown to be consistently important in other human diseases with intravitreous neovascularization. The avascular retina has been shown to express VEGF in animal models. Also VEGF was overexpressed in the avascular retina of a human infant with stage 3 ROP. The junction of vascular and avascular retina was described as a proliferation of primitive vascular mesenchyme (possibly describing angioblasts) in a vanguard of advancing vasculature with an intraretinal band of endothelial cells in the rearguard, and extraretinal neovascularization extending into vitreous. With the change from a vascularly active process (stage 3 ROP) to a fibrovascular one (stage 4 ROP), there is often mesenchymal tissue that will fold upon itself to form a retinal detachment ( Figure 72.8 ).

Etiology
Environmental risk factors
Although genetics plays a role, outside stimuli, such as nutrition and oxygen, are important in the development of ROP. Arginyl-glutamine and omega-3 fatty acids have been shown to reduce intravitreous neovascularization or avascular retina, respectively, in the mouse oxygen-induced retinopathy (OIR) model.
Role of oxygen: animal models of oxygen-induced retinopathy
After the initial appearance of ROP/RLF in the 1940s, the importance of high oxygen at birth was appreciated and animal models of OIR were developed. Most of these models exposed animals to high constant oxygen, which has been found both to cause capillary obliteration and to inhibit the differentiation of precursor cells into endothelial cells. Now, high oxygen at birth is avoided in NICUs in the USA. However, these models continue to provide important data in understanding the role of oxygen exposure in retinal vascular development and in pathologic angiogenesis. The cat model was used in initial observations of hyperoxia-induced vaso-obliteration and in describing the role of astrocytes in retinal vascular development. The mouse OIR model permits the study of mechanisms of hyperoxia-induced vaso-obliteration and relative hypoxia by permitting the use of genetically manipulable animals. No model reproduces stage 4 or 5 ROP. However, the beagle OIR model develops retinal folds similar to those reported in human fibrovascular ROP at the junction of vascular and avascular retina.
The rat 50/10 OIR model provides the most relevant model of acute ROP in the USA today. Inspired oxygen extremes in the rat 50/10 OIR model led to rat arterial oxygen levels similar to the transcutaneous oxygen levels measured in a preterm infant who developed severe ROP. Also, rather than constant oxygen used in other models, the 50/10 OIR model exposes pups to repeated fluctuations in oxygen, which increase the risk of severe ROP. The 50/10 OIR model reproducibly and consistently develops first avascular retina (analogous to zone of human ROP), then vessel tortuosity (analogous to plus disease in ROP), and later intravitreous neovascularization (analogous to stage 3 ROP).
Other retinopathy models mimic conditions that occur in the preterm infant, including hypercarbia and metabolic acidosis. Newborn pups exposed to minute-to-minute oxygen fluctuations similar in extremes to what human preterm infants experience develop vascular abnormalities in the peripheral retinas, particularly if the fluctuations are performed around a hyperoxic rather than hypoxic mean.
Similar in all animal models is the creation of avascular retina associated with intravitreous neovascularization at junctions of vascular and avascular retina at a time during development when oxygen supply is limited because of insufficiently developed retinal vascularization and a regressing hyaloidal vasculature while oxygen demand is increased from maturing photoreceptors.
The role of supplemental oxygen
As discussed above, high constant oxygen caused vaso-obliteration through apoptosis, resulting in hypoxic avascular areas of retina, and subsequent intravitreous neovascularization (see above). Likewise, fluctuations in oxygen caused apoptosis of endothelial cells, in part causing larger avascular retinal areas in the 50/10 OIR model.
Some studies showed that supplemental oxygen reduced the severity of OIR. Mice raised in sustained hyperoxia beyond postnatal day (p)12 had less vaso-obliteration and neovascularization compared to mice exposed to the standard OIR model. Rats exposed to oxygen fluctuations and recovered in supplemental oxygen (28%) rather than room air had reduced intravitreous neovascularization at some time points. Despite these data, the Supplemental Therapeutic Oxygen for Prethreshold ROP (STOP-ROP) multicenter clinical trial did not find an overall significant benefit from supplemental oxygen given to infants with prethreshold ROP.
In the 50/10 OIR model, supplemental oxygen increased nicotinamide adenine dinucleotide phosphate (NADPH) oxidase activation, accounting partly for pathologic intravitreous neovascularization, even though it also reduced neurosensory retinal VEGF. Furthermore, hypoxic retina, quantified by insoluble retinal pimonidazole (Hypoxyprobe), was not reduced with supplemental oxygen. These results show that increased oxygen in the retinal vasculature reduces the stimulus for VEGF production but does not overcome overall retinal hypoxia that may occur with increased metabolic demand of developing photoreceptors. Furthermore, the retinal hypoxia appears unmet by the choroid. It was previously found that, unlike in the adult rat, the choroid in the p15 rat was unable to support increased oxygen tension with supplemental oxygen. Therefore, supplemental oxygen may not have as beneficial an effect as hoped in the retina and it can also lead to greater complications of pulmonary disease.
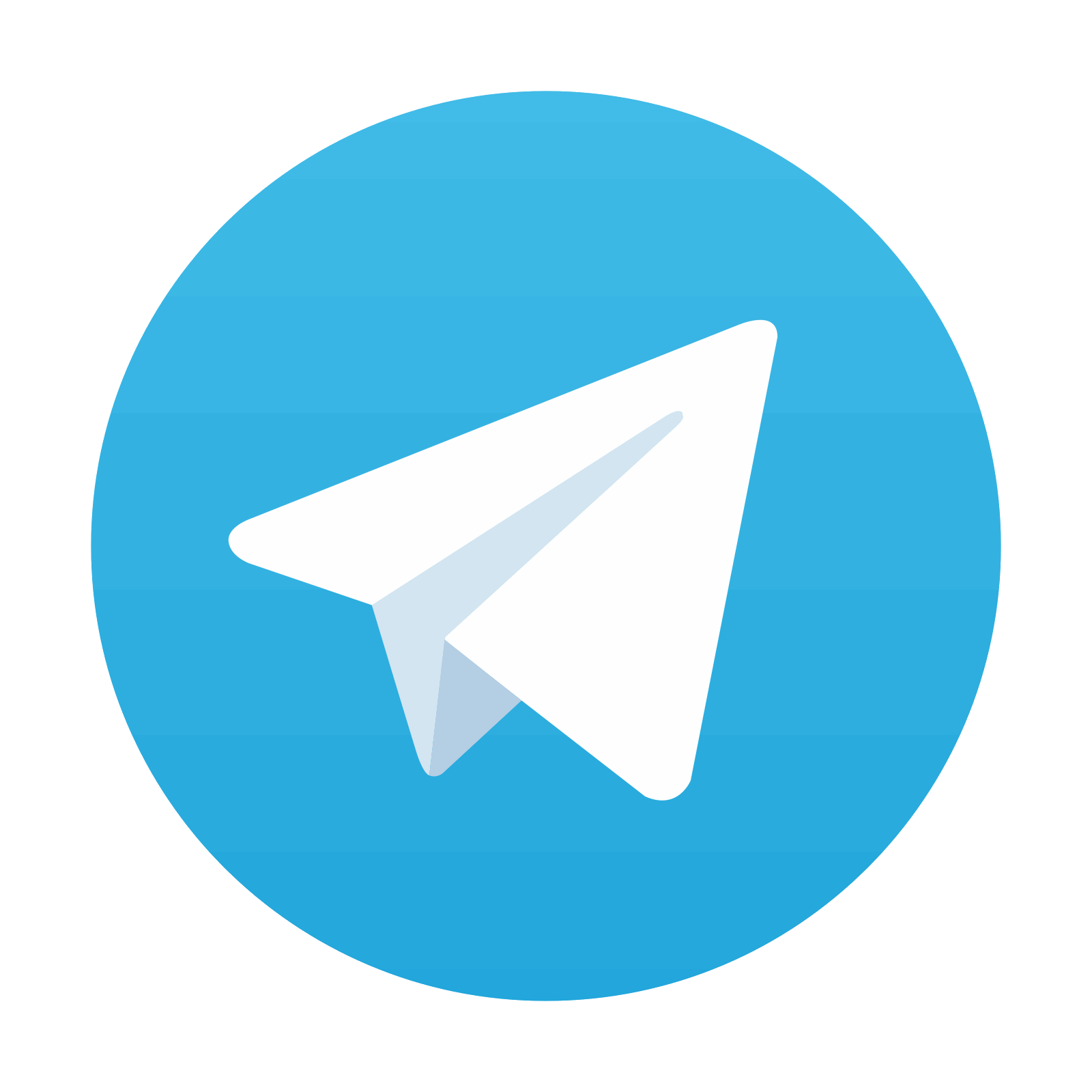
Stay updated, free articles. Join our Telegram channel

Full access? Get Clinical Tree
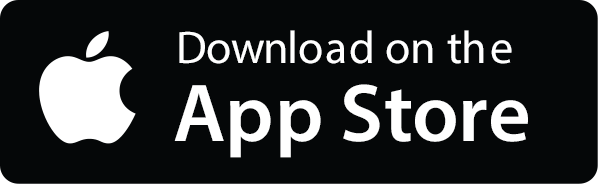
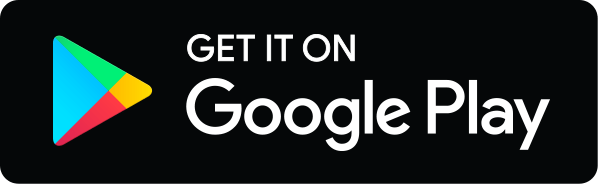