Clinical background
Key symptoms and signs
Keratoconus (KC) is a slowly progressive, noninflammatory condition in which there is central thinning of the cornea, changing it from dome-shaped to cone-shaped. KC comes from the Greek words kerato, meaning cornea, and conus, meaning cone. KC causes the cornea to become thinner centrally or inferiorly with resultant gradual bulging outward ( Figure 6.1 ).

Patients with KC initially notice blurring and distortion of vision ( Box 6.1 ). They may also complain about photophobia, glare, disturbed night vision, and headaches from eyestrain. As KC progresses, patients are increasingly myopic and astigmatism can become more irregular. KC is a bilateral condition, though usually asymmetric in severity and progression. In the early stages of the disease, KC is not visible to the naked eye. However, in the later stages of progression, the cone-shaped cornea can be visible to an observer when the patient looks down while the upper lid is raised. The pointed cornea will push the lower lid out in the area of the cone like a V-shaped dent in the lower lid. This anterior protrusion seen in the lower lid is called Munson’s sign ( Figure 6.2 ). Fleischer’s ring is a partial or complete iron deposition ring in the deep epithelium encircling the base of the KC cone. It appears yellow to dark brown in color and is best seen with the cobalt blue light at the slit lamp. Rizutti’s sign is a conical reflection on the nasal cornea when light is shined from the temporal side. Vogt’s striae may be seen in the deep stroma of the apex of the cone.
- •
Keratoconus is a slowly progressive, noninflammatory condition that involves central or inferior thinning of the cornea, changing it from dome-shaped to cone-shaped
- •
Keratoconus affects approximately 1 in 2000 people in the USA. Most cases are sporadic but approximately 6–10% have a hereditary component for this ocular disorder
- •
Keratoconus can be diagnosed by retinoscopy, keratometry, keratoscopy, and computed corneal topography
- •
Munson’s sign, Fleischer’s ring, Rizutti’s sign, and Vogt’s striae are signs of keratoconus

Historical development
KC has been recognized since the 1750s and was first carefully described and differentiated from other ectatic conditions in the 1850s. Diagnosis of KC, especially in its early forms (forme fruste KC), has been greatly improved with the development of videokeratography and algorithms which allow quantification of the topographical surface and identification of the KC phenotypes.
Epidemiology
KC is the commonest corneal dystrophy in the USA, affecting approximately 1 in 2000 people. Although KC occurs sporadically in most individuals, approximately 6–10% have a hereditary component since it is reported in multiple generations of families and identical twins. If a first-degree relative has KC then the prevalence of other family members developing KC is approximately 3.34%, which is significantly higher than the general population. It affects people of all races and both sexes, though there is a slight female preponderance.
Diagnostic workup
Corneal distortion with KC is seen on retinoscopy, keratometry, keratoscopy, and computed corneal topography ( Figure 6.3 ). There is often localized, abnormal inferior or central corneal steepening. This results in asymmetry with a large refractive power difference across the surface of the cornea. Some ophthalmologists use the inferior–superior (I-S) value when determining if KC is present on corneal topography. This measurement determines differences in corneal refractive power between inferior and superior points on the cornea and may aid in determining if KC is present or may develop in the future.

Gene array analyses of KC corneas have demonstrated altered levels of alpha-enolase, beta-actin, aquaporin 5, and desmoglein 3, some of which have been proposed as molecular markers for KC. However, at the present time markers are not used routinely in clinical practice for diagnosis.
Treatment
Management of KC usually begins with spectacle correction, if possible. When eye glasses can no longer correct the condition as the astigmatism worsens, specially fitted contact lenses can often reduce the distortion from the irregular shape of the cornea ( Box 6.2 ). Finding a KC contact lens specialist is important as frequent contact lens changes and checkups are usually required for good visual results. In advanced KC, when good vision can no longer be attained with contact lenses and/or the patient is intolerant of contact lens wear, penetrating keratoplasty is usually recommended. Approximately 10–20% of KC patients eventually require penetrating keratoplasty, and the success rate is greater than 90%, one of the highest for corneal transplantation. A new, major advancement in penetrating keratoplasty involves the use of the femtosecond laser to make the cuts in the donor and recipient corneas so that the fit between the two is more precise. This new development has shown great promise for penetrating keratoplasty for KC where there is improved wound healing, faster visual recovery, and quicker removal of sutures postoperatively. Another relatively new treatment option is the placement of intracorneal polymethyl methacrylate (PMMA) segments (Intacs, Addition Technology) inserted into the mid-stroma of the more peripheral cornea in an attempt to flatten the cone. Some patients still require contact lenses in order to attain functional vision after placement of Intacs.
- •
Treatment for most patients includes specially fitted contact lenses
- •
Approximately 10–20% of keratoconus patients eventually require penetrating keratoplasty and the success rate is greater than 90%
- •
Recent advancements in penetrating keratoplasty involve the use of the femtosecond laser to make precise cuts in the donor and recipient corneas to improve their fit
- •
Another new treatment option is the placement of intracorneal polymethyl methacrylate (PMMA) segments (Intacs, Addition Technology) into the midstroma of the peripheral cornea in an attempt to flatten the cone
- •
Corneal collagen cross-linking is a new treatment concept which involves applying photosensitizing riboflavin (vitamin B 2 ) eye drops to the de-epithelialized cornea and then exposing the eye to ultraviolet A light
- •
Controlled trials are under way to investigate the safety and efficacy of this ultraviolet cross-linking procedure
Corneal collagen cross-linking is a new treatment concept which involves applying photosensitizing riboflavin (vitamin B 2 ) eye drops to the de-epithelialized cornea and then exposing the eye to ultraviolet A light. Researchers have found a significant increase in corneal rigidity in animal eyes following this treatment regimen. Early studies in KC patients have shown that progression of KC was halted after this cross-linking treatment. Randomized controlled trials to investigate the safety and efficacy of this treatment are under way at this time.
Prognosis and complications
KC usually has its onset during puberty, with a gradual and irregular progression over approximately 20 years. The rate of progression and severity of the condition are quite variable, ranging from mild astigmatism to severe corneal thinning, protrusion, and scarring. In advanced KC, there may be a rupture in Descemet’s membrane, causing sudden clouding of vision due to acute stromal or epithelial edema, called acute corneal hydrops ( Figure 6.4 ). Topical corticosteroid and 5% NaCl drops are usually used to treat the acute hydrops episode. This condition often resolves over weeks to months and may result in central corneal scarring or flattening.

Pathology
Loss of Bowman’s layer and stromal thinning
A hallmark histological feature of the KC cornea is focal regions where the Bowman’s layer is absent and the epithelial cells are in direct contact with the underlying stroma ( Figure 6.5 ). These sites also show decreased levels of fibronectin, laminin, entactin, type IV collagen, and type XII collagen ( Box 6.3 ). In areas of active disease, the stromal extracellular matrix (ECM) demonstrates elevated levels of type III collagen, tenascin-C, fibrillin-1, and keratocan, but many of these changes are nonspecific and can also be found in general wound-healing processes. Most interestingly, the ECM abnormalities in KC corneas are not uniform. The corneal stroma can lose more than half its normal thickness and have deposits of fibrotic ECM while in an adjacent, thicker region the matrix patterns are normal. In addition, there is variability in the epithelial thickness, with some areas having only 1–3 cell layers and other regions appearing completely normal.

- •
Keratoconus corneas have disruption in Bowman’s layer which allows the epithelial cells to be in direct contact with the underlying stroma
- •
Keratoconus corneas have increased levels of apoptosis found in anterior stromal keratocytes, epithelial, and endothelial cells
KC corneas are unusually thin and pliable. Biochemical studies reported decreased total protein and sulfated proteoglycan levels, normal collagen cross-linking, and variable total collagen content. Recent studies showed that stromal lamellar slippage may contribute to the thinning and anterior protrusion of KC corneas.
Apoptosis in keratoconus
Apoptosis is the process by which cells undergo an organized, programmed cell death. KC corneas have increased levels of apoptosis associated with the anterior stromal keratocytes, epithelial cells, and endothelial cells ( Figure 6.5 ). Erie and coworkers showed an even greater decline in keratocyte density in KC patients using contact lenses. The KC corneas have elevated levels of leukocyte common antigen-related protein (LAR), a transmembrane phosphotyrosine phosphatase that stimulates apoptosis, and cathepsins G, B, and V/L2, which represent a caspase-independent pathway for apoptosis. Cathepsins mediate apoptosis by triggering mitochondrial dysfunction, cleaving Bid and releasing cytochrome c. Furthermore, KC corneas have decreased levels of tissue inhibitors of metalloproteases, TIMP-1 and TIMP-3, which can modulate apoptosis. Finally, the moderate to severe atopy and vigorous eye rubbing often found in KC patients may contribute to apoptosis since studies showed that chronic, repetitive injury to the corneal epithelium stimulates anterior stromal cell apoptosis.
Enzyme activities in human corneas
It is generally accepted that KC stromal thinning is associated with increased activities in ECM-degrading enzymes. In the early 1960s it was noted that KC corneas had degraded epithelial basement membranes and increased gelatinase activities. It was subsequently demonstrated that KC corneas have increased levels of lysosomal enzymes (acid esterase, acid phosphatase, acidic lipase), cathepsins G, B, and VL2, matrix metalloproteinase-2 (MMP-2) and MT1-MMP (MMP-14) which can degrade many forms of ECM. Moreover, many of the naturally occurring inhibitors for those enzyme families are found in lower levels. In addition to corneal involvement, the conjunctiva of KC patients shows increased lysosomal enzyme activities.
A major corneal function is to eliminate the reactive oxygen/nitrogen species (ROS/RNS) and aldehydes that are generated by ultraviolet light. For this purpose, the cornea possesses numerous antioxidant enzymes such as superoxide dismutases (SODs), catalase, aldehyde dehydrogenases (ALDH 3 A1), glutathione reductase, glutathione S -transferase, and glutathione peroxidase ( Figure 6.6 ). When ROS/RNS are not eliminated, they can react with other molecules and form cytotoxic aldehydes and peroxynitrites. These antioxidant enzyme activities change with aging and in response to cytokines and growth factors and this can increase the susceptibility to oxidative damage. Many of the antioxidant enzymes of the lipid peroxidation and nitric oxide pathways are abnormal, suggesting their involvement in KC pathology.

Etiology
Environmental risk factors for keratoconus
Numerous studies report an association between KC and atopy, which includes asthma, eczema, and hayfever ( Box 6.4 ). Recently Kaya and coworkers provided evidence that KC patients with full or partial atopy have unique topographical and pachymetric characteristics compared to KC patients without atopy. However, some suggest that it is not so much that atopy per se leads to KC but it is the associated vigorous eye rubbing which contributes to the development of KC. Many case reports exist in the literature describing persistent, vigorous eye rubbing and development of KC in children, patients with Down syndrome, and even adults with unilateral KC. The Collaborative Longitudinal Evaluation of Keratoconus (CLEK) study also suggests that asymmetry in corneal curvature may be related to vigorous, unilateral eye rubbing that occurs in some KC patients. It is likely that KC is influenced by both environmental factors and some genetic component.
- •
Associations between keratoconus and atopy, which includes asthma, eczema, and hayfever, have been described
- •
Vigorous eye rubbing may contribute to the development of keratoconus
- •
Patients with trisomy 21 (Down syndrome) have a high incidence of keratoconus
- •
Keratoconus has an autosomal-dominant inheritance with variable penetration
- •
At least 10 different chromosomes are linked to keratoconus
- •
The genetics of keratoconus are complex and involve multiple genes
Genetic risk factors for keratoconus
KC can be found in 0.5–15% of trisomy 21 (Down syndrome) patients and is less frequently associated with Ehlers–Danlos syndrome and osteogenesis imperfecta. Case reports show KC patients also having other ocular diseases such as Leber’s congenital amaurosis, cataracts, granular corneal dystrophy, Avellino corneal dystrophy, and posterior polymorphous dystrophy. However, the vast majority of KC patients do not have other ocular or systemic diseases.
Rabinowitz et al showed that KC has an autosomal-dominant inheritance with variable penetration. At the present time, 10 different chromosomes have been linked to KC (21, 20q12, 20p11-q11, 18p, 17, 16q, 15q, 13, 5q14.3-q21.1, 3p14-q13, 2p24) but at least 50 candidate genes have been excluded as playing a role in development of KC. A Japanese study showed that three human leukocyte antigens (HLA-A26, B40, and DR9) were associated with early-onset KC. A defect in the SOD1 gene on chromosome 21 has also been linked to KC. It is controversial as to whether the homeobox gene VSX1 is associated with KC. Novel mutations of VSX1 were reported in a patient with both KC and posterior polymorphism dystrophy and in a series of individual KC patients. However, another study reported a single nondisease-causing polymorphism of Asp144Glu and concluded that the VSX1 gene lacked association with KC. The expression of VSX1 occurs during wound healing as myofibroblasts differentiate and may play a role in abnormal stromal repair processes.
The genetics of KC are complex and involve multiple genes. As seen in other diseases, the general KC phenotype may result from defects in a variety of genes that are all related to a final common pathway. Further investigations will be required to clarify the contributions of the genetic and environmental components to the development and progression of KC.
Pathophysiology
The biological basis of oxidative damage in keratoconus corneas
KC corneas have numerous signs of oxidative damage ( Table 6.1 and Box 6.5 ) with increased levels of cytotoxic aldehydes from the lipid peroxidation pathway, ROS (superoxides, hydrogen peroxide, and hydroxyl radicals) and RNS (nitric oxide and peroxynitrite) ( Figure 6.6 ). These elements can alter cellular structure and function by reacting with the proteins, DNA, and lipids ( Figure 6.7 ).
|
- •
Keratoconus corneas are defective in their ability to process and eliminate reactive oxygen/nitrogen species, which causes oxidative damage
- •
A number of antioxidant enzymes are abnormal in keratoconus corneas
- •
Oxidative elements can alter cellular structure and function by reacting with the proteins, DNA, and lipids
- •
The cultured keratoconus fibroblasts demonstrate inherent, hypersensitive responses to oxidative stressors
- •
Keratoconus involves multiple molecular and biochemical events, all related to a “final common pathway” that yields the keratoconus phenotype
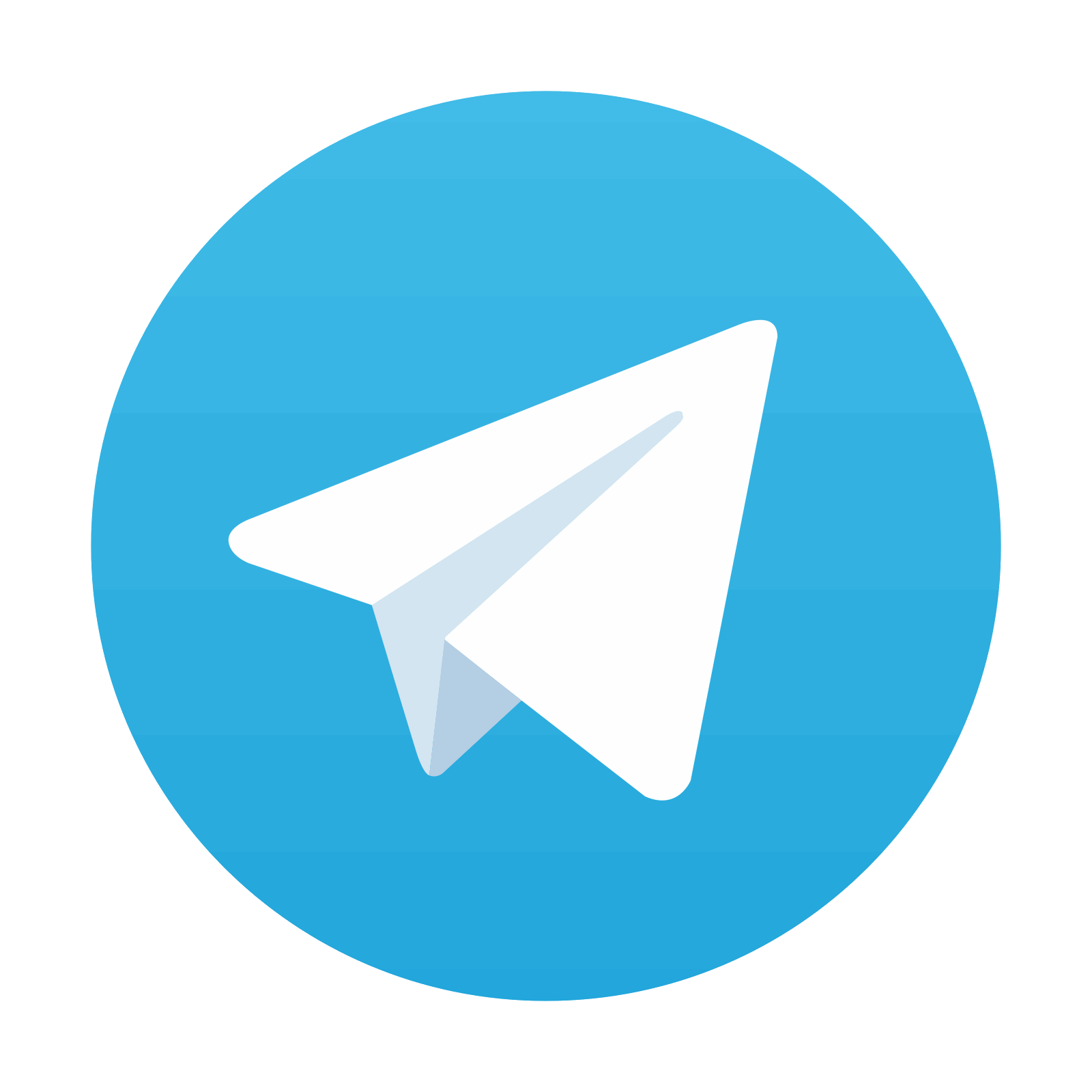
Stay updated, free articles. Join our Telegram channel

Full access? Get Clinical Tree
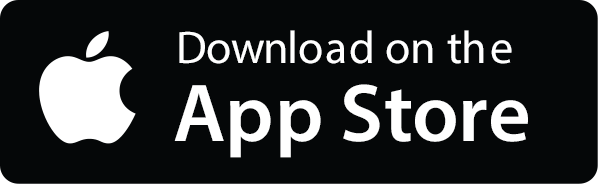
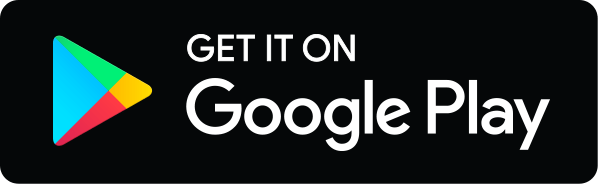
