Clinical background
Classification
From a traditional clinical perspective, amblyopia is defined as a loss of visual acuity of three lines or more on a clinical letter chart that is not optically correctable and is not due to an ophthalmoscopically observable pathological cause. Amblyopia is classified by its underlying etiologic association with one or more of the following: strabismus, anisometropia, or form deprivation. Approximately one-third of amblyopes have a strabismus (eye deviation), one-third have anisometropia (unequal refractive error), and one-third have a mixture of the two. Deprivation amblyopia is rare (incidence 0.1%).
Initially amblyopia was conceptualized as a single condition resulting from one of these three types of disruption to normal visual development ( Figure 58.1 ). Since then two more complicated classifications have been suggested, one based on the detailed properties of the behavioral deficit in small samples of amblyopes that suggested a classification in terms of the presence or absence of a strabismus and the other based on a few summary measures conducted on a large sample of around 400 amblyopes, suggesting a classification based on whether binocular function has been preserved. According to the summary measures of McKee et al, only around 10% of strabismics have binocular function as opposed to 80% of anisometropes, so these two different dichotomies are very similar. However, what is neglected by all these classifications is that there is considerable variation from patient to patient even within one class. Amblyopia can be associated with disrupted vision at a variety of different ages and it is known from animal models that there are different critical periods for different visual functions.

Even if one were to consider amblyopia simply in terms of a monocular visual loss (which would be misleading, as argued above) it is still much more than simply a loss in visual acuity. Amblyopia is a complex syndrome in which many seemingly unrelated visual functions are impaired. Letters presented in a row with other letters adjacent are much harder to discriminate than isolated letters, a phenomenon known as crowding. Subtle spatial distortions are also a part of an amblyope’s perception: the edges of letters close to the fovea are less blurred and simple repetitive patterns with a fine structure (i.e., high spatial frequencies) are seen to be distorted within central vision. Further anomalies for spatial and motion processing are detailed below. In addition, under normal binocular viewing conditions, the two eyes of an amblyope do not work together correctly. Therefore, with both eyes open amblyopes are effectively monocular as most of the information seen by the fellow amblyopic eye is suppressed. The site and nature of this suppressive mechanism, thought to be cortical, are largely unknown but its importance, from both etiologic and treatment perspectives, is immense. Indeed, the visual deficit in the amblyopic eye that occurs under binocular viewing may include several components, including full monocular amblyopia, reduced peripheral function of the amblyopic eye’s visual field in a region corresponding to the fovea of the fellow fixing eye (anomalous retinal correspondence owing to the strabismus), and suppression or masking from the fellow fixing eye ( Box 58.1 ).
- •
Monocular amblyopia
- •
Reduced peripheral function suppression
Treatment
The traditional treatment for amblyopia has been to occlude the good eye with the intention of forcing the amblyopic eye to function. If the child has a significant amount of hyperopia, atropine penalization can be used to blur the good eye by paralyzing its accommodation. In the case of emmetropia, an opaque occluder can be worn in front of the good eye. In the late 1980s it was generally accepted that such occlusion had to be complete and fulltime for it to be effective. This produced great psychological and social hardship for young children, most of whom were of school age, and consequently the compliance was never what it should have been. Owing to a number of innovative treatment approaches, it is now generally accepted that part time occlusion, if associated with an intensive, attention-demanding task, can be just as successful as fulltime occlusion with none of the associated psychosocial side-effects. The recovery of vision is believed to be age-dependent, with little recovery possible from occlusion beyond 12 years, but see PEDIG. However, active training regimes based on the principle of perceptual learning have recently been shown to be effective in restoring at least some visual function in adult amblyopes.
Interestingly, the rationale behind both the standard treatment for amblyopia and perceptual training approaches is that amblyopia is a monocular problem and, until the monocular function of the amblyopic eye is restored, one cannot expect the two eyes to work together in a binocular and/or stereoscopic sense. What has been ignored is that the monocular loss could be the consequence of disrupted binocular mechanisms that have to be rectified prior to monocular recovery and that the amblyopia per se may not be the limiting impediment to binocular function. Accordingly, any therapeutic approach should involve binocular stimulation and an attempt to reduce not only the monocular acuity loss, but also any additional suppressive influences exerted by the good eye on the amblyopic eye. Recent results suggesting that a reduction of intracortical inhibition in animals deprived of vision early in life leads to acuity improvement further highlight the importance of cortical suppressive mechanisms ( Box 58.2 ).
- •
Loss of binocular connections
- •
Interocular suppression
Laboratory perspective
Psychophysics
One observation that has stood the test of time is that amblyopes require more contrast to detect stimuli with their amblyopic eyes, particularly those at higher spatial frequencies. Peak contrast sensitivity is often reduced to some extent but contrast sensitivity at higher spatial frequencies shows a more pronounced reduction. Unfortunately this has little diagnostic utility because similar losses are seen for all types of amblyopes as well as for many different types of optical, retinal, and cortical pathology. It does, however, provide a more sensitive way of monitoring recovery from treatment than the more conventional acuity measures simply because of the steeper than unity slope of the contrast sensitivity fall off. On log/log axes this is usually between 3 and 4, giving a corresponding contrast sensitivity magnification.
The way that the contrast deficit is distributed across the visual field has some diagnostic value and suggests an important difference in the underlying mechanisms responsible for strabismic and nonstrabismic amblyopia. The contrast sensitivity deficit is confined to central vision for strabismics, whereas it is more evenly distributed across the visual field in nonstrabismic anisometropes ( Figure 58.2 ). This provides an explanation for another curious finding, that the contrast sensitivities of the two eyes of a strabismic amblyope normalize (i.e., are equated in sensitivity) under conditions of low light levels whereas those of nonstrabismic anisometropes do not.

At suprathreshold levels, contrast is perceived normally by the amblyopic eye, as the deficit is confined to threshold. Again strabismics and nonstrabismic amblyopes show subtle differences in the way their visual systems go from obvious contrast deficits at threshold to normal contrast perception above threshold. For strabismics, this transition is abrupt, whereas for nonstrabismic anisometropes it is gradual. For suprathreshold conditions, even though contrast is perceived normally, visual perception is quite abnormal for amblyopes for two other, possibly related, reasons that seem to have nothing to do with the aforementioned threshold deficit. The first of these reasons involves spatial distortions that mainly affect central vision and high spatial frequencies. These distortions predominantly affect strabismic amblyopes. Their basis is not well understood but their presence has led to the suggestion that there may be a disruption to the retinotopic map provided by the amblyopic eye input ( Box 58.3 ). Another suggestion is that spatial distortions are a result of anomalous interactions between cells with different orientation preferences subserved by the amblyopic eye. A possibly related finding is that strabismic amblyopes are more uncertain of the spatial position of objects when using their amblyopic eye. Nonstrabismic anisometropes do not have such a deficit. This has nothing to do with the poorer vision of the strabismic eye and it shows the unexpected property (i.e., compared with the contrast threshold deficit) of being spatial scale-invariant. What this means is that the positional uncertainly of large objects is just as pronounced as that for small objects. This is shown in Figure 58.3 (left panel) where spatial accuracy in a three-element alignment task for the fixing eye is compared to that of the fellow amblyopic eye for stimuli of a number of spatial scales (i.e., sizes). Sensitivity for both eyes varies with spatial scale, being better at finer spatial scales. Importantly, the deficit in amblyopia is similar for large and fine scales. Therefore a deficit in spatial accuracy does not provide a good explanation for spatial distortion since the former is spatial scale-invariant and the latter is not. Apart from an inaccuracy there is also, in most cases, a standing distortion (measured as a bias in alignment tasks) that may well relate to the perceived distortions. A number of attempts have been made to make this connection; both deficits could reflect different aspects of the fidelity of the retinotopic map. Animals with amblyopia secondary to a strabismus or lid suture experience exactly the same scale-invariant positional uncertainty as found in humans. The size (often an order of magnitude or more) and spatial scale invariance of this deficit are shown in Figure 58.3 (middle and right panel). The fact that such an anomaly occurs not only in cases of strabismus but also as a consequence of lid suture suggests that a basic, but hitherto unknown, aspect of visual development has been disrupted, rather than it simply being the consequence of an adaptation to the strabismus, such as anomalous retinal correspondence.
- •
Reduced contrast sensitivity for small objects
- •
Positional uncertainty for all sized objects

So far we have only considered the detection or appearance of stimuli in local regions of the visual field, regions small enough that they could be potentially explained in terms of single cells in V1. There are also reported deficits in amblyopia involving the processing of stimuli across much larger regions of visual space. Such tasks are considered to be “global” if information in very different regions of space has to be interrelated in some way. Cells with sufficiently large receptive fields and the processing properties required to accomplish the combination of information across space are found in the ventral and dorsal streams of the extrastriate cortex. Although local motion sensitivity (i.e., V1) is thought to be normal in amblyopia (but see Ho and Giaschi ), global motion sensitivity has been shown to be anomalous in both eyes of strabismic amblyopes. The form of the deficit suggests it is not due to the visibility problem (i.e., V1) but to the “global processing” of local motion thought to occur in the dorsal stream of the extrastriate cortex. Optic flow is also affected, suggesting a site beyond middle temporal area along the dorsal processing stream. A similar deficit occurs for global form processing, suggesting an extrastriate deficit along the ventral processing stream. There are two processes involved in the global processing of information, one involving integration and the other differentiation. The former involves the utilization of local signals distributed in different parts of the stimulus field, whereas the latter involves the segregation of signal from noise in the same parts of the field. Both operations must occur together in global signal/noise tasks (i.e., coherence measures) of the type that have revealed deficits in amblyopic processing. In similar tasks without noise where only signal integration is required, amblyopes perform normally on both a global form and global motion tasks. Since it is the introduction of noise to a global task that results in reduced performance for amblyopes, one is led to believe that signal/noise segregation is the main problem rather than signal integration per se. Until we know more about the physiological basis for this important operation in normal vision, it is premature to discuss its physiological basis in amblyopia. However, a starting point would be the suppressive surrounds that have been shown to be an important part of extrastriate receptive fields (e.g., MT) and thought to play a role in signal/noise segregation.
There are also suggestions that the deficits in amblyopia extend to visual cognition ( Box 58.4 ). It has been shown that numerosity judgments are deficient, that higher-order motion is defective, and that the perception of faces is impaired.
- •
V1 (contrast sensitivity and positional uncertainty)
- •
Dorsal and ventral extrastriate cortex (global motion and form)
Models
Models of visual loss in amblyopia are not well developed and tend to apply only to separate aspects of the dysfunction. They have tried to explain the contrast sensitivity deficit and the binocular vision deficit in terms of the function of single cells in area V1. None has been completely successful. For example, the loss of contrast sensitivity in amblyopia that affects mainly high spatial frequencies and central vision, at least for strabismics, is thought to be located in area V1 because some centrally located neurons display reduced contrast sensitivity and anomalous spatial properties. The fact that there is such variability from animal to animal and that the magnitude of the single-cell deficit is not enough to explain adequately the full extent of the behavioral deficit has led people to think that there is more to the story than is gleaned from the responses of single cells in V1. In strabismic animals with a mild to moderate amblyopia there do not appear to be fewer neurons subserving the amblyopic eye, but some of these neurons do show a reduced sensitivity. In strabismic animals where the amblyopia is severe, there is a suggestion that fewer neurons (i.e., as determined by the encounter rate) might subserve amblyopic function. What is generally agreed is that fewer neurons appear to have binocular connections in amblyopic animals.
The binocular vision deficit in amblyopia has traditionally been thought to be the result of loss of binocular connections in area V1. However, a recent study has shown that binocular summation can be normal in strabismic amblyopes once the sensitivity difference between their eyes is taken into account, suggesting that substantial binocular connections may be intact. It remains a possibility that the connections at the single-cell level are in fact intact and that this can only be revealed by first equating the contrast of the inputs to the two eyes, or observing the influence of one eye’s input on the other, rather than the response from each eye alone. Another important aspect of the physiology is that strabismic animals display interocular inhibitory interactions that may underlie the reduced binocular function in strabismic amblyopia and which may be amenable to treatment. There is good evidence that binocular function, including stereo, can be restored in strabismic and anisometropic amblyopes under suitable treatment regimes. The key issue for the future is to understand the nature of these suppressive interactions that prevent normal binocular and stereoscopic function in amblyopia.
The positional uncertainty deficit that is an important feature of the vision of strabismic amblyopes ( Figure 58.3 ) has been explained in two related ways. Levi and Klein suggested that there were fewer cells driven by the amblyopic eye and as a consequence of the less than adequate sampling of space, positional accuracy is reduced ( Figure 58.4B ). The scale invariance of the deficit ( Figure 58.3 ) would then imply that this undersampling occurs equally at all scales. The other proposal ( Figure 58.4A ) is that there is an uncalibrated neural disarray, implying not fewer cells, but rather a disarray in the retinotopic map. The idea is that at birth our visual systems are broadly accurate in their retinotopy but during visual experience this is refined or calibrated by functional Hebbian rules. A strabismus disrupts this calibration and results in a positionally uncalibrated cortex for the input from the deviated eye. While both proposals are able to explain the inaccuracy problem, the disarray hypothesis can account for the fixed spatial distortions reported by amblyopes. The physiology suggests that there are a number of explanations including a loss of cells, modified cellular properties, and aberrant nerve connections.

The global motion and global form deficits in amblyopia have been investigated more extensively psychophysically. It has been proposed that the deficits are not due to poor contrast sensitivity or indeed poor local motion or local orientation discrimination, as might occur in area V1, but rather are due to impaired segregation of signal and noise, a property of extrastriate cortex.
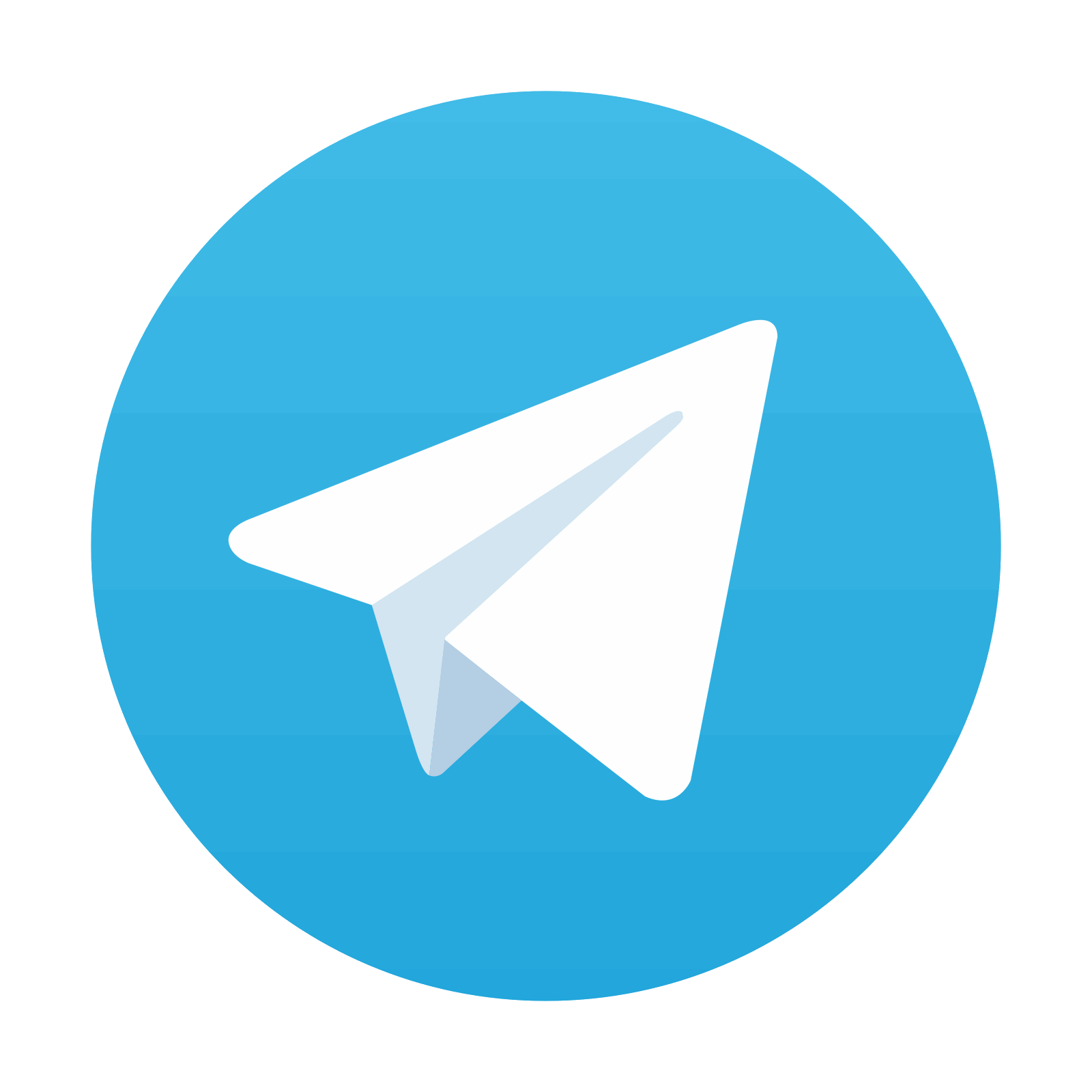
Stay updated, free articles. Join our Telegram channel

Full access? Get Clinical Tree
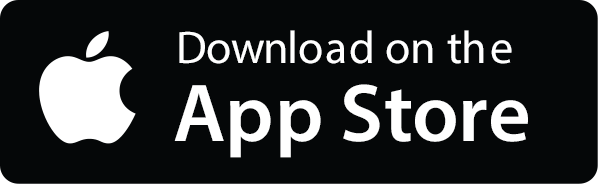
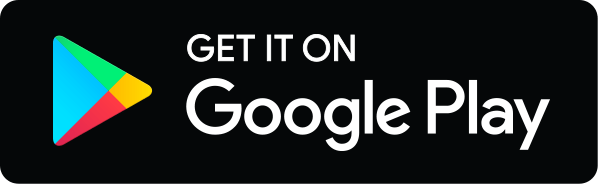