Clinical background
The term retinitis pigmentosa (RP) is used to describe a group of inherited disorders in which vision loss is caused by degeneration of the rod and cone photoreceptor cells of the retina. RP occurs in nonsyndromic and syndromic forms. It has recently been recognized that in many of the syndromic disorders of which RP is a part, the common link between the affected tissues is cilia, as the light-sensitive outer segments of photoreceptor cells are specialized sensory cilia ( Figure 74.1 ).

Symptoms and signs
In all types of RP, nyctalopia (night blindness) is often the first symptom noticed. This is due to dysfunction and death of rod photoreceptor cells, which mediate vision under conditions of dim illumination. This symptom is often noticed in adolescence, but the age of onset can be variable, ranging from early childhood to adulthood. Loss of peripheral visual field follows, and is progressive, resulting in constriction of visual fields. Central vision, mediated predominantly by cone photoreceptor cells, is ultimately lost in many cases as well, often in later adulthood.
The progression of visual symptoms is associated with dysfunction and death of photoreceptor cells and consequent changes in the retinal pigment epithelium, which is visible on fundus exam. For example, in childhood, the fundus may appear relatively healthy, or small regions of depigmentation may be noted prior to the detection of the typical bone spicule pigmentation in the midperiphery ( Figure 74.2A ). As more photoreceptor cells die, and visual field is lost, fundus abnormalities become more notable, with more prominent bone spicule pigmentation and associated retinal atrophy ( Figure 74.2B ). Eventually, attenuation of retinal blood vessels and optic atrophy are evident, as further loss of photoreceptor cells and secondary loss of retinal ganglion cells occurs ( Box 74.1 ).

Other degenerations
In addition to RP, many other inherited retinal degenerations have been described. These have been classified clinically by their age of onset, the types of photoreceptor cells affected, the region of the retina involved, and rates of progression. Leber congenital amaurosis (LCA) is a severe early-onset form of retinal degeneration, in which poor vision associated with nystagmus is evident early in childhood. Cone and cone–rod dystrophies are characterized by early-onset cone dysfunction, in contrast to RP, in which rods are typically affected first. Congenital stationary night blindness is characterized by early-onset night -blindness like RP, but has a more stable clinical course.
Epidemiology
The prevalence of RP in the USA, Europe, and Japan is approximately 1 in 4000. This translates into ∼1.5 million individuals affected with RP worldwide. Data from the Beijing Eye Study suggest that the prevalence in China may be higher, at approximately 1 in 1000. These data predict approximately 1.3 million people affected with RP in China alone, although this estimate is based on a relatively small sample size compared to the total Chinese population. Data from studies in Japan and Denmark and Kuwait indicate that RP is among the leading causes of blindness or visual impairment, especially in working-aged people, accounting for 25–29% cases in that age group (21–60 years).
Diagnostic workup
Clinical evaluation of a patient with symptoms of RP, such as nyctalopia and decreased visual fields, involves thorough ophthalmic examination, testing of visual function, consideration of systemic evaluations, and genetic testing. Visual acuity may remain normal even in later stages of classic RP, in which rod photoreceptors are affected first. Early loss of central acuity suggests the possibility of early cone photoreceptor dysfunction. Anterior-segment exam is important to rule out other causes of vision loss, and to look for posterior subcapsular cataracts, which develop in up to 50% of patients with RP.
Visual field testing is important both for detecting field loss for diagnostic purposes, and for following disease status over time. Full-field evaluations using a Goldmann perimeter or Humphrey field analyzer are useful for detecting the midperipheral scotomas typically observed in patients with RP. Progression of field loss is associated with loss of rod and cone photoreceptor function, resulting in small residual islands of vision.
Electroretinography
Electroretinogram (ERG) testing is an important diagnostic tool for patients with RP. The ERG measures the function of rod and cone photoreceptors. It is a measure of the field potential generated by the circulating ion currents in photoreceptor cells. Standards for the performance of ERGs have been established by the International Society for Clinical Electrophysiology of Vision (ISCEV). In the ISCEV standard ERG evaluation, five steps are used to evaluate rod and then cone function: the rod ERG, the combined rod–cone ERG, oscillatory potentials, single flash cone ERG, and 30-Hz flicker ERG. Responses to low levels of white or blue light in dark-adapted subjects are used to evaluate rod photoreceptor function. A brighter standard flash of white light is then used to elicit a maximal or combined response from both the rod and cone photoreceptors. A typical response includes a negatively deflected a -wave, followed by a positive b -wave ( Figure 74.3 ). The a -wave is a measure of the photoreceptor response; the b -wave is thought to be generated by cells in the inner retina.

Following light adaptation, cone responses to a single white flash are recorded in the presence of background illumination. Finally, flicker ERGs recorded at approximately 30 flashes per second (30 Hz) are used to measure responses from cones; rod photoreceptors cannot recover rapidly enough to respond to the rapid flashes ( Figure 74.4 ).

In patients with RP, ERG responses are decreased. Indeed, decreased photoreceptor function can be detected by ERG in children who remain asymptomatic until young adulthood. In RP, decreased rod photoreceptor responses are typically noted first, followed by decreases in cone responses. A typical young adult with RP will have reduced amplitudes of both rod and cone responses, and delays in the response times ( Figures 74.3 and 74.4 ). Patients with more severe retinal degeneration, such as early-onset forms of RP or LCA, may have nondetectable ERG responses ( Figures 74.3 and 74.4 ).
ERG amplitudes can provide objective measures of retinal function, and thus are useful for accurate diagnosis and for tracking the course of disease. It has also been suggested that the amplitudes of the 30-Hz cone response can be used to provide information about visual prognosis. The dark adaptation threshold can also be a useful assessment of rod photoreceptor function. This test measures the lowest intensity of white light that can be perceived in a dark- adapted state. Optical coherence tomography (OCT) can be useful for monitoring the thickness of the retina in patients with RP.
Genetic testing
Genetic testing to identify the mutations which cause an individual patient’s disease has become an important part of clinical care of patients with RP and related disorders. This is important because it can help confirm the diagnosis, assist with family planning, and provide more detailed information about the prognosis of the specific form of RP identified. Genetic diagnoses will also be increasingly important as genetic treatments for RP and related disorders are developed.
Clinical genetic testing for RP is improving, and several clinical labs now provide relevant testing. An up-to-date list can be found at www.genetests.org . Identification of pathogenic mutations in patients with RP can be challenging, due in part to the polygenic nature of these disorders (see below). New developments with high-throughput mutation detection and sequencing will hopefully simplify this process in the relatively near future.
Systemic evaluation
As discussed below, RP and related retinal degenerations are often associated with systemic disorders. It is therefore important to consider potential disease associations when evaluating patients with RP. This is especially true for children. Common systemic associations include defects in other sensory or primary cilia, such as hearing loss in Usher syndrome, cystic renal disease in Alstrom, Bardet–Biedl, Joubert, and Senior Loken syndromes. Retinal degeneration can also occur in the setting of mitochondrial disease, and metabolic disorders. Three specific forms of RP that are important to consider in children are Refsum’s disease (phytanic acid oxidase deficiency), Bassen–Kornzweig syndrome (abetalipoproteinemia), and RP with ataxia caused by α-tocopherol transport protein deficiency, as early intervention can be beneficial in these disorders.
Differential diagnosis
Other retinal degenerative disorders can present with visual symptoms like those caused by RP. The fundus appearance in gyrate atrophy is distinct from that of RP, with patches of choroidal and retinal atrophy in the midperiphery. Plasma ornithine levels are elevated in this disease, which is caused by deficiency of the ornithine aminotransferase ( OAT ) gene. Fundus appearance is also helpful for distinguishing choroideremia from RP. In this X-linked condition, RPE and choroidal atrophy are evident on fundus exam.
Treatment
Several studies have been performed to assess the value of nutritional supplements for patients with RP. A randomized clinical trial demonstrated that vitamin A supplementation slowed the decline of photoreceptor cell loss as measured by 30-Hz cone ERG amplitude in patients with RP. Vitamin A supplementation was also associated with slower loss of visual field in the subset of trial patients who performed the visual field tests with the greatest precision. Studies of dietary supplementation with the omega-3 fatty acid docosahexaenoic acid (DHA), which is present at relatively high levels in photoreceptor outer-segment membranes, did not show a clear benefit.
Several promising treatments for RP and related disorders are nearing or in clinical trials. The value of sustained intraocular release of the neurotrophic factor ciliary neurotrophic factor (CNTF) is being evaluated in a phase II trial, after being found to be safe in a small phase I study. Gene augmentation therapy for specific forms of RP, LCA, and related disorders has shown promise in preclinical and early phase I research studies. Based on these results, further clinical trials of gene therapy for LCA2, caused by mutations in the RPE65 gene, are currently in progress in England and the USA. RNA interference-mediated knockdown of mutant alleles that cause disease via dominant negative mechanisms is also being evaluated. Several approaches to using stem cells for the treatment of inherited retinal degenerations have also shown promise, including the use of RPE-like cells derived from human embryonic stem cells.
Pathology
Several clinicopathologic studies of the pathology of RP have been reported. These studies show that loss of vision in end-stage RP correlates with widespread loss of photoreceptors. In samples from patients with retained vision, a single layer of cones with shortened outer segments was observed in the macula, reflecting the slower loss in cones in most types of RP. Bone spicule pigmentation is caused by migration of RPE cells into the retina, where they cluster around blood vessels. Pathologic studies also show that the neural retina undergoes significant remodeling in human RP. Loss of photoreceptors, especially cones, appears to trigger retinal remodeling, with changes in cell organization and connections. These include neuronal and glial migration, rewiring of retinal circuits with elaboration of new neurites and synapses, and glial hypertrophy. These remodeling changes may affect the success of potential therapeutic strategies for RP and related disorders.
Etiology
Genetics of RP and related disorders
Nonsyndromic RP
RP and related disorders are caused by mutations in genes which encode proteins that are required for photoreceptor cell function. These disorders are genetically heterogeneous, with over 80 disease genes identified to date ( Tables 74.1–74.3 ). Indeed, it has recently been pointed out that photoreceptor cells are subject to more genetic diseases than any other cell type. The website RetNet provides a curated listing of disease genes and loci for retinal degenerative disorders.
Disease category | Inheritance pattern | Total number of genes and loci | Number of identified genes |
---|---|---|---|
Nonsyndromic | |||
Cone–rod dystrophy | AD | 7 | 5 |
Cone–rod dystrophy | AR | 5 | 3 |
Leber congenital amaurosis | AR | 15 | 14 |
Retinitis pigmentosa | AD | 16 | 15 |
Retinitis pigmentosa | AR | 18 | 13 |
Retinitis pigmentosa | XL | 6 | 2 |
Ciliopathy syndromes | |||
Alstrom syndrome | AR | 1 | 1 |
Bardet–Biedl syndrome | AR | 12 | 12 |
Nephronophthisis-associated | AR | 9 | 8 |
Usher syndrome | AR | 11 | 9 |
Other syndromic disorders | |||
Lipofuscinoses | 2 | 2 | |
Mitochondrial disorders | 4 | 4 | |
Refsum disease | 4 | 4 |
Gene symbol | Protein name | % 1 | Function/mechanism of disease 2 | |
---|---|---|---|---|
adRP | ||||
RHO | Rhodopsin | 25 | Phototransduction, cilia structure | |
RP1 | Retinitis pigmentosa 1 | 5.5 | Cilia structure | |
PRPF31 | Pre-mRNA processing factor 31 | 5 | RNA splicing | |
PRPF3 | Pre-mRNA processing factor 3 | 4 | RNA splicing | |
PRPH2 | Peripherin 2 | 2.5 | Cilia structure | |
PRPF8 | Pre-mRNA processing factor 8 | 2 | RNA splicing | |
IMPDH1 | Inosine monophosphate dehydrogenase 1 | 2 | Nucleotide biosynthesis | |
NRL | Neural retina leucine zipper | 1 | Overexpression of rhodopsin | |
CRX | Cone–rod homeobox protein | 1 | Cilia structure – transcription factor | |
CA4 | Carbonic anhydrase IV | pH balance | ||
FSCN2 | Fascin 2 | Cilia structure | ||
GUCA1B | Guanylate cyclase activator 1B | Phototransduction | ||
SEMA4A | Semaphorin B | Cilia structure | ||
TOPORS | Topoisomerase I binding, arginine/serine-rich | RNA splcing | ||
RP9 | Retinitis pigmentosa 9 | RNA splicing | ||
NR2E3 | Photoreceptor-specific nuclear receptor | Cilia structure – transcription factor | ||
Unknown | 45 | |||
arRP | ||||
USH2A | Usherin | 8 | Cilia structure | |
ABCA4 | ATP-binding cassette, subfamily A member 4 | 5.6 | Visual cycle | |
CNGB1 | Cyclic nucleotide gated channel beta 1 | 4 | Phototransduction | |
PDE6B | Phosphodiesterase 6B, cGMP-specific, rod, beta | 3.5 | Phototransduction | |
PDE6A | Phosphodiesterase 6A, alpha subunit | 3.5 | Phototransduction | |
RPE65 | Retinal pigment epithelium-specific protein 65 kDa | 2 | Visual cycle | |
CNGA1 | Cyclic nucleotide gated channel alpha 1 | 1 | Phototransduction | |
CRB1 | Crumbs homolog 1 | 1 | Retinal organization | |
LRAT | Lecithin retinol acyltransferase | 1 | Visual cycle | |
MERTK | MER receptor tyrosine kinase | 1 | Retinal pigment epithelium function | |
TULP1 | Tubby-like protein 1 | 1 | Cilia structure | |
RHO | Rhodopsin | 1 | Phototransduction, cilia structure | |
RLBP1 | Retinaldehyde binding protein 1 | 1 | Visual cycle | |
CERKL | Ceramide kinase-like | 1 | Sphingolipid metabolism | |
RGR | Retinal G-protein-coupled receptor | 0.5 | Visual cycle | |
NR2E3 | Photoreceptor-specific nuclear receptor | 0.25 | Cilia structure – transcription factor | |
SAG | S-arrestin | Phototransduction | ||
NRL | Neural retina leucine zipper | Overexpression of rhodopsin | ||
RP1 | Retinitis pigmentosa 1 | Cilia structure | ||
PRCD | Progressive rod–cone degeneration | |||
PROM1 | Prominin 1 | Cilia structure | ||
Unknown | 60–70 | |||
X-linked RP | ||||
RPGR | Retinitis pigmentosa GTPase regulator | Cilia structure | ||
RP2 | XRP2 protein | |||
Unknown | 10–20 | |||
LCA | ||||
CEP290 | Centrosomal protein 290 kDa | 15 | Cilia structure | |
GUCY2D | Guanylate cyclase 2D, membrane (retina-specific) | 12 | Phototransduction failure | |
CRB1 | Crumbs homolog 1 | 10 | Retinal organization | |
IMPDH1 | Inosine monophosphate dehydrogenase 1 | 8 | Nucleotide biosynthesis | |
RPE65 | Retinal pigment epithelium-specific protein 65 kDa | 6 | Visual cycle | |
AIPL1 | Aryl hydrocarbon receptor interacting protein-like 1 | 5 | Cilia structure – chaperone | |
RPGRIP1 | Retinitis pigmentosa GTPase regulator interacting protein 1 | 4 | Cilia structure | |
RDH12 | Retinol dehydrogenase 12 ( all -trans and 9- cis ) | 3 | Visual cycle | |
LCA5 | Leber congenital amaurosis 5 | 2 | Cilia structure | |
CRX | Cone–rod homeobox protein | 1 | Cilia structure – transcription factor | |
TULP1 | Tubby-like protein 1 | 1 | Cilia structure | |
MERTK | MER receptor tyrosine kinase | RPE function | ||
LRAT | Lecithin retinol acyltransferase | Visual cycle | ||
RD3 | Retinal degeneration 3 | |||
Unknown | 20–30 |
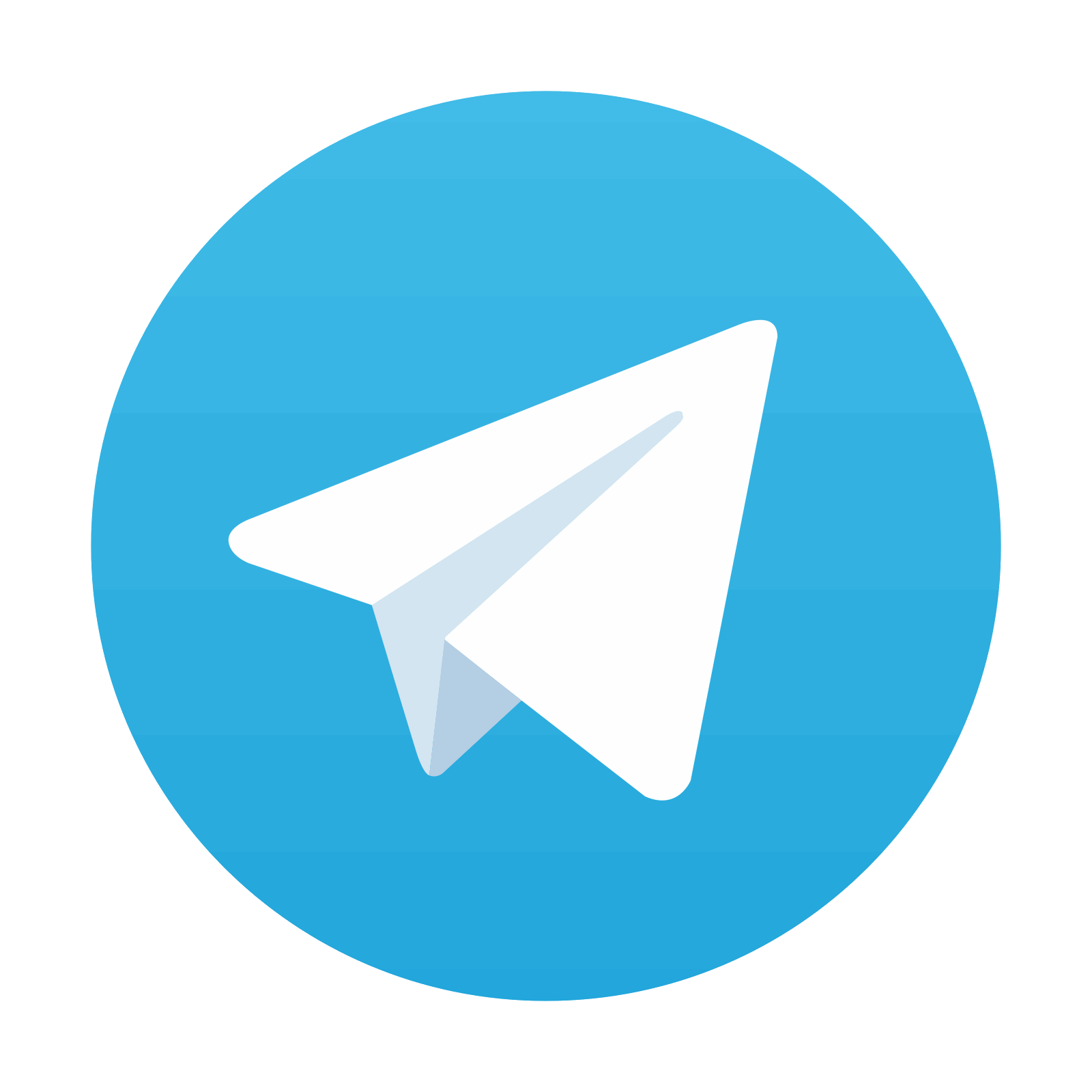
Stay updated, free articles. Join our Telegram channel

Full access? Get Clinical Tree
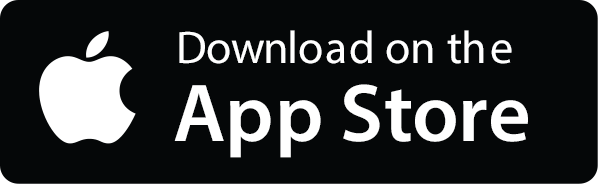
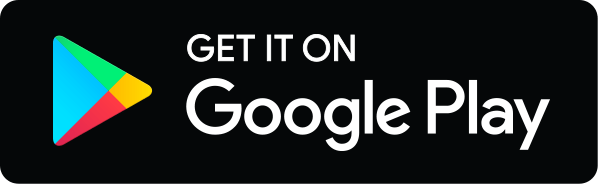
