Purpose
To determine the intra- and intervisit reproducibility of circumpapillary retinal nerve fiber layer (RNFL) measures using handheld optical coherence tomography (OCT) in sedated children.
Design
Prospective cross-sectional and longitudinal study.
Methods
Children undergoing sedation for a clinically indicated magnetic resonance imaging for an optic pathway glioma and/or neurofibromatosis type 1 (NF1) had multiple 6 × 6 mm volumes (isotropic 300 × 300 or nonisotropic 1000 × 100 samplings) acquired over the optic nerve. Children with 2 handheld OCT sessions within 6 months were included in the intervisit cohort. The intra- and intervisit coefficient of variation (CV) and intraclass correlation coefficient (ICC) were calculated for the average and anatomic quadrant circumpapillary RNFL thickness.
Results
Fifty-nine subjects (mean age 5.1 years, range 0.8–13.0 years) comprised the intravisit cohort and 29 subjects (mean age 5.7 years, range 1.8–12.7 years) contributed to the intervisit cohort. Forty-nine subjects had an optic pathway glioma and 10 subjects had NF1 without an optic pathway glioma. The CV was comparable regardless of imaging with an isotropic and nonisotropic volume in both the intra- and intervisit cohorts. The average circumpapillary RNFL demonstrated the lowest CV and highest ICC compared to the quadrants. For the intervisit cohort, the average ICC was typically higher while the CV was typically lower, but not statistically different compared to the other quadrants.
Discussion
Circumpapillary RNFL measures acquired with handheld OCT during sedation demonstrate good intra- and intervisit reproducibility. Handheld OCT has the potential to monitor progressive optic neuropathies in young children who have difficulty cooperating with traditional OCT devices.
The ability of time-domain and spectral-domain optical coherence tomography (SD OCT) measures of circumpapillary retinal nerve fiber layer (RNFL) to diagnose and monitor optic neuropathies in adults has been well established. The intra- and intervisit reproducibility of SD OCT circumpapillary RNFL measures has recently been enhanced by eye tracking and registration technology, typically yielding an intraclass correlation coefficient (ICC) greater than 90% and coefficient of variation below 4.0%. Despite the addition of eye-tracking technology, many infants, toddlers, and young children frequently cannot cooperate with traditional table-mounted SD OCT imaging owing to their young age and/or comorbid medical conditions.
The development of a handheld SD OCT has enabled pediatric practitioners to acquire high-resolution images of the circumpapillary RNFL and macula in neonates, infants, and young children. Whereas neonates and infants can be imaged while awake, the portability of the handheld OCT permits acquisition in toddlers and young children during sedation.
Handheld OCT measures of circumpapillary RNFL thickness have previously demonstrated a close relationship to vision loss (eg, visual acuity and/or visual field) in children with optic pathway gliomas. In order to interpret longitudinal changes in circumpapillary RNFL measures, the reproducibility of handheld OCT must be established. We investigated the intra- and intervisit reproducibility of handheld OCT circumpapillary RNFL measurements in sedated children being evaluated for optic pathway gliomas.
Methods
Subjects
Children undergoing a sedated magnetic resonance imaging (MRI) scan as part of their clinical care and enrolled in an ongoing longitudinal study of handheld OCT were eligible for inclusion. The longitudinal study primarily recruits children with optic pathway gliomas and those with neurofibromatosis type 1 (NF1). All subjects were recruited through the Neuro-Ophthalmology or Ophthalmology clinics at Children’s National Medical Center and received a comprehensive ophthalmologic examination. Written informed consent from the parent/guardian and written assent from the child (when applicable) was obtained before study enrollment. The study adhered to the tenets of the Declaration of Helsinki and was approved by the Institutional Review Board at Children’s National Medical Center. All data collected was Health Insurance Portability and Accountability Act (HIPAA) compliant.
The diagnosis of NF1 and NF1-related optic pathway glioma was based on standardized clinical criteria. Subjects with biopsy-proven low-grade gliomas (ie, World Health Organization grade 1 juvenile pilocytic astrocytoma or grade 2 fibrillary astrocytoma) in the absence of NF1 were considered to have a sporadic optic pathway glioma. Age-appropriate quantitative visual acuity (VA) testing was attempted on all subjects. Subjects were classified as having vision loss if they demonstrated decreased VA, defined as ≥0.2 logMAR below age-based norms, and or had visual field (VF) loss in 1 or more anatomic quadrants. Subjects who experienced vision loss from non–optic pathway glioma–related mechanisms (ie, amblyopia, papilledema, or glaucoma) were excluded.
Subjects with 2 or more acceptable handheld OCT scans (same sampling volume and eye) acquired during a single imaging session were included in the intravisit cohort. A 6 × 6 mm volume (isotropic 300 × 300 or nonisotropic 1000 × 100 samplings) was acquired over the optic nerve. Subjects could contribute scans from 1 eye for each type of sampling volume. Subjects were eligible for the intervisit cohort if they met all of the following criteria: (1) second handheld OCT imaging session within 6 months meeting the above intravisit criteria; (2) stable ophthalmologic examination (ie, no more than 0.1 logMAR decline in visual acuity and no change in their visual field); and (3) stable MRI without evidence of tumor growth. Children unable to complete quantitative visual acuity testing were excluded from the intervisit cohort as there would be no way to detect clinical decline. Acceptable scans were defined as no obvious motion artifacts and even illumination (no vignetting or shadowing) on OCT fundus images.
Image Acquisition With Handheld Optical Coherence Tomography
Handheld OCT acquisition was identical to the previously published protocol. Mydriatic eye drops were instilled approximately 1 hour before the subjects’ MRI. Once the child was adequately sedated using a standardized anesthesia protocol, handheld OCT imaging was performed using a high-resolution handheld device acquiring 36 000 A-scans per second (Bioptigen, Durham, North Carolina, USA). A 6 × 6 × 2 mm volume scan centered on the optic nerve head using 1000 A-scans across 100 B-scans or 300 A-scans across 300 B-scans was acquired. The total number of handheld OCT volumes acquired during an imaging session was dependent on the time available to the examiner, as the current protocol does not prolong the child’s exposure to anesthesia.
Handheld Optical Coherence Tomography Image Analysis
Automated custom-made software segmented the volume and determined the circumpapillary RNFL thickness. The optic nerve head margin was drawn manually by the same investigator (C.-L.C.). Circumpapillary RNFL measures were derived from 256 A-scans around a 3.4 mm circle that was centered at the geographic center of the optic nerve head, and were equally divided into 4 quadrants (ie, 64 samples per quadrant). One investigator (C.-L.C.) processed all of the raw handheld OCT data, which were de-identified and did not include clinical information.
As the device did not provide signal quality information for each volume, image and signal quality was quantified by calculating the quality index based on a previously described method. Briefly, quality index (QI) was the product of 2 parameters, intensity ratio and tissue signal ratio, acquired from a signal intensity histogram of an OCT volume. The histogram represents the pixel intensity/reflectivity distribution of the entire volume. Intensity ratio is analogous to signal-to-noise ratio for the entire volume, whereas tissue signal ratio is the ratio of the number of pixels of retinal signal vs background noise. Handheld OCT scans with a QI value less than 22 were considered to be of poor image quality and were eliminated from all analysis.
Statistics
Demographic and clinical characteristics were summarized by standard descriptive statistics (eg, means and standard deviations for continuous variables). The Shapiro-Wilk test for normality was used to determine the need for parametric ( t test) vs nonparametric (Wilcoxon rank-sum test) comparisons when analyzing the differences between volume type and vision groups. A paired t test was performed for subjects contributing eyes to both volumes. The coefficient of variation (CV) and ICC (2-way mixed-effects model) was calculated for the global average and anatomic quadrant circumpapillary RNFL thickness of the intra- and intervisit cohorts. Subjects could contribute 2 or more scans of the same volume, eye, and visit to the CV analysis in the intra- and intervisit cohorts. For subjects with more than 1 eligible imaging session, their earliest study visit was selected for the intravisit cohort. The 2 scans with the highest QI were selected for the intravisit ICC calculation. The average of all available quality scans from visit 1 and visit 2 were used to calculate the intervisit ICC. In cases of a unilateral isolated optic nerve glioma without vision loss, the non–optic pathway glioma eyes were eliminated from the analysis. When normal vision subjects had eligible scans contributed from both eyes, a random-number generator determined which eye would be included in the analysis. Children could contribute both eyes to an analysis if one was classified as having abnormal vision while the other eye was classified as having normal vision. Children with vision loss in both eyes were permitted to contribute both eyes to the analysis, as the magnitude and location of their deficit was never identical between eyes. Data were analyzed using commercially available software (STATA, version 13; StataCorp, College Station, Texas, USA).
Results
Intravisit Cohort
Fifty-nine subjects contributed to the intravisit analysis. The median age was 4.6 years (range 0.79–13.0, N = 36), with 61% of subjects being female. A majority of subjects were white (68%, n = 40), followed by multiracial (15%, n = 9), black non-Hispanic (14%, n = 8), and Asian (3%, n = 2). Fifty-nine percent of subjects (n = 35) had an optic pathway glioma secondary to NF1, 24% (n = 14) had sporadic optic pathway gliomas, and 17% (n = 10) had NF1 without an optic pathway glioma. Twenty-seven optic pathway glioma subject eyes experienced vision loss; 12 were imaged with nonisotropic volumes (NF1–optic pathway glioma = 4, sporadic optic pathway glioma = 8) and 15 were imaged with isotropic volumes (NF1–optic pathway glioma = 7, sporadic optic pathway glioma = 8). Thirty-six subjects contributed 1 eye to each volume. Table 1 lists the RNFL thickness, CV, ICC and ICC 95 th percentile confidence interval for the non-isotropic and isotropic volumes of the intravisit cohort.
Average | Region | ||||
---|---|---|---|---|---|
Superior | Nasal | Inferior | Temporal | ||
1000 × 100 a | |||||
Normal vision | |||||
N = 32 b | |||||
Thickness (mean ± SD) | 126.4 ± 13.6 | 155.7 ± 20.2 | 102.3 ± 15.8 | 150.6 ± 18.3 | 97.1 ± 19.3 |
CV (%) | 1.5 | 3.0 | 4.6 | 3.4 | 4.0 |
ICC | 0.987 | 0.953 | 0.934 | 0.931 | 0.963 |
ICC 95% CI | 0.97, 0.99 | 0.90, 0.97 | 0.86, 0.96 | 0.86, 0.96 | 0.92, 0.98 |
Abnormal vision | |||||
N = 12 b | |||||
Thickness (mean ± SD) | 81.8 ± 22.9 | 102.0 ± 27.2 | 72.4 ± 25.3 | 96.9 ± 33.7 | 56.1 ± 22.7 |
CV (%) | 3.3 | 4.2 | 11.5 | 3.7 | 8.3 |
ICC | 0.992 | 0.987 | 0.956 | 0.992 | 0.962 |
ICC 95% CI | 0.97, 0.99 | 0.95, 0.99 | 0.85, 0.98 | 0.97, 0.99 | 0.86, 0.98 |
300 × 300 c | |||||
Normal vision | |||||
N = 46 b | |||||
Thickness (mean ± SD) | 125.7 ± 19.9 | 157.1 ± 25.3 | 100.5 ± 19.1 | 150.0 ± 25.0 | 95.4 ± 22.3 |
CV (%) | 2.5 | 3.5 | 6.4 | 4.3 | 5.1 |
ICC | 0.985 | 0.967 | 0.929 | 0.967 | 0.953 |
ICC 95% CI | 0.97, 0.99 | 0.94, 0.98 | 0.87, 0.96 | 0.94, 0.98 | 0.91, 0.97 |
Abnormal vision | |||||
N = 15 b | |||||
Thickness (mean ± SD) | 91.2 ± 16.2 | 113.6 ± 19.9 | 79.9 ± 14.9 | 111.4 ± 26.0 | 59.9 ± 19.5 |
CV (%) | 3.3 | 3.3 | 6.3 | 4.2 | 9.4 |
ICC | 0.990 | 0.982 | 0.958 | 0.987 | 0.954 |
ICC 95% CI | 0.96, 0.99 | 0.94, 0.99 | 0.87, 0.98 | 0.96, 0.99 | 0.85, 0.98 |
a A total of 104 scans contributed to the CV analysis.
Nonisotropic volume analysis: intravisit cohort
Thirty-eight subjects contributed 44 subject eyes (104 volumes acquired) to the nonisotropic analysis, 12 of which had abnormal vision. All circumpapillary RNFL quadrants and global average thicknesses were higher in the normal vision group compared to the abnormal vision group ( P < .001 for all comparisons). The average circumpapillary RNFL thickness demonstrated a lower CV and higher ICC compared to the quadrants in both the normal and abnormal vision groups. The nasal quadrant, regardless of vision status, most frequently demonstrated the highest CV and the lowest ICC, although on occasion other quadrants would demonstrate similar results.
All CV values were not normally distributed ( P < .001). The CV values of the average, superior, inferior, and temporal quadrants were not statistically different between those with and without vision loss ( P > .05, all comparisons). In the nasal quadrant, the CV of the normal vision group was significantly lower compared to the abnormal vision group (Z = −3.162, P < .01; 4.6% vs 11.5%).
Isotropic volume analysis: intravisit cohort
Fifty-three subjects contributed 61subject eyes (197 volumes), 12 with vision loss, to the isotropic volume analysis ( Table 1 ). All circumpapillary RNFL quadrants and global average thicknesses were higher in the normal vision group compared to the abnormal vision group ( P < .001 for all comparisons). The average circumpapillary RNFL thickness demonstrated a lower CV and higher ICC compared to the quadrants in both the normal and abnormal vision groups. The superior and inferior quadrants demonstrated similarly high ICC values, whereas the nasal and temporal quadrants showed the lowest ICC values, with the low end of the confidence interval reaching 0.87 and 0.85, respectively. The nasal quadrant, regardless of vision status, most frequently demonstrated the highest CV and the lowest ICC, although on occasion other quadrants would demonstrate similar results.
The CV values of the superior, nasal, and inferior quadrants were not statistically different between those with and without vision loss ( P = .98, P = .59, P = .78, respectively). The normal vision group’s CV was lower for the temporal quadrant ( P = .0071; 5.1% vs 9.4%) and neared significance ( P = .050) for the global average (2.5% vs 3.3%).
Intravisit cohort: volume comparison
The nonisotropic and isotropic volumes did not show statistically significantly different CV values in all quadrants and the global average ( P > .05, all comparisons), even when comparing based on vision loss/status. For subjects contributing eyes to both volume types, a paired t test of CV values failed to demonstrate a significant difference between volumes across all quadrants ( P > .05, all comparisons).
Intervisit Cohort
Twenty-nine unique subjects (median age 5.7 years, range 1.8–12.7 years) contributed in the intervisit analysis. This cohort included more female (17/29 = 58.6%) and white subjects (22/29, 76%), followed by multiracial (5/29, 17%) and black non-Hispanic (2/29, 7%). Twenty-one had NF1-related optic pathway gliomas, 7 had sporadic optic pathway gliomas, and 1 had NF1 without an optic pathway glioma. Nine subject eyes experienced vision loss (NF1–optic pathway glioma = 6, sporadic optic pathway glioma = 3). Table 2 lists the RNFL thickness, CV, ICC, and ICC 95th percentile confidence interval for the nonisotropic and isotropic volumes of the intervisit cohort. In general, the global average demonstrated the lowest CV and highest ICC measures. The superior, inferior, and temporal quadrants demonstrated similar, but slightly more variable, CV and ICC measures as compared to the global average. The nasal quadrant typically demonstrated the highest CV values.
Average | Region | ||||
---|---|---|---|---|---|
Superior | Nasal | Inferior | Temporal | ||
1000 × 100 | |||||
Normal vision | |||||
N = 17 a | |||||
Thickness (mean ± SD) | 126.8 ± 15.1 | 156.5 ± 20.6 | 99.2 ± 14.5 | 151.7 ± 21.4 | 99.8 ± 17.9 |
CV (%) | 1.9 | 3.3 | 6.2 | 2.9 | 3.3 |
ICC | 0.981 | 0.939 | 0.868 | 0.962 | 0.977 |
ICC 95% CI | 0.95, 0.99 | 0.83, 0.97 | 0.64, 0.95 | 0.89, 0.98 | 0.93, 0.99 |
Abnormal vision | |||||
N = 2 a | |||||
Thickness (mean ± SD) | 93.9 ± 14.8 | 117.7 ± 11.1 | 82.7 ± 11.5 | 113.2 ± 20.0 | 62.2 ± 21.0 |
CV (%) | 2.7 | 3.8 | 11.0 | 4.6 | 5.9 |
ICC | – | – | – | – | – |
ICC 95% CI | – | – | – | – | – |
300 × 300 | |||||
Normal vision | |||||
N = 24 a | |||||
Thickness (mean ± SD) | 127.5 ± 16.3 | 159.3 ± 22.3 | 100.1 ± 16.5 | 155.2 ± 23.2 | 95.3 ± 18.0 |
CV (%) | 1.9 | 3.7 | 5.8 | 2.8 | 2.9 |
ICC | 0.984 | 0.948 | 0.916 | 0.968 | .986 |
ICC 95% CI | 0.96, 0.99 | 0.88, 0.97 | 0.88, 0.96 | 0.92, 0.98 | 0.96, 0.99 |
Abnormal vision | |||||
N = 7 a | |||||
Thickness (mean ± SD) | 99.1 ± 16.8 | 120.8 ± 21.3 | 89.1 ± 15.6 | 121.6 ± 29.9 | 64.7 ± 17.6 |
CV (%) | 3.1 | 4.5 | 3.6 | 6.6 | 3.4 |
ICC | 0.978 | 0.962 | 0.965 | 0.956 | 0.99 |
ICC 95% CI | 0.77, 0.99 | 0.80, 0.99 | 0.75, 0.99 | 0.59, 0.99 | 0.96, 0.99 |
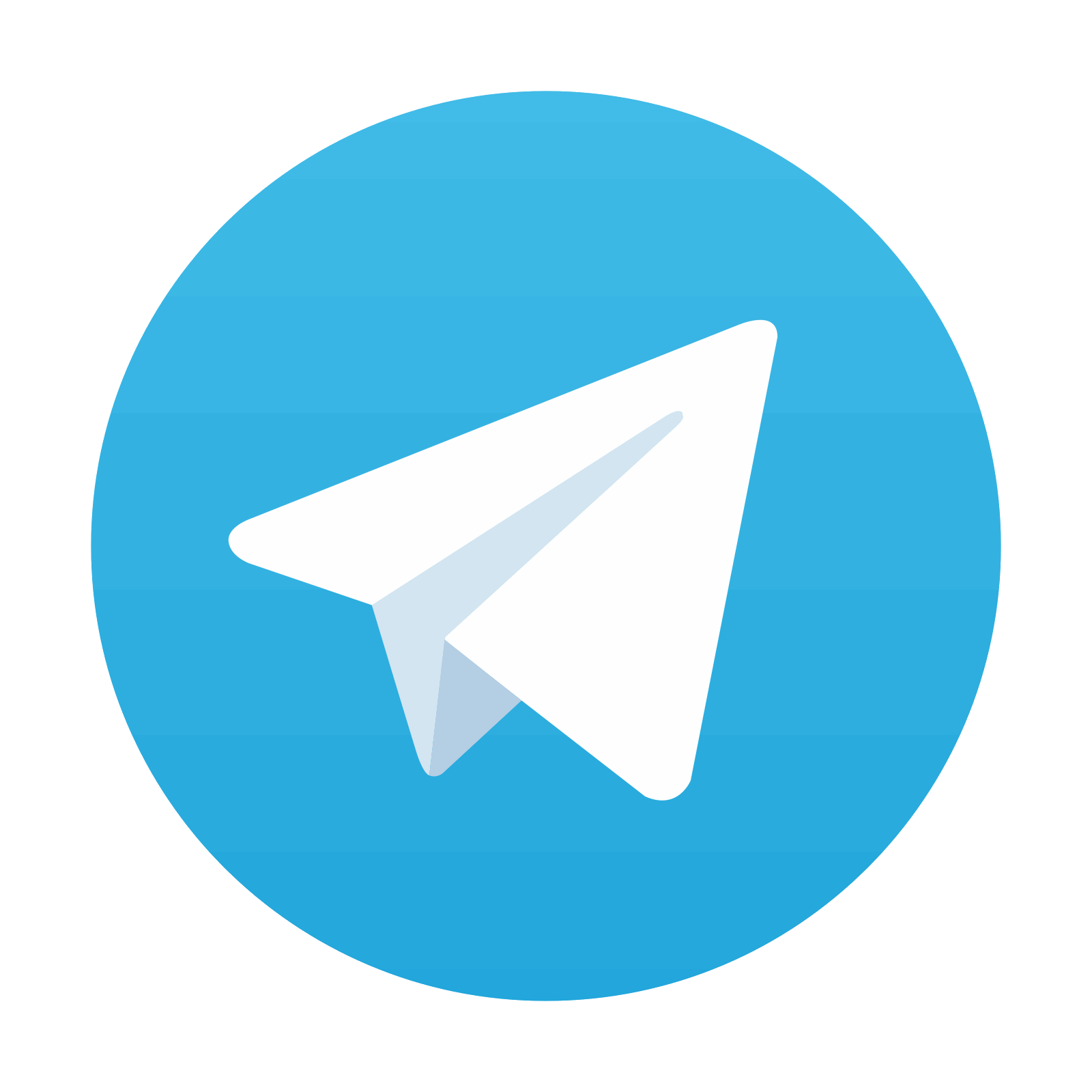
Stay updated, free articles. Join our Telegram channel

Full access? Get Clinical Tree
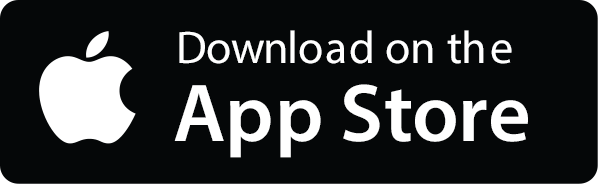
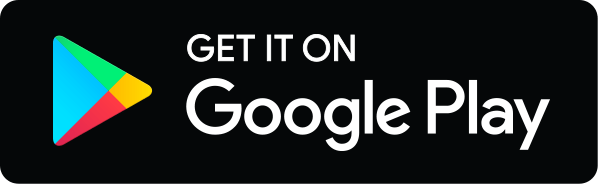
