33
Rehabilitation and Reanimation of the Paralyzed Face
Kevin A. Shumrick
The human face is unique across the animal kingdom in its ability to express a wide range of emotions and intent. Facial expressions in lower animals are used primarily to express anger or aggression, whereas the human face is capable of clearly signaling to other humans the four major emotions (fear, anger, happiness, and sadness) as well as more subtle nuances such as sympathy, disgust, amusement, disbelief, and surprise. These facial expressions have clearly evolved to aid in the social interaction of humans, and paralysis of the face significantly compromises an individual’s ability to function effectively in society. Patients with facial paralysis present an alien countenance that others find disquieting. There is the unspoken fear that whatever is affecting the face is contagious, and many of the basics of social interaction (handshaking, cheek kissing) are approached with trepidation by both the patient and acquaintances. Patients with facial paralysis avoid having photos taken and thus drop out of the photographic record of a family. Similarly, patients begin to avoid social functions and family gatherings because they feel embarrassed and worry that they will make others uncomfortable. All these factors combine to isolate patients socially and are compounded when they try to reenter the work force. At the workplace patients with facial paralysis may face not only social isolation but also subtle discrimination that may prevent them from obtaining jobs or advancing in jobs.
In addition to their socializing functions, the muscles of facial expression are important for proper functioning of the face. The orbicularis oris and oculi are critical for maintaining the sphincteric effect of the lips and eyelids, and without them the affected side loses competency. With loss of the ipsilateral orbicularis oris the affected side of the mouth droops, and drooling of liquids and food results. It is difficult to use a straw and puff the cheek. Without the buccal muscle, food accumulates in the buccal space, contributing to halitosis and dental caries. The orbicularis oculi is responsible for the ability to actively blink the eye and squint. Loss of the ability to effectively blink predisposes the eye to desiccation and corneal ulcers as well as injury by foreign bodies that would normally be blocked by blinking or squinting.
Even though this chapter is devoted to rehabilitation of the paralyzed face, an understanding of the basics of facial nerve anatomy, physiology, and function is essential to plan the timing and method of repair. Additionally, a basic understanding of the fundamentals of nerve degeneration and regeneration helps the surgeon recognize when success of a facial nerve repair is not likely and plan for an alternative method of reanimation.
Facial Nerve Anatomy
The facial nerve is composed of both sensory and motor nerve fibers. Distal to the geniculate ganglion the facial nerve consists of 10,000 fibers of which 7000 are myelinated and innervate the muscles of facial expression.1 The remaining 3000 fibers supply secretomotor fibers to the salivary and lacrimal glands, and sensory fibers to the lingual taste buds. By the time the facial nerve has exited the stylomastoid foramen, most of the sensory nerve fibers have left the main trunk, and the remaining portion of the nerve is composed almost entirely of myelinated fibers to the facial muscles.
Each motor neuron consists of a cell body located at the brainstem with a myelinated axon that travels to a motor end-plate located on a facial muscle. The axons have Schwann cells on their surface, which produce myelin. The myelin is present throughout the length of the axon except at the nodes of Ranvier, which are approximately 2 mm apart (Fig. 33–1). Outside the Schwann cell of each axon is the endoneurium, which is a loose connective tissue layer. The facial nerve axons, with their enclosing endoneurium, are grouped into fascicles of varying numbers of axons. These fascicles are distinguished by being surrounded by a layer of connective tissue referred to as perineurium. Finally, the fascicles are bundled together, along with venules and arterioles, by the epineurium into what is formally called a nerve. The epineurium is also referred to as the nerve sheath (Fig. 33–1). The facial nerve does not begin as a multifascicular nerve; when it exits the brainstem and enters the internal auditory canal, it consists of a single fascicle. This monofascicular arrangement continues through the labyrinthine and tympanic segments of the nerve. Distally, in the mastoid portion, the nerve begins to divide into several fascicles, with each fascicle surrounded by perineurium (Fig. 33–1). At the level of the stylomastoid foramen the nerve has divided into six to ten fascicles.2 There has been a lively debate in the literature as to whether the main trunk of the facial nerve has a topographic distribution, which could be useful when performing nerve repairs or nerve grafting.3–5 This is discussed further in the section on nerve repair, but at present the topographic distribution of the facial nerve has not proven to be clinically useful.
Figure 33–1 Cross-sectional anatomy of the facial nerve as it travels from the temporal bone to the facial muscles. Note that in the internal auditory canal it is monofascicular and in the mastoid portion of the facial canal it is divided into multiple fascicles by perineurium.
Because several methods of facial reanimation require grafting or nerve transfer to the intraparotid facial nerve, a brief discussion of the extratemporal bone facial nerve anatomy is in order. The facial nerve exits the temporal bone at the stylomastoid foramen. It then travels anteriorly through the substance of the parotid as a single nerve for approximately 2 cm, at which point it consistently bifurcates into an upper and lower division (Fig. 33–2). The facial nerve divisions continue anteriorly for several millimeters and then divide into a total of five branches, giving rise to the pes anserinus. The typical branches of the facial nerve are the temporal, zygomatic, buccal, mandibular, and cervical, and these branches can have significant variation in their subsequent course and subdivisions.6–8 Davis et al6 identified six different patterns of facial nerve branching with no one type occurring more than 28% of the time.
Even with this variability there are several anatomic land-marks that can facilitate locating various key portions of the extratemporal facial nerve, as noted by Crumley and Scott9 (Fig. 33–3):
1. The pes anserinus is consistently located at a point 1 cm anterior and 2 cm inferior to the tragal cartilage.
2. The superior division of the facial nerve travels on a line from the pes anserinus to approximately the lateral corner of the eyebrow.
3. The buccal branch leaves the pes anserinus and travels anteriorly and slightly superiorly passing 1 cm inferior to the bottom of the zygomatic arch.
4. The marginal mandibular branch leaves the pes and travels inferiorly and anteriorly, passing down over the angle of the mandible and then proceeding inferior to the rim of the mandible for 3 cm before ascending back over the rim of the mandible at its junction with the facial vessels.
Despite the variability of these facial nerve branching patterns, there is consistently found to be cross-innervation between the various branches and even between the upper and lower divisions. This cross-innervation between divisions and branches of the facial nerve is most pronounced between the zygomatic and buccal branches and least for the marginal mandibular branch. This explains why significant deficits of the midfacial muscles are rarely seen from a peripheral facial nerve injury and relatively common from a marginal mandibular nerve injury. This interconnection of branches and divisions also helps explain the phenomenon of synkinesis following injury of the main trunk of the facial nerve because with regeneration the neuronal sprouts not only have free access to reinnervate their original muscle fibers, but also take aberrant routes to facial muscles of different branches and even different divisions. It is not surprising, therefore, that the midface (which has the most interconnections) is also the site of most prominent synkinesis, with the eye and upper lip moving in synchrony.
Figure 33–2 Branching of intraparotid facial nerve into upper and lower divisions with five main branches. There is considerable cross-innervation between the zygomatic and buccal branches, which makes these branches most resistant to peripheral injuries, but also most susceptible to synkinesis.
Figure 33–3 Anatomic landmarks for portions of the extratemporal facial nerve. The pes anserinus is located approximately 1 cm anterior and 2 cm inferior to the tragus. The marginal branch dips inferior to the margin of the mandible at the angle and then reemerges above it approximately 3 cm anterior to the angle. The buccal branch runs approximately 1 cm inferior to the zygomatic arch.
Physiology of Nerve Repair
The facial nerve, like other motor nerves, is composed of central nervous system (CNS) axons, which have synaptic connections with several adjacent muscle fibers (referred to as a motor unit). There are estimates that each of the 7000 neuron cell bodies supplies innervation for approximately 25 muscle fibers. It is recognized in muscle physiology that the smaller the ratio of muscle fibers to neuron cell bodies, the more specialized the movement possible. As an example, the gastrocnemius muscle has a ratio of 2000 muscle cells for each neuron cell body, whereas in the larynx the ratio is one to one.8 The nerve cell body, located in the pons, manufactures functionally important substances, such as neurotransmitters and enzymes, which are transported via the axoplasmic transport system to the motor end-plate.10,11 Additionally, trophic substances produced at the distal end of the nerve are transmitted back to the cell body and allow central monitoring of the distal nerve via these molecules. Thus, we see that the individual nerve fibers are in a dynamic state with regard to monitoring the health status of the entire nerve and adjusting to changing conditions. When a nerve is injured, distinct histologic and biochemical changes take place in the proximal cell body, distal injured nerve, motor end-plate, and motor unit (muscle fibers). The degree and severity of these changes are due to a variety of factors, which include type of injury (i.e., crush versus transection), age of patient, nutritional status, and distance from injury to cell body.10 Sunderland12,13 has developed a classification of facial nerve injury, based on the degree of disruption, which accounts for the observed physiology of nerve repair and predicts the degree of functional recovery. With the fourth- and fifth-degree nerve injuries, commonly seen with facial paralysis patients, all components of the nerve fiber (endoneurium, perineurium, and epineurium) are disrupted.
The neuron cell body undergoes marked metabolic changes following transection of an axon. Protein synthesis and RNA increase steadily for approximately 3 weeks, at which time their levels reach a steady state that persists until the nerve has reestablished connections with a motor end plate. The observation that it takes approximately 3 weeks for a nerve fiber to fully convert to a regenerative state has led some authors to advocate waiting 3 weeks before performing a nerve repair or nerve grafting; however, it has now been well established that the optimum time for repair is as soon after the injury as possible. In the proximal stump of the injured nerve, axonal sprouts begin to form within 3 days of transection. These axonal sprouts grow peripherally at a rate of approximately 1 mm per day. Thus, for a facial nerve injury in the temporal bone to regenerate 14 cm to the facial muscles would take approximately 140 days.8
In the nerve segment distal to the site of injury, Wallerian degeneration begins rapidly. The Schwann cells proliferate and convert into macrophages, which phagocytose the myelin, axoplasm, and other products of degeneration. The motor end-plates retract, and if they are not reinnervated within a reasonable amount of time (the exact time is not known but appears to be several years), the synaptic clefts will eventually be obliterated by collagen deposition.
It should be noted that with a facial nerve injury in which the nerve has been transected, the distal nerve end will still conduct impulses via a nerve stimulator and cause facial motion for up to 72 hours after the injury. This is a crucial time period for nerve repair because it allows the surgeon to employ the nerve stimulator to accurately and definitively identify the distal end of the nerve and perform a primary repair or nerve graft. Delay of repair beyond the 72-hour time period means that the nerve must be dissected much more extensively to ensure that the distal end has been accurately identified. Additionally, scarring and fibrosis will begin making the dissection even more difficult. All these factors argue for early exploration of an injury when transection is suspected, and performing a repair in as timely a fashion as possible.
Facial Muscle Degeneration
Although specific data on the degeneration of human facial muscles is lacking, it appears that facial muscles follow a comparable course to other muscles that are denervated. Namely, following denervation there is a progressive diminution of muscle fiber diameter to approximately one-half the original diameter within 1 month of denervation. Following this acute phase of degeneration the muscle fiber appears to stabilize and remains unchanged for a variable period of time. The eventual degree of muscle degeneration is related to the completeness of the original neuronal lesion and whether or not the muscle obtains some degree of reinnervation (from the facial nerve, adjacent facial muscles, or aberrant reinnervation from an alternate nerve such as the trigeminal). During this time it appears that the muscle fiber is susceptible to reinnervation, although with reinnervation it does not regain its original bulk.
A crucial issue in performing a nerve graft or nerve transfer is whether or not there are receptive, viable muscle fibers available for reinnervation; if not, then an alternative method of reanimation should be chosen. This concept is critical to avoid the tragic scenario of performing a hypoglossal-facial anastomosis in a patient with irreversibly atrophied facial muscles who will not regain facial movement and will have the additional disability of a hemitongue deficit. The only way to accurately and expeditiously determine the status of the facial muscles is through electromyography (EMG). EMG can provide four types of information with regard to the status of the facial muscles9: (1) EMG can show normal voluntary action potentials, which indicate that the muscles have connections with nerves and are being stimulated to voluntarily contract (indicating that the facial muscles are innervated and not receptive to reinnervation). (2) Polyphasic potentials may be detected, which indicate that reinnervation is proceeding even if there has not been return of voluntary function. The implication is that with simple observation there should be return of innervation and surgery should be delayed and the patient observed for an additional period of time. (3) Denervation or fibrillation potentials indicate the presence of viable but denervated muscle fibers that should be receptive to an attempt at reinnervation. (4) A final possible EMG finding is electrical silence, which indicates the absence of viable, functioning muscle fibers. This could be due to atrophy or congenital absence; in either event a reinnervation attempt would be unsuccessful due to a lack of viable, receptive muscle fibers for the nerve to reinnervate.
Facial Nerve and Muscle Regeneration
When the Schwann cells begin to phagocytose the myelin surrounding the axons, they form tubes referred to as Büngner bands. With regeneration the sprouting axons find their way (probably via chemotaxis factors) into these Büngner tubes and thereby to the denervated muscle fibers. As axonal sprouts form at the proximal end of the neuron cell body, there may be multiple individual sprouts. However, only one sprout is retained once one reaches a denervated muscle; the rest of the sprouts are then resorbed. There appears to be a time limit within which an axon needs to reestablish a connection with a muscle cell via the Büngner bands or it will undergo degeneration. The exact time course for this degeneration is variable but has been noted to occur within as little as 4 months. Thus, if a nerve is injured and requires a primary repair or nerve graft, the sooner the reparative surgery is performed the better the chances of eventual recovery.
Anatomy and Function of the Facial Muscles
The major disability of facial nerve paralysis is loss of motion of the facial muscles. Therefore, to restore some semblance of normal facial function, it is important to understand the arrangement and function of the facial musculature. The major physiologic function of the facial musculature is to provide competent sphincters of the mouth and eye. Ancillary functions would include aiding mastication and expressing emotions. The ability to express facial emotions through the manipulation of soft tissue by the facial muscles arises from the fact that facial muscles have extensive connections with the overlying facial skin. These attachments of muscle to skin are somewhat unique in the human body; the usual situation for voluntary, skeletal muscle is for it to connect bone to bone or bone to tendon.
Muscles of the Periorbital Region
The major functional muscle of the periorbital region is the orbicularis oculi, which constitutes the majority of the soft tissue of the eyelids (Fig. 33–4). The orbicularis oculi run longitudinally along the eyelids with connections to the medial and lateral canthal ligaments, frontal bone, maxilla, zygoma, and skin. When the orbicularis oculi contracts, the eyelids are foreshortened and closed. It is important to note that, as will be seen in the section on eyelid reanimation, the major movement during eyelid closure comes from the upper eyelid with the lower eyelid moving only slightly.
Figure 33–4 The major muscles of facial expression.
The importance of the ability to close the eye rapidly and forcefully cannot be overemphasized. The blink is a major protective mechanism from foreign bodies as well as for lubricating the eye and removing any debris that may have accumulated on the surface of the cornea. Additionally, the ability to squint or narrow the eye aperture is important to protect the eye from too much light or blowing objects (e.g., sand, rain, etc.).
Other muscles of the forehead region function primarily to move the eyebrows and these are the frontalis, corrugator supercilii, and procerus (Fig. 33–4). Although these muscles are much less important for protecting the eye than the orbicularis oculi, they are quite significant for maintenance of normal facial expression. A major consequence of forehead paralysis is pronounced drooping of the eyebrow, which is frequently so severe that, in conjunction with a hypotonic orbicularis oculi, it will droop over the eye and cause visual field obstruction.
Perioral Muscles
The perioral muscles function primarily to control the lips and provide the sphincteric action necessary for mastication and speech. They also provide the motion to bring about the smile and its variations, which are, in all likelihood, the most important of facial expressions. The major perioral muscle is the orbicularis oris, which encircles the mouth and provides the bulk of soft tissue comprising the lips (Fig. 33–4). With contraction of the orbicularis oris the lips are compressed together, the deep fibers press the lips against the teeth, and the superficial fibers pull the lip into a pout due to the purse-string effect.14
Although the orbicularis oris is the most important muscle for controlling the oral aperture, several other muscles affect the configuration and positioning of the lips. These muscles may be grouped into elevators of the upper lip, elevators of the corner of the mouth, depressors of the lower lip, and depressors of the corner of the mouth (Fig. 33–4). However, the major muscles affecting the positioning of the corner of the mouth, for practical purposes, are the zygomaticus major and, to a lesser degree, the zygomaticus minor (Fig. 33–4). The zygomaticus muscles arise from the inferior body of the zygoma and insert at the corner of the mouth in the region of the oral commissure by insinuating themselves with the orbicularis oris and depressors of the commissure. Additionally, the zygomaticus muscles send fibers to the overlying skin, which contribute to the formation of the nasolabial fold. The primary function of the zygomaticus muscles is to provide tone and support to the corner of the mouth (with maintenance of the nasolabial fold) and with active contraction elevation of the corner of the mouth into the primary position of the smile. The smile can be modified by the ancillary perioral muscles to add the subtleties of facial expression such as a sneer, grimace, etc.
Methods of Facial Reanimation
Reinnervation Techniques
Primary Anastomosis
If a facial paralysis results from trauma to the facial nerve, the most successful outcome will occur if the nerve is able to regenerate back through its original fascicles. This may be possible when there has been a crush-type injury to the nerve without actual transection. Once the integrity of the nerve has been disrupted, the best result will be obtained if the two ends of the nerve can be accurately reapproximated. It has been advocated that intrafascicular (using the perineurium) repair of neuron fascicles gives the best return of nerve function, and this method of repair has been proposed for repair of facial nerve injuries, to lessen the synkinesis that can significantly mar an otherwise successful neural repair. However, for intrafascicular repair to decrease synkinesis requires that the nerve has a distinct topographic distribution with nerves in each fascicle going primarily to specific muscles or muscle groups. There is some evidence that there may, in fact, be a distinct topographic orientation of the facial nerve. May4 had noted clinically that certain types of facial nerve injuries seemed to be reflected in specific deficits of facial movement. Kempe3 performed topographic stimulation of the facial nerve in patients undergoing resection of glomus jugulare tumors and noted a topographic representation of fibers within the main nerve trunk. However, a very detailed anatomic study by Gacek and Radpour2 showed that small fascicles of the facial nerve at the level of the internal auditory meatus carry motor fibers to all the distal branches of the facial nerve and that the facial nerve is, at best, only loosely topographically organized. Thus, although an intrafascicular repair does indeed ensure a more accurate reapproximation of individual fascicles, within each fascicle there are nerve fibers going to multiple, different muscles and synkinesis may still result.8,15 A further concern with intrafascicular repair is that several authors have raised the possibility that the additional dissection required for an intrafascicular repair could enhance scarring and injure the blood supply with significant diminution of functional results.16,17 If there is a clean laceration of the trunk with the two ends easily available and it is possible to clearly identify corresponding fascicles in each end, then it would be reasonable to attempt an intrafascicular repair of comparable portions of the nerve. Otherwise, most authors now advocate a meticulous epineurial repair with no attempt to reestablish a topographic intrafascicular repair.8,9,15 The suture method of choice is five to seven sutures of 9-0 monofilament used in a epineurial repair.18,19
The location of the transection of the nerve dictates, to some degree, the method of repair. Lesions of the facial nerve at the brainstem or in its temporal portion have been notoriously difficult to suture-repair a primary end-to-end anastomosis. At the brainstem the nerve lacks mobility and it is very difficult to suture deep in the wound. Kanzaki et al20
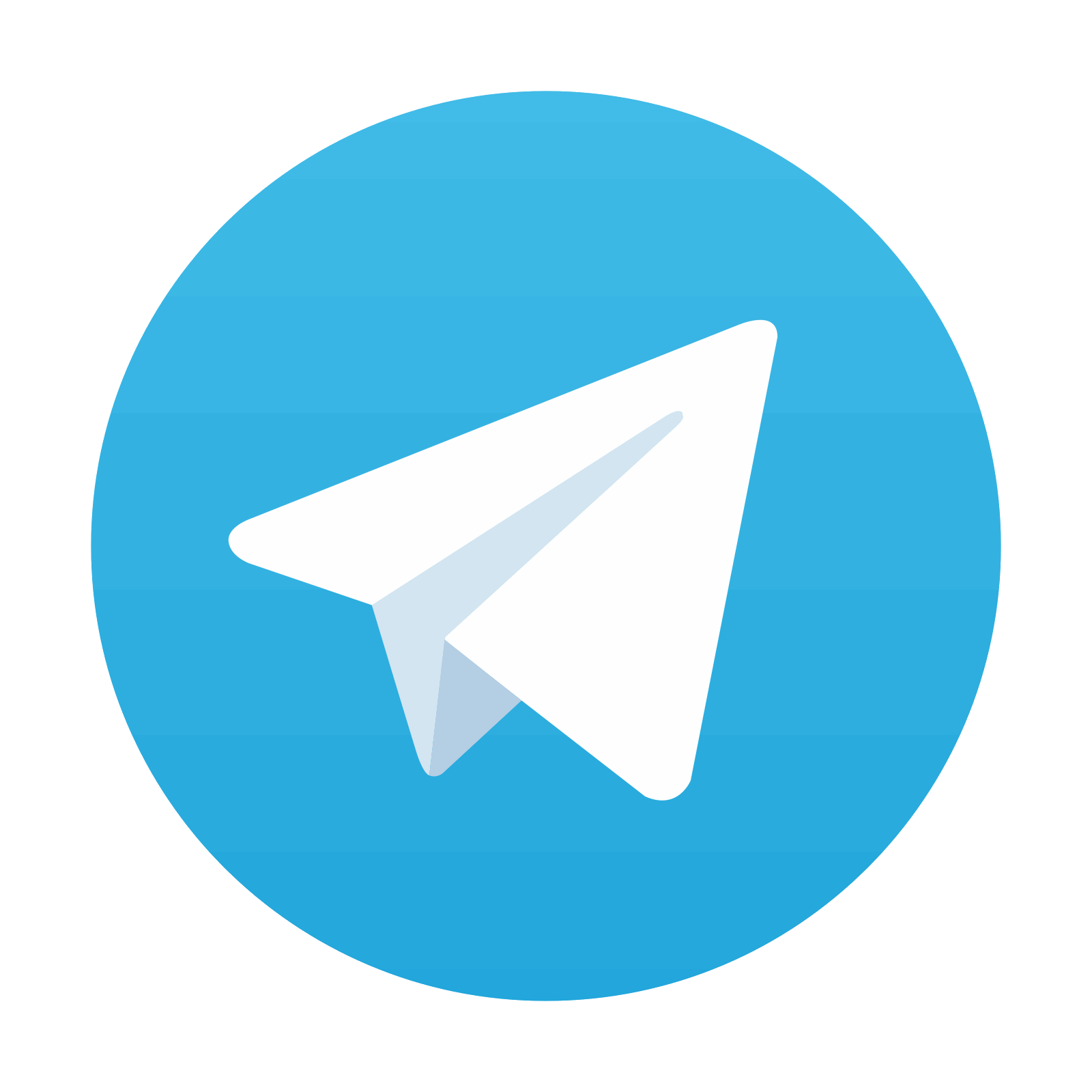
Stay updated, free articles. Join our Telegram channel

Full access? Get Clinical Tree
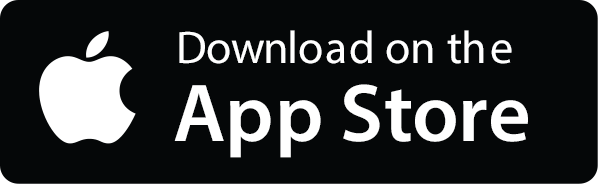
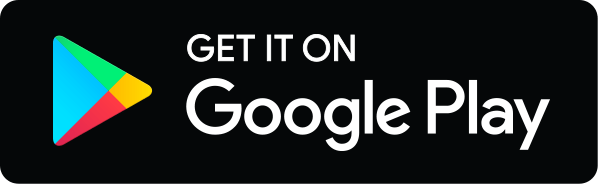