Regenerative Strategies for Overcoming Deafness
Alan G. Cheng
Stefan Heller
The mammalian organ of Corti comprises a complex but organized array of mechanosensitive hair cells separated by supporting cells (see Fig. 169.1). Upon mechanical stimulation, inner hair cells transmit neural signals to innervating spiral ganglia neurons, which in turn relay neural impulses to the central nervous system in a tonopic manner. Simultaneously, outer hair cells feed back mechanical forces to augment the sensitivity and frequency selectivity of the cochlea. The integrity of all of these cell types is critical for auditory function.
Etiologies of hearing loss include genetic disorders, acoustic trauma, aging, infection, and toxins such as aminoglycoside antibiotics and the antineoplastic agent cisplatin. One common underlying pathology of hearing loss is degeneration of outer and/or inner hair cells. While hair cell-specific mutations, certain drugs (e.g., aminoglycosides and cisplatin) and noise are likely the primary cause for sensory hair cell loss, there are also scenarios in which hair cells degenerate in non-cell autonomous fashion. Such a pattern of cell loss has also been observed in lesions primarily involving the supporting cells such as gap junction gene mutations (e.g., GJB2 encoding connexin 26) (1) or mutations that affect ion homeostasis in the stria vascularis (2). Because of the established causal relationship between hair cell loss and hearing loss, much effort has been devoted to investigate hair cell regeneration as a possible therapeutic intervention for hearing loss.
HAIR CELL REGENERATION, NONMAMMALIAN VERTEBRATES VERSUS MAMMALS
In non-mammalian vertebrates such as fish, amphibians, and birds, sensory organs have the inherent capacity to replace lost hair cells. In fish, the lateral line organs are used to detect water movement and contain hair cells that are continuously turned over and replaced (3). Likewise, in the vestibular organs in birds, sensory hair cells degenerate and regenerate at a steady state, and the degree of regeneration increases upon damage by aminoglycoside treatment, which has been investigated in the macula of utricle (4, 5), suggesting that loss of hair cells induces a repair response. A similar regenerative response exists in the avian basilar papilla (hearing organ in birds), where researchers discovered recurrence of sensory hair cells initially lost from damage caused by aminoglycoside or noise (6, 7, 8). Indeed, these newly regenerated hair cells were innervated and hearing function was restored (9). Complex cortical functions including language acquisition requires intact neural connections between the cochlear end organ to higher cortical centers. When correlates of such learning ability were examined, song birds with regenerated cochleae that had initially been lesioned with aminoglycosides learnt and sang new songs (10). These lines of evidence indicate that in non-mammalian vertebrates, hair cell regeneration is part of the inner ear’s natural self-repair process.
In recent years, researchers have built on the above initial findings to delineate the mechanisms underlying hair cell regeneration. Using thymidine analogs to mark proliferating cells, the supporting cells in both the avian basilar papilla and utricles were found to generate new hair cells in two manners: via mitotic division or transdifferentiation (11). Transdifferentiation occurs when supporting cells directly acquire a hair cell phenotype without a preceding mitotic division. When the process of hair cell regeneration was examined in the avian cochlea after lesioning by aminoglycosides, both processes significantly contribute to the repopulation of lost hair cells (11, 12). Inhibitors of proliferation do not affect the degree of supporting cell transdifferentiation, suggesting that the two mechanisms may operate independently of each other (12).
In the mammalian cochlea, loss of hair cells is permanent and no spontaneous regeneration of hair cells has been observed. Unlike the cochlea, limited hair cell
regeneration has been observed in mammalian utricles. Morphologic evidence suggests that new, immature hair cells appear after aminoglycoside damage in vitro (13, 14), yet the lack of proliferation marker among these hair cells implies that they emerge via transdifferentiation, most likely from supporting cells. Recently, cells isolated from the mammalian utricular sensory epithelium were found to have stem cell properties, where they self-renew and were pluripotent (15), and may contribute to the regenerative capacity of utricles. Cells with stem cell properties were also found in the neonatal (see section below), but not adult cochlea (16); mechanisms underlying this fundamental difference between the mammalian auditory and balance organs are not completely understood.
regeneration has been observed in mammalian utricles. Morphologic evidence suggests that new, immature hair cells appear after aminoglycoside damage in vitro (13, 14), yet the lack of proliferation marker among these hair cells implies that they emerge via transdifferentiation, most likely from supporting cells. Recently, cells isolated from the mammalian utricular sensory epithelium were found to have stem cell properties, where they self-renew and were pluripotent (15), and may contribute to the regenerative capacity of utricles. Cells with stem cell properties were also found in the neonatal (see section below), but not adult cochlea (16); mechanisms underlying this fundamental difference between the mammalian auditory and balance organs are not completely understood.
In cochlea damaged as a result of aminoglycoside administration, hair cell loss is accompanied by an initial preservation of adjacent supporting cells (17). Although expression of specific markers in surviving supporting cells are mostly unaltered immediately after damage, the architecture of the sensory epithelium successively transforms from pseudostratified and complex, via an intermediate cell mound with collapsed tunnel of Corti, into to a flat, monolayered epithelium (see Fig. 169.2). A modest loss of supporting cells was noted in long deafened cochleae (17, 18, 19).
Aminoglycoside-induced hair cell loss is one of the best understood model systems for hearing loss/deafness. Studies on this model of cochlear degeneration illustrate the dynamic cellular and architectural changes following hair cell degeneration, and allow the investigation of various approaches aiming to regenerate hair cells. Yet it is important to note that this model of cochlear degeneration may differ, at least in part, from other causes of hearing loss such as those involving other cell types including mutations of the connexin or pendrin genes (1, 20).
NOTCH PATHWAY
One key molecular pathway regulating hair cell differentiation is the Notch signaling pathway, which plays several roles during the development of many organ systems. In regenerating chicken basilar papilla, Notch ligand expression is upregulated and inhibition of Notch signaling causes excessive regeneration of hair cells (21). This finding suggests that active Notch signaling functions to suppress supporting cells from acquiring a hair cell fate. However, Notch inhibition fails to induce extra hair cell formation from quiescent supporting cells in the undamaged avian cochlea, suggesting that a separate damage-induced signal is necessary.
In the developing cochlear duct, hair cells and supporting cells share a common progenitor (22). During late embryogenesis, Notch signaling pathway regulates the mammalian cochlea through a process termed lateral inhibition (reviewed in [23]). Developing hair cells express Notch ligands (24, 25), which in turn activate Notch signaling in adjacent supporting cells. Active Notch signaling in supporting cells turns on the Hes and Hey genes and represses the expression of Atoh1, a gene critical for hair cell differentiation (see section below). Such an interaction is important in the formation of rows of hair cells intercalated by supporting cells (26). Similar to the avian cochlea, inhibition of the Notch pathway leads to ectopic hair cell formation in the embryonic and early postnatal mouse cochlea, indicating that mammalian supporting cells have the potential to acquire a hair cell phenotype (27, 28, 29, 30). Stringent analyses identified a subset of organ of Corti supporting cells capable of directly differentiating into hair cells in the neonatal mouse cochlea: (a) inhibition of Notch signaling leads to excessive outer hair cells at the expense of Deiters’ cells, and (b) inhibition of both Notch and fibroblast growth factor (FGF) signaling causes formation of excessive inner hair cells from pillar cells (29, 31). Together these experiments suggest that supporting cells can be coerced to become new hair cells, and raise the question whether they can also function to replace lost hair cells under pathologic conditions.
To gain insights into the role of Notch signaling in hair cell regeneration, researchers have studied the use of gamma secretase inhibitors, which are potent antagonists of Notch signaling by preventing cleavage and release of the intracellular domain on Notch receptors that is responsible for downstream gene regulation. In damaged chicken basilar papilla and the zebrafish lateral line system, both organs that spontaneously regenerate lost hair cells, Notch inhibition led to an increase in hair cell production (21, 32). These observations suggest that events occurring during hair cell regeneration recapitulate those in development.
Whether inhibition of Notch signaling can stimulate hair cell regeneration in the mature mammalian cochlea has not been extensively examined. In one study where guinea pigs were deafened by aminoglycoside, pharmacologic inhibition of Notch signaling has yielded limited ectopic hair cells without regeneration of outer or inner hair cells (33
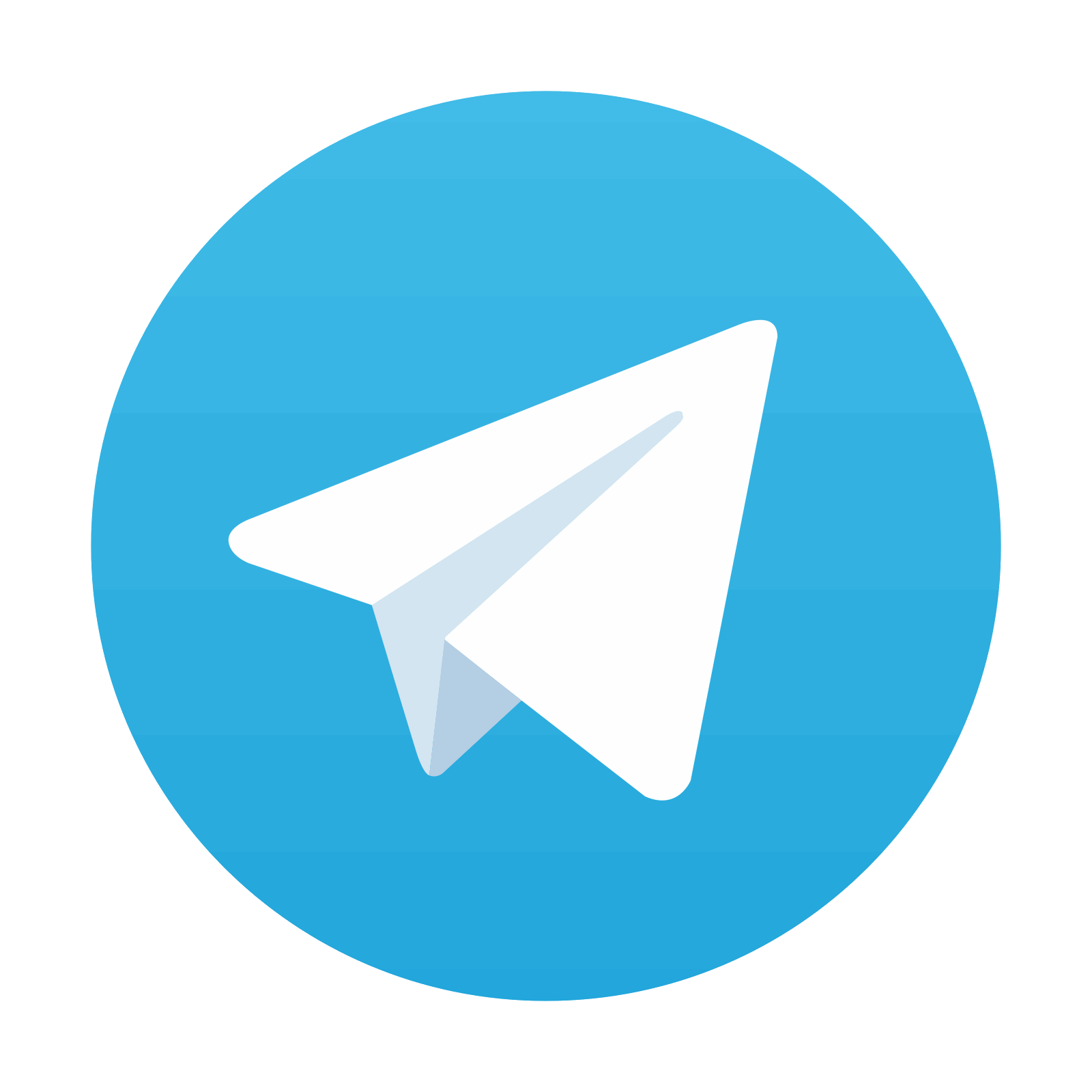
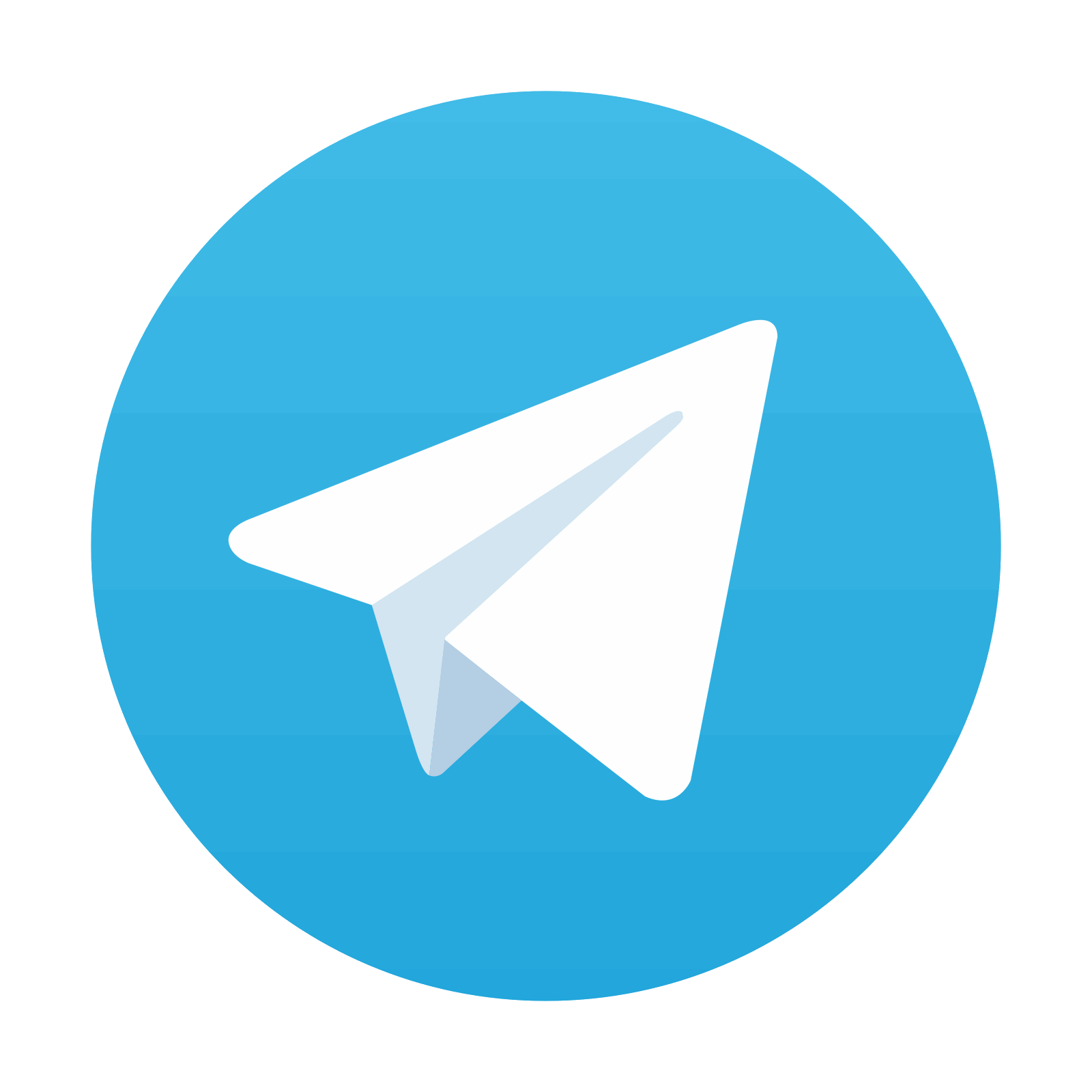
Stay updated, free articles. Join our Telegram channel

Full access? Get Clinical Tree
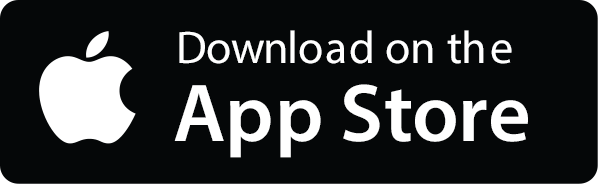
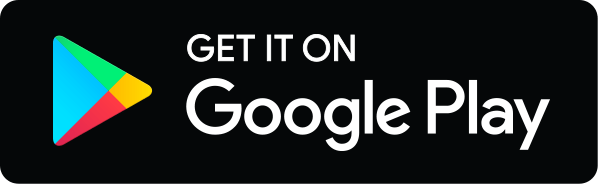