Fig. 16.1
Schematic representation of the targets and methods for the regenerative medicine in cochlear implantation. The electrode of cochlear implant was placed in the scala tympani. Neurotrophic factors, stem cells, or progenitor cells are administered to preserve or regenerate spiral ganglion cells. Growth factors are used for the preservation or regeneration of hair cells. Cells or agents are administered from the scala tympani in both treatments. IHC inner hair cell, OHC outer hair cells, SPG spiral ganglion cells
16.2 Spiral Ganglion Cells
16.2.1 Spiral Ganglion Cells as a Target of Regenerative Medicine
One of the most important factors that limit the outcome of cochlear implantation is the number of spiral ganglion cells, i.e., primary auditory neurons. Cochlear implant achieves its effects by stimulating spiral ganglion cells in the modiolus of the cochlea. Spiral ganglion cells are bipolar neurons that send two types of fibers in opposite directions. Longer central fibers, the primary auditory fibers, form the cochlear nerves and extend to the cochlear nucleus in the brainstem. Shorter peripheral fibers extend to inner and outer hair cells. The survival of spiral ganglion cells is dependent on the presence of hair cells and their release of neurotrophic factors. This explains why adult patients with short durations of deafness have better outcomes than those with long durations of deafness [1–3]. A longer duration of deafness causes more degeneration of spiral ganglion cells probably due to the lack of neurotrophic factors from hair cells [4]. Although a relationship between the number of survival spiral ganglion cells and the outcome of cochlear implantation was not found from postmortem histological studies using temporal bones of patients who underwent cochlear implantation [5, 6], 10 % of normal numbers of spiral ganglion cells were found to be necessary for successful cochlear implantation [5]. New-generation cochlear implant processing strategies that use current focusing and steering (e.g., HiRes 120 strategy) [7] may require more surviving spiral ganglion cells because current focusing and steering addresses many single electrodes and virtual sites of stimulation along the length of the cochlea. Cochlear nerve deficiency (CND) is another etiology with few numbers of spiral ganglion cells. CND is defined as an absent or hypoplastic cochlear nerve detected by magnetic resonance imaging (MRI) and/or computed tomography (CT) imaging and its prevalence is 1–5 % of bilateral sensorineural hearing loss [8, 9]. CND is one cause of congenital hearing loss. The outcome of cochlear implantation for CND cases is poorer than that for non-CND cases [10], which is different from degeneration of spiral ganglion cells in adult patients.
16.2.2 Neurotrophic Factors
Several strategies have been developed to preserve or regenerate spiral ganglion cells. One strategy is to preserve spiral ganglion cells and regenerate peripheral neural fibers toward sensory epithelia of cochleae by using neurotrophic factors [11–17] (Fig. 16.1). Preventing spiral ganglion cells from degenerating will enhance the outcome of cochlear implantation as discussed in the previous section. In addition, extending peripheral neural fibers toward sensory epithelia enhances the efficacy of cochlear implantation because cochlear implants are usually placed in the scala tympani where the electrodes cannot directly stimulate spiral ganglion cells. If peripheral fibers are regenerated from spiral ganglion neurons, direct stimulation of spiral ganglion neurons by cochlear implants will be accomplished [18].
Neurotrophic factors are produced by neurons, glial cells, sensory cells, and muscle fibers. Once neurotrophic factors bind their receptors, receptor cells show cell proliferation, cell differentiation, cell maturation, neuronal plasticity, axonal outgrowth or repulsion, and even apoptosis. Neurotrophic factors are possible factors to treat neurodegenerative diseases including Alzheimer’s disease, Parkinson’s disease, and amyotrophic lateral sclerosis through their various effects. Among several neurotrophic factors, nerve growth factor (NGF), brain-derived neurotrophic factor (BDNF), neurotrophin-3 (NT-3), and glial cell line-derived neurotrophic factor (GDNF) are predominantly functional in the cochleae. They are secreted from hair cells and they control the fate of spiral ganglion cells both in the development and adult stages. Many studies have shown that exogenous application of neurotrophic factors protected degeneration of spiral ganglion cells and induced the regeneration of peripheral neural fibers that enhanced the effects of cochlear implants. Neurotrophic factors can be delivered into cochleae in various ways other than direct infusion using pumps [11–13, 15]. Viral vectors [14, 16] and transfected cells [19] are used to deliver neurotrophic factors into the cochlea, and they provide longer and more stable application of neurotrophic factors. Even the transplantation of neural stem cells into cochleae causes the release of neurotrophic factors (GDNF and BDNF) because of differentiation of neural stem cells into glial cells [20]. BDNF delivered by biodegradable gels significantly reduced the threshold elevation of electrically evoked auditory brainstem responses that reflect the function of spiral ganglion cells [21]. Cochlear implant electrodes themselves are also candidate carriers for delivering neurotrophic factors into the cochlea. Recent studies have shown the coating of the electrode with neurotrophic factors [22] or adding the delivering channels to the electrodes [23].
16.2.3 Stem Cells and Progenitor Cells
Neurotrophic factors can protect degeneration of spiral ganglion cells and induce the regeneration of peripheral neural fibers but they do not regenerate spiral ganglion cells. To achieve the regeneration of spiral ganglion cells, several studies have investigated the transplantation of neural stem cells or neural progenitor cells that are derived from pluripotent stem cells (Fig. 16.1). Transplantation of embryonic [24] or adult [25] neural stem cells into the cochlea showed survival of transplanted cells but showed no or very little differentiation of transplanted cells into neural fate. A more efficient method is the use of progenitor cells induced from pluripotent stem cells, which in most studied cases were embryonic stem (ES) cells. The transplantation of ES cells solely did not show efficient differentiation into neurons. However, when they were transplanted with embryonic neuronal tissues, an efficient differentiation of transplanted cells into neurons was observed [26]. That study suggested the efficacy of neural induction of ES cells to achieve preferable regeneration of spiral ganglion cells. Many kinds of neural induction methods for ES cells have been described [27, 28] and those methods were applied to the transplantation of ES cells into cochleae. The results showed survival of neural cells derived from transplanted progenitors in the modiolus where spiral ganglion cells exist and functional improvement of auditory neurons was suggested [29]. Transplantation of ESC-derived otic progenitors into animals with viable hair cells and damaged spiral ganglion cells restores a population of spiral ganglion cells and auditory brainstem responses, thereby suggesting functional regeneration of spiral ganglion cells [30].
The most important problem when using neural stem cells or ES cells is the source of preparation. If the transplantation therapy is applied to the clinical situation, using neural stem cells is not realistic because those cells are prepared from embryonic or adult brain tissues. Induced pluripotent stem cells (iPSCs) that can be prepared from any tissues in the body [31] are a more suitable source of the regenerative medicine for spiral ganglion cells because iPSCs and ES cells are interchangeable.
16.3 Hair Cells
The regeneration and preservation of hair cells as well as spiral ganglion cells have become important in the clinical scene of cochlear implantation (Fig. 16.1). Since von Ilberg et al. reported the benefits of “electrical-acoustic stimulation” (EAS) for those who have residual hearing in low frequency [32], the functional preservation and even regeneration of hair cells have become important issues. The concept of EAS involves the combination of the acoustic stimulation of residual low-frequency hearing with hearing aids and the electrical stimulation of the high-frequency hearing with cochlear implants in the same ear, and this elicits more benefits, including hearing for speech in noise and music appreciation compared with the electrical stimulation only [33]. To obtain the benefits of EAS, functional hair cells are necessary. The development of soft and short cochlear implant electrodes [33–35] and minimally invasive surgical techniques [33, 36, 37] has contributed to the preservation of low-frequency hearing. However, the rate of low-frequency hearing preservation is variable [38] and sometimes it can be very low depending on the skill of surgeons. To obtain the benefits of EAS more consistently, treatment with pharmacological agents that can preserve or regenerate hair cells may need to be considered. Steroids are widely used in hearing preservation cochlear implant surgery [38] although the detailed mechanisms of hair cell protection with steroids are still unclear. Several promising reagents for the preservation or even regeneration of hair cells have also been reported. Most of them belong to the growth factor class (Fig. 16.1), including acidic fibroblast growth factor (aFGF), insulin-like growth factor-1 (IGF-1), epidermal growth factor (EGF), transforming growth factor-beta1 (TGF-beta1), and GDNF [39]. IGF-1 has protective effects of hair cells in vivo in noise-induced hearing loss [40, 41] or ischemia-induced hearing loss [42]. In vitro study using neonatal mouse cochleae revealed that the maintenance of hair cell numbers in the injured cochlea was achieved through the inhibition of apoptosis [43]. Surprisingly, IGF-1 also induced proliferation of supporting cells in the cochlea, and this contributed to the maintenance of hair cell numbers. These results suggested that IGF-1 may induce the regeneration as well as the preservation of cochlear hair cells. A clinical trial for idiopathic sudden sensorineural hearing loss that is refractory to steroid therapy showed that IGF-1 has positive effects on the recovery of hearing [44]. In both basic and clinical research, IGF-1 is proving to be one of the most preferable agents for enhancing the hearing preservation rate in the cochlear implant surgery.
References
1.
2.
Gantz BJ, Woodworth GG, Knutson JF, Abbas PJ, Tyler RS. Multivariate predictors of audiological success with multichannel cochlear implants. Ann Otol Rhinol Laryngol. 1993;102(12):909–16.PubMed
3.
Summerfield AQ, Marshall DH. Preoperative predictors of outcomes from cochlear implantation in adults: performance and quality of life. Ann Otology Rhinol Laryngol Suppl. 1995;166:105–8.
4.
Nadol Jr JB, Young YS, Glynn RJ. Survival of spiral ganglion cells in profound sensorineural hearing loss: implications for cochlear implantation. Ann Otol Rhinol Laryngol. 1989;98(6):411–6.PubMed
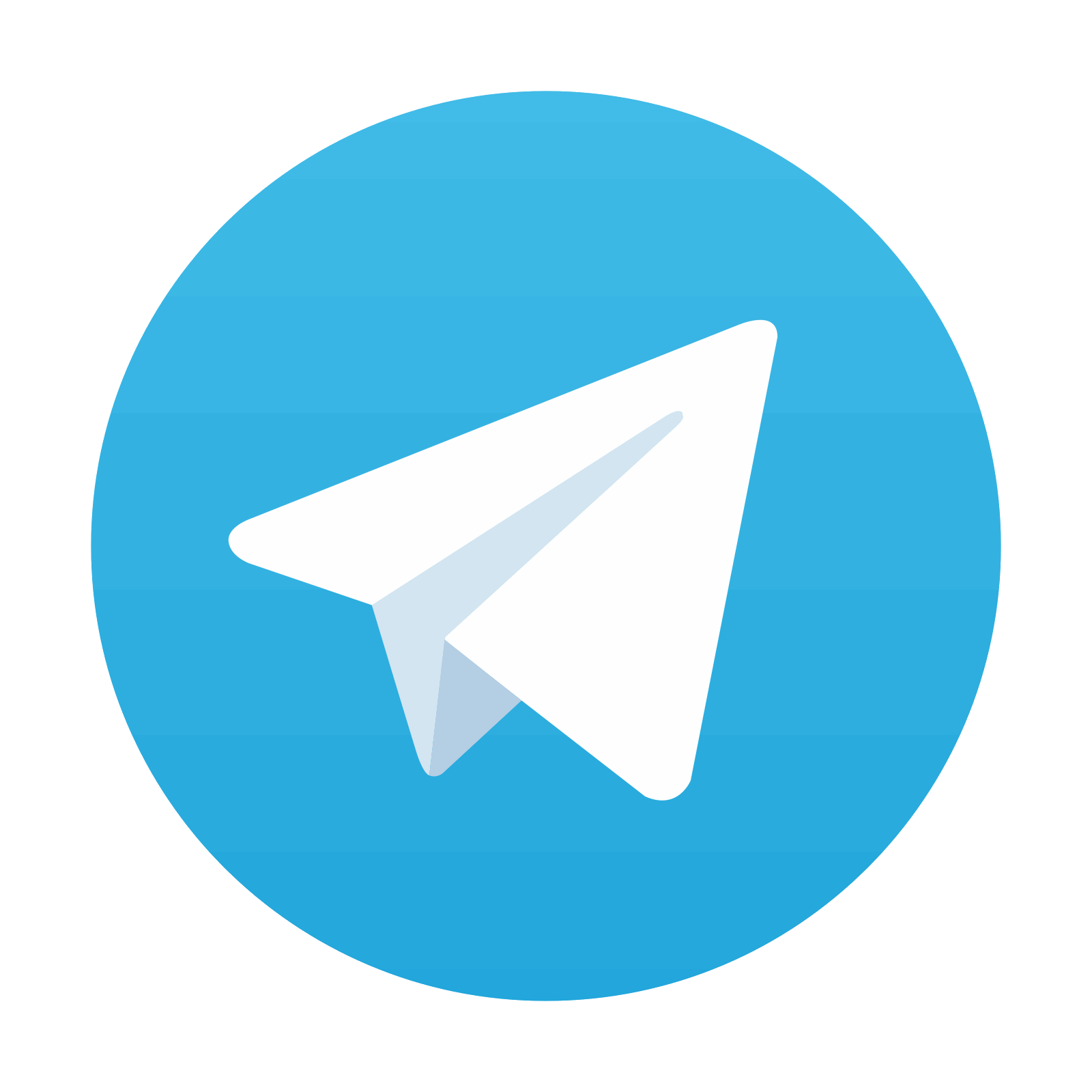
Stay updated, free articles. Join our Telegram channel

Full access? Get Clinical Tree
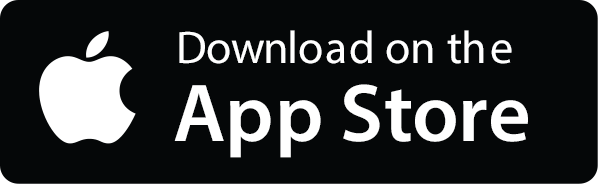
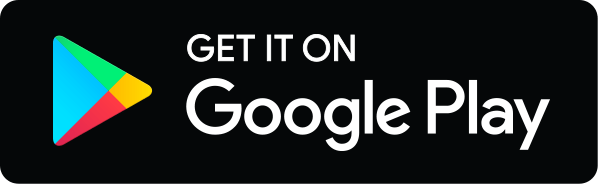