Fig. 25.1
Shows a plot of pressure and flow during respiration. As the patient inspires, the curves go downward showing a decrease in pressure and the corresponding movement of air in the direction of the lungs. As the patient changes to expiration, the curves move upward corresponding pressure increasing and the movement of air out of the nose
25.2 The Context of Rhinomanometry in Assessing Nasal Respiratory Function
25.2.1 What Are the Functions Associated with Nasal Respiration?
The movement of the diaphragm and lungs results in the movement of air through the nose. The passage of air through the nose is beneficial for the lungs because of the warming, humidification, and protective functions of the nose. The mucous layer in the nose can trap particulates, allowing the cilia to sweep them away (assessment of ciliary function covered by M. Jorissen in Chaps. 2 and 28). In addition, elements of the immune system are able to have contact with antigens, stimulating protective responses (covered by R. Kern in Chap. 3). Furthermore, air is delivered to the olfactory area providing the enhancement of taste and the protective function of detecting potentially harmful substances or organisms (assessment covered by P. Rombaux in Chaps. 10 and 30). The measurement of some of the important physiologic components of nasal respiration, warming, humidification, mucociliary clearance, and olfaction, is covered in other chapters in this book as noted above.
As air passes through the nose, comfortable nasal breathing corresponds with a dimension and shape of the nasal airway that in general optimizes the above functions. Rhinomanometry assesses the overall effect of nasal airway dimension and shape on the passage of air through the nose.
Comfortable nasal breathing corresponds with a dimension and shape of the nasal airway that in general optimizes the multiple functions of the nose.
25.2.2 What Anatomic Elements Are Encountered During Nasal Respiration?
Measurement of the nasal airway assesses the nasal airstream which may be variably affected by the physical dimension of the components of the nasal airway including the vestibule, valve area, turbinates, and sinus openings. The presentation of these anatomic elements as air is inspired through the nose is different than the shape of the presenting surfaces as air is expired. This may reflect different functions of the two phases.
The first part of inspiration is the passage of air through the vestibule in a curve first superiorly and then toward the nasal valve area. The airstream then passes through a narrower area referred to as the internal valve. This corresponds to the area under the caudal end of the upper lateral cartilages and is clearly seen on casts or volume renderings of the nasal airway (Fig. 25.2). As the airstream leaves the valve area, it is dispersed in a wider distribution, thus providing contact with more surface area of the turbinates than if the airstream had passed unimpeded. The turbinates provide the working surface of the nose. By having protuberant curved surfaces, they act as flanges in the airstream providing increased surface areas on which to contact the air for humidification, warming, and filtering. The air then passes on to the choana and turns again, this time in the direction of the larynx and tracheobronchial tree.
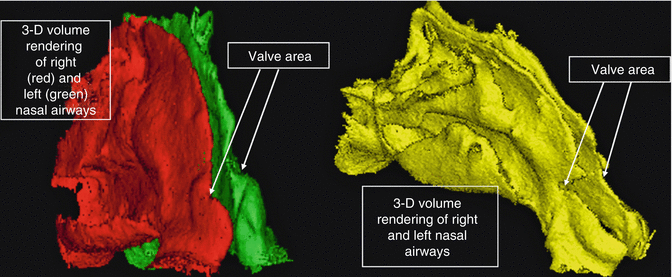
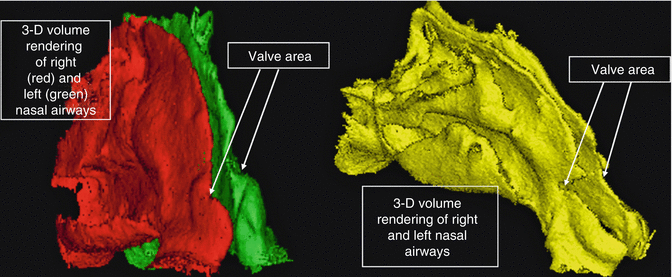
Fig. 25.2
Volume rendering of the nasal airway. Using 3D image analysis software and CT scans, the nasal airway is portrayed. This gives the same image early anatomists accomplished with wax castings. Note the narrow part of the airway at the valve area
As the inspired airstream passes the uncinate, the natural os of the maxillary sinus is protected from exposure to the passing airstream. Openings from the frontal sinus, anterior ethmoid cells, and posterior ethmoid cells are similarly sheltered by presenting baffles though maxillary sinus accessory openings and postsurgical openings can present additional openings to the inspired air. Computational fluid dynamics (CFD) studies have suggested only minimal, if any, effect of single maxillary sinus openings on the respiratory airstream in a typical (unoperated) nose, whereas the presence of an accessory os can result in some airflow into the maxillary sinus (Hood et al. 2009; Zhu et al. 2012).
When considering any possible impact of the nasal passage during expiration, retention of heat is usually mentioned. This may be facilitated by having the air pass over the turbinates before encountering the restriction of the valve area. The baffles that sheltered the ostia in the inspiratory direction now may function to catch the expired air. Some associate this phenomenon with nitric oxide from the maxillary sinus serving a regulatory function in respiration (for measurement of nitric oxide, see Chap. 9 by P. Hellings). Computational fluid dynamics (CFD; see below) in 3D models showed an increase in maxillary sinus airflow rate with high expiratory flow rates simulating nose blowing (Zhu et al. 2012).
As the airstream leaves the valve area, it is dispersed in a broader distribution, thus providing contact with more surface area of the turbinates than if the airstream had passed unimpeded.
25.2.3 Objective Measurement of Nasal Respiratory Function
Of the various anatomic elements present, measurements of the airstream are primarily influenced by the dimension of the valve and turbinates, by the relative thickness of the mucosal lining, and, at times, by the respiratory effort of the patient.
The objective methods of assessing the nasal respiratory passages include (a) measurement of the dimension of the airway, (b) measurement of the nasal airflow alone, and (c) rhinomanometry, the simultaneous measurement of the transnasal pressure and airflow.
25.2.3.1 Measurement of the Dimension of the Airway
The assessments of the dimensions of the nasal airway are not measurements of the airflow through the nose during respiration. Imaging with CT or MRI and then doing a 3D reconstruction of the airway will demonstrate the airway dimensions in different parts of the nose. This can be helpful especially when used in conjunction with computational fluid dynamics (CFD).
Acoustic rhinometry (see Chap. 26 by E. Hizal and O. Cakmak) also measures the airway dimension by calculations done on sound waves reflected back by intranasal structures. While this is not an assessment of the flow of air through the nose, it can be useful for measuring relative airway dimensions as well as changes with time, treatment, or various interventions (Lal et al. 2006).
Computational fluid dynamics (CFD) uses imaging, typically a CT scan done at one point in time, to generate a 3D model of the airway and then apply fluid dynamic modeling to that airway (Zhao and Dalton 2007). By using different transnasal pressures representing a respiratory cycle, the software can calculate the relative flow velocity in a number of anatomic sites in the nasal airway for various points in time during respiration. This capability offers exciting possibilities for the future study of the impact of various anatomic variations or pathology on the airstream. Those studying nasal physiology will be faced with the task of finding the meaning of the plethora of different flow vectors that result from CFD analysis of the nasal airway. To identify the meaningful parameters derived from the large amount of data is a tantalizing possibility for future study that will be facilitated by ever increasing computer processing speed and data handling (see Chap. 19 by R. Mösges).
25.2.3.2 Measurement of the Nasal Airflow Alone (Peak Flow Measurement)
Measuring only nasal airflow is popular especially with physicians who already use similar equipment to monitor their asthmatic patients by measuring peak lower airway flows. While this has the limitations of some dependence on patient effort, it has been relatively popular because of its simplicity and the ready availability of the equipment (Timperley et al. 2011). It has been demonstrated that physicians would like to have a simple tool for objective assessment of results in allergic rhinitis (Serrano et al. 2007). Since the rate of flow changes throughout the respiratory cycle, taking the measurement at some constant point can help decrease the variability of results and provide a standard for comparison. In this case, the “constant” point is the “peak” airflow reached with maximal effort. Peak nasal inspiratory flow can be measured by modifying the peak flow device for nasal inspiration. The measurements can be affected by valve collapse occurring at higher airflows that may not occur at normal physiologic flows (Barnes and Lipworth 2007). Nonetheless, this method has been popular, and normative values have been collected (Ottaviano et al. 2006; Papachristou et al. 2008; da Cunha Ibiapina et al. 2011; van Spronsen et al. 2012).
Both peak nasal inspiratory flow (PNIF) and peak nasal expiratory flow (PNEF) measurements have been used. There is some debate about the variability of results (Blomgren et al. 2003; Teixeira et al. 2011), but the tests have been shown to be useful, particularly for challenge testing in patients with allergic rhinitis (Wilson et al. 2003).
25.2.3.3 Rhinomanometry: The Simultaneous Measurement of the Transnasal Pressure and Airflow
Collecting simultaneous pressure and flow values allows the calculation of nasal resistance or conductance. Calculation of the ratio of pressure to flow could be done at any one of many simultaneous pressure-flow values along the continuously changing curve during respiration (Fig. 25.3). Using a specific airflow value at which to measure the pressure-flow values is an important element allowing consistent comparisons. Viewing the entire sigmoid pressure-flow curve also allows the observation of the position and amount of curvature that reflects the amount of flow the patient is generating for the range of pressures occurring in the course of their nasal breathing. Rhinomanometry is used (except in rare studies) to assess the pressure and flow across the entire nasal airway, from nasal entrance to nasopharynx.
The use of CFD analyses done from CT images inspires thoughts of using microsensors to inobtrusively detect the pressure and flow changes during respiration for multiple sites in the nasal airway. Just as Lindemann (Lindemann et al. 2006) had actual measurements using tiny thermocouples to validate the corresponding CFD calculations they did for temperature at many sites in the nose, multiple localized pressure and flow measurements could verify the results of CFD analyses that yield multiple differing flow vectors at different anatomic sites in the nasal airway. Such validation of CFD, if combined with actual pressure measurements for a given patient, could move it out of the category of assessing airway dimensions to the category of yielding measured information about nasal airflow.
The plot of pressure and flow during inspiration and expiration yields a sigmoid curve that is closer to the x-axis (pressure axis) when nasal obstruction is greater.
25.2.4 Rhinomanometry for Measurement of Nasal Respiratory Function
As noted in the introduction, when rhinomanometry is performed, continuous measurement of transnasal pressure shows a rising and falling curve in the positive and then negative direction throughout each respiratory cycle (Fig. 25.1). As the changing pressure drives an accelerating and then decelerating flow of air, a plot of airflow shows a similar positive and negative excursion. Plotting pressure (x-axis) versus flow (y-axis) during inspiration and expiration yields a sigmoid curve that is closer to the x-axis when obstruction is greater (Figs. 25.3 and 25.4). Vogt pointed out that the path of the pressure-flow curve away from the origin during inspiration (the accelerating limb) often does not follow the exact same curve on the path back to the origin (decelerating phase). The same is true for the expiratory limb (Fig. 25.5).
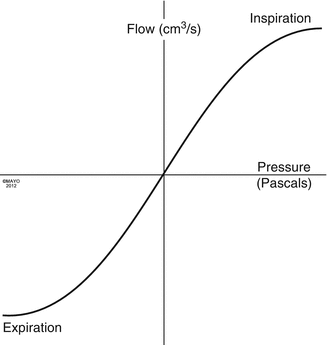
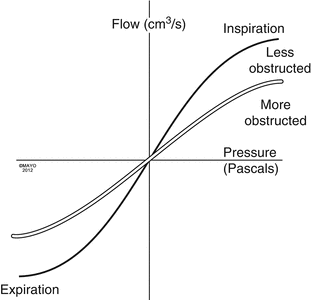
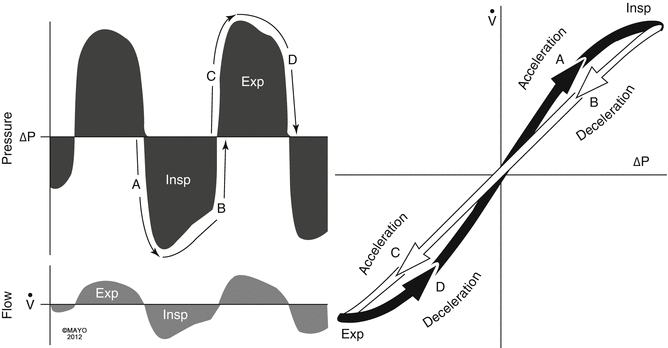
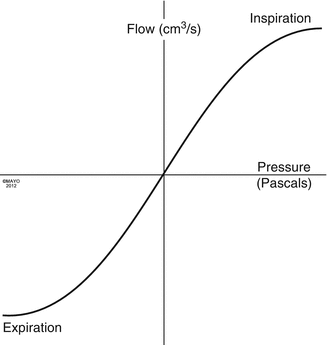
Fig. 25.3
The plot of pressure versus flow. Each point represents the simultaneous measurement of pressure and the corresponding flow value. Pressure values are on the x-axis and flow values on the y-axis. The sigmoid shape of the curve shows that in general there is a gradual increase in the pressure to flow ratio as one goes further out the curve toward the maximum values reached in normal respiration. Thus, for a given patient, the resistance value reported can vary depending on the point on the curve that is selected for calculating the result
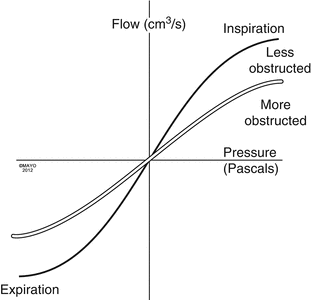
Fig. 25.4
The sigmoid pressure-flow curves for two different patients. The curve that is closer to the x-axis (pressure) represents the more obstructed nasal airway with higher resistance values
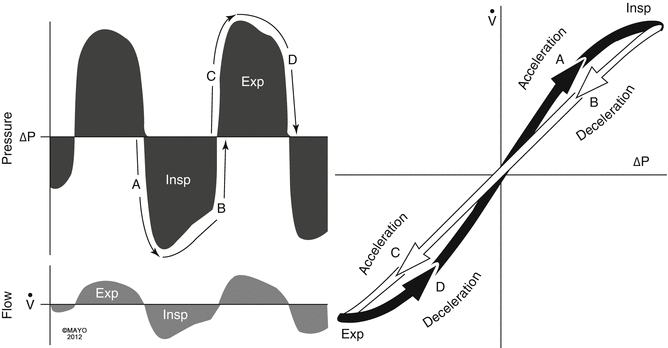
Fig. 25.5
The path of the pressure-flow curve away from the origin during inspiration (the accelerating limb, A) does not follow the same curve on the path back to the origin (decelerating phase, B). The same is true for the expiratory limb (C, D)
25.3 How Is the Measurement Done with Rhinomanometry?
25.3.1 Different Techniques: Most Common Method
The most commonly employed method of doing rhinomanometry is called anterior masked rhinomanometry. The different methods of rhinomanometry are distinguished by the location of the pressure detection and the apparatus for flow measurement. Table 25.1 lists the different types (methods) of rhinomanometry and the methods of pressure and flow detection that define them.
For measurement of the nasal airway of a patient with a nasal septal perforation, only the total airway is measured because the septal perforation would cause an error in unilateral pressure assessment. Anterior rhinomanometry thus is not used in patients with nasal septal perforations. Since the total airway is not measured when the anterior method is used, it is necessary to derive the total airway values by adding the right and left flow values for each corresponding pressure value along the pressure-flow curve (Fig. 25.6).
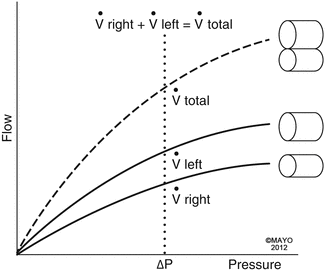
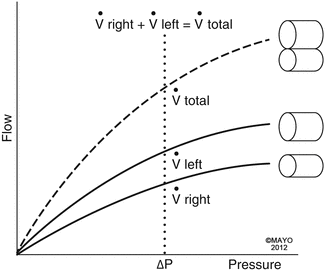
Fig. 25.6
For a given pressure value, the total flow is equal to the right-sided flow plus the left-sided flow, both measured at that pressure value. Flows are only additive if measured at the same pressure. This is analogous to two electrical currents being additive at the same voltages
Table 25.1
The different types of rhinomanometry. Anterior masked rhinomanometry is the type most commonly employed
Type of rhinomanometry | Flow detection | Pressure detection | Side(s) that can be directly measured |
---|---|---|---|
Anterior masked with full face mask | Device on outlet of full face mask | Catheter with sealed connection to non-measured nostril | Unilateral |
Anterior masked with partial face mask | Device on outlet of partial face mask | Catheter with sealed connection to non-measured nostril | Unilateral |
Anterior with nozzle | Device connected to nozzle held to nostril opening | Nozzle held to non-measured nostril | Unilateral |
Posterior with full face mask | Device on outlet of full face mask | Catheter by nasopharynx – either transoral or transnasal | Total or unilateral |
Posterior with partial face mask | Device on outlet of partial face mask | Catheter by nasopharynx – either transoral or transnasal | Total or unilateral |
Body plethysmograph | Movement of chest inside body plethysmograph | Posterior catheter by nasopharynx – either transoral or transnasal or anterior catheter to non-measured nostril | Total or unilateral |
25.3.2 Transnasal Flow Measurement: Anterior or Posterior Method
Flow through the nasal airway is most commonly measured by attaching a flowmeter at the outlet of the mask which is sealed tightly on the patient’s face. The usual flowmeter consists of pressure detection on either side of a resistive element. Originally nozzles were used to measure the flow through each nostril. When using a mask (or body plethysmograph), unilateral measurements can be done by occluding the opposite nostril with tape.
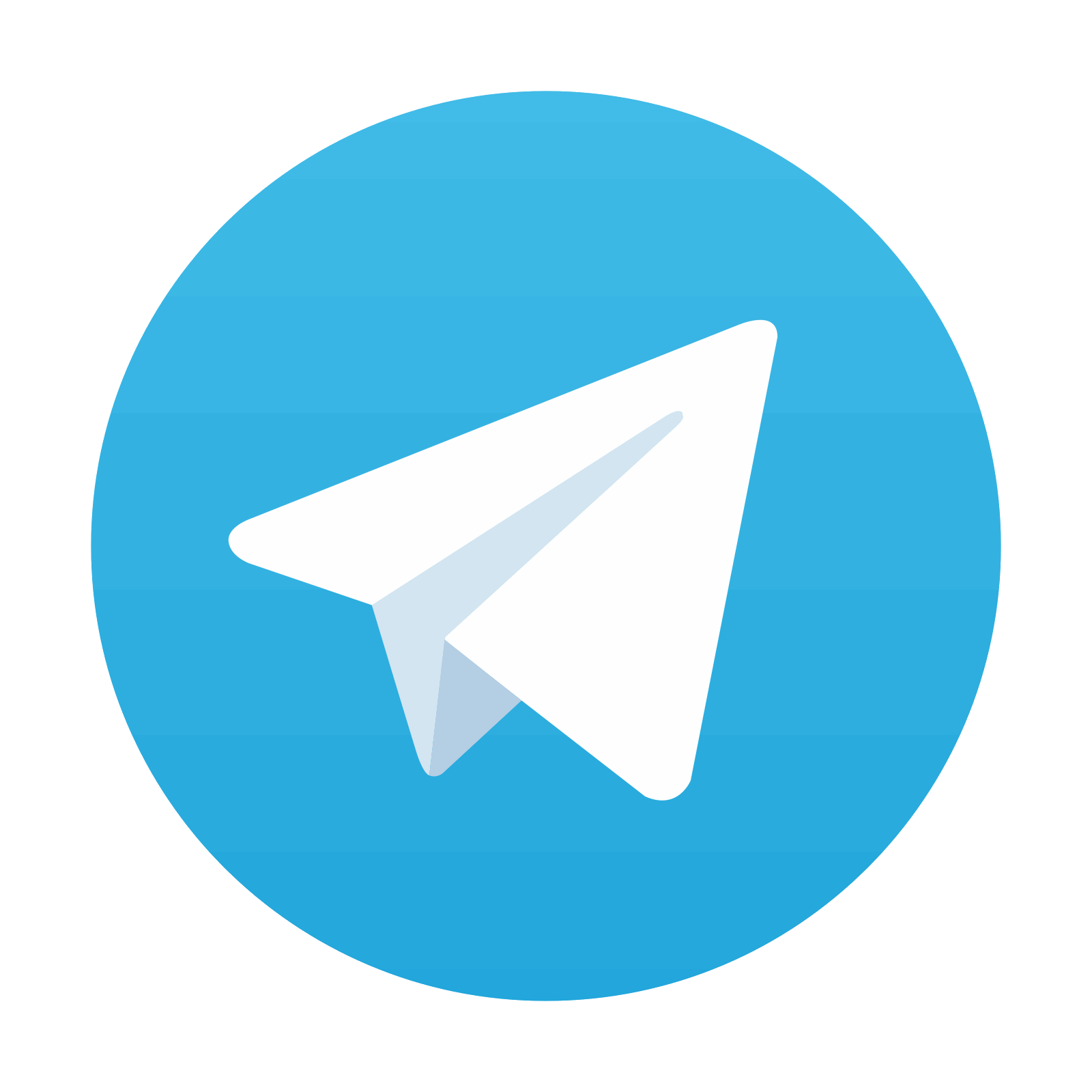
Stay updated, free articles. Join our Telegram channel

Full access? Get Clinical Tree
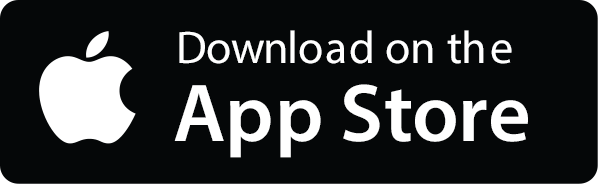
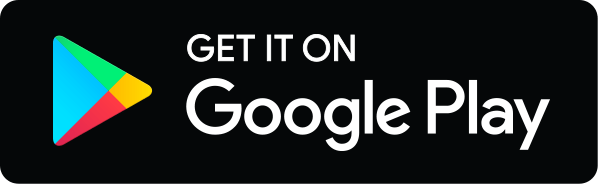