In the sixth fetal month, ingrowth of connective tissue divides the cartilaginous nasal capsule into the individual septal cartilage, lateral cartilages and alar cartilages. Thus, the complex cartilaginous nasal portal is developed which later forms the cartilaginous framework of the mobile nose.
In the model of the fetal cartilaginous capsule aged 30–32 weeks (Fig. 34.2), we look on the two vaults of the lateral cartilages fused together with the septal cartilage in the supraseptal groove. The median portion bifida septi remains as the rest of the fusion of the vaults. Two semicircular-shaped cartilaginous bars have been almost completely separated from the caudal septal cartilage. They encompass the nasal vestibule medially, dorsally and laterally to form the definite alar cartilages. In the medial upper angle of the alar cartilage, an isolated piece of cartilage is visible on the right-hand side, while on the left-hand side, it is still a process in connection with the left sidewall. Later, these pieces become the sesamoid cartilages. The same happens to small processes of the lateral sidewall which are concealed by the semicircular cartilage in Fig. 34.2. The paraseptal cartilages are concealed by the caudal part of the semicircular cartilages. The triangular cartilages are not yet differentiated. In the newborn, they are still continuous with the cartilaginous anterior skull base. Their caudal margins are firmly connected to the alar cartilages and to the piriform aperture. In the newborn, the alar cartilages are developed as separate structures as in the adult and overlap with their cranial margins and the caudal margins of the triangular cartilages.
34.1.3 34.1.3 Postnatal Development
The septal cartilage of the newborn is progressively transformed from posteriorly, cranially and caudally into a unit of posterior bone and remaining anterior quadrangular cartilaginous plate as clearly depicted by Virchow in 1857 (Virchow 1857). This old knowledge has recently been supported by Kim et al. (2010) who evaluated the anatomical correlations among components of the nasal septum using computed tomography. They found again that the area of the cartilaginous septum decreases with age, while the area of the perpendicular plate increases with age at the expense of the area of the septal cartilage. However, the area of the total nasal septum remains constant.
In the first decade of life, the triangular cartilages show regression from the cephalic to the caudal end under the nasal bones until being transformed in the approximately triangular shape of the adult nose. Only a small cartilaginous remnant of the triangular cartilage remains overlapped by the caudal margin of the nasal bone. Caudally, the triangular cartilages are overlapped by the cranial margins of the alar cartilages.
Very rarely the regression process of the triangular cartilage is retarded or even impeded. This may happen in case of a median nasal fistula or of a nasal dermoid cyst which can cranially end at the crista galli. Nasal bifidity is another congenital malformation with impeded regression of the triangular cartilages.
Potter et al. (2000) studied 35 adult, white cadaveric specimens and specially focused on the caudal attachment of the triangular cartilage to the septal cartilage, where usually a small cleft facilitates mobility between the caudal edge of the triangular cartilage and the septum, the so-called weak triangle of Converse. The attachment ranged from no cartilaginous connection (68 %) to complete fusion (32 %), i.e. a coincident location of the anterior septal angle and the caudal edge of the triangular cartilage.
In the adult, the relationship between the triangular cartilage and the lateral crus of the alar cartilage shows four variations (Dion et al. 1978). Most often Dion et al. found an overlap of the caudal margin of the triangular cartilage by the cranial margin of the lateral crus. Less frequently are the relationships ‘end to end’, ‘scroll’ and ‘opposed scroll’.
Each cartilage of the cartilaginous vault is encased in its own fibrous capsule, whose fibres decussate, form a fibrous band or aponeurosis and join the capsule of an adjacent cartilage. This aponeurosis, acting as a flexible membrane, allows freedom of movement between the neighbouring cartilages. According to Hinderer (1971), the most distinctive fibres are:
1.
Those between the terminal ends of the triangular cartilage and septum that supply the mobility necessary for valve action between these two structures
2.
Those between the caudal end of the septum and the medial crura of the columella which form the membranous septum
3.
Those between the caudal margin of the triangular cartilages and the cranial border of the alar cartilages
A permanent continuity between the encasing fibrous capsule of the triangular cartilage and the periosteum of the nasal bone was found by Bruintjes et al. (1998), supporting the clinical observation of the firm connection between the nasal bones and the triangular cartilages.
Additional mobility of the interacting nasal cartilages is provided by the thin layer of seven muscles covering the external nasal pyramid. Besides their mimic function, some muscles act as dilators of the valve region or openers of the nostrils or provide stability for the lateral nasal wall (Bruintjes et al. 1996). Especially, the dilator naris accompanies each nasal inspiration, thus directly varying with ventilation, nasal resistance, hypoxia and hypercapnia. It stabilises the anterior nasal airway and precedes diaphragmatic contractions and ceases to act with mouth or tracheostomal breathing (Cole 1993).
The framework of nasal cartilages with their fibrous connections and covering muscle layer acts as shield and portal to the erectile lining of the nasal cavities. Eugene Kern from Rochester, USA, termed the nasal mucosa ‘the organ of the nose’ to sum up all its many functional tasks which are described in other chapters of this book. While the nasal vestibule is covered with squamous epithelium continuing some millimetres around the edge of the caudal margin of the triangular cartilages, the nasal mucosa with ciliated cylindrical epithelium starts in the posterior valve region (Bachmann and Legler 1972; Wustrow 1951). Figure 34.3 shows the histological section through the posterior valve region of a newborn. The heads of the inferior turbinates catch our eyes as elevations from the mucosal lining of the lateral walls. The slightly deviated cartilaginous septum with its mucosal lining presents its thickening in the middle covered by the thick pad of erectile mucosa, the septal body or intumescentia septi. The different thickness of the vertically cut septal cartilage is clearly visible: the thick cranial part merges caudally with a thin segment to end in the broad deviated cartilaginous foot embedded in the premaxillary bone. This pattern of different cartilaginous thickness remains persistent throughout lifetime as investigated by van Loosen et al. (2000).
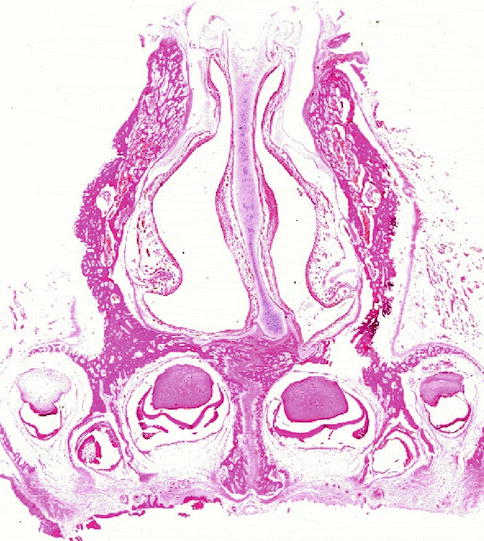
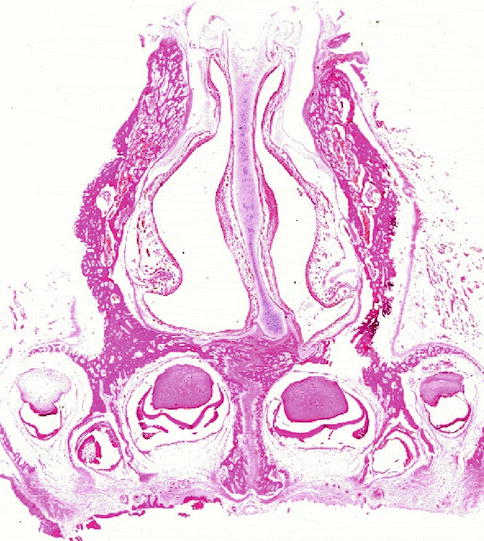
Fig. 34.3
Section through the posterior valve region of a newborn cadaver specimen. Note heads of the inferior turbinates, deviated anterior septal cartilage with septal body and asymmetric maxilla. Hematoxylin-eosin (gift of Lindsay Gray/Perth to author 1975)
34.2 34.2 Part II: Functional Aspects
In this book, nasal functions like breathing, resistance, turbulence and nasal cycle are treated in special chapters in detail. Thus, I can confine to a few comments on functions where nasal cartilages are essentially involved like in the nasal valve region or the vulnerable anterior septum.
The anterior septodorsal cartilage, the paraseptal and the alar cartilages with the sesamoid cartilages form the framework of the nasal lobule and thus the portal to the upper airways. Soft tissue connections between the cartilages of varying thickness and several small muscles acting on their outside enable mobility and/or stability of the lateral nasal walls. Thus, the entrance to the nose can be dilated and narrowed to modify the inspiratory and expiratory airstreams. The entrance of the airflow is comparable with two flat, oval, hollowed structures, the nasal vestibules, which terminate in the aperture between the septum and the caudal end of the triangular cartilages, both parts of the nasal valve region. The vestibule is at an oblique angle to the cavum (Bachmann and Legler 1972). According to Cole’s studies (Cole 1993, 2003) the nasal valve region consists of four anatomical airflow-resistive components: the aperture between septum and triangular cartilage, the bony entrance to the nasal cavum which is occupied by erectile tissues of both lateral (head of inferior turbinate) and septal nasal walls (including septal body) that modulate the cross-sectional area of the airway and airflow resistance. Two-thirds of the total nasal resistance during inspiration is provided by the nasal valve region. This has clearly been proved by Haight and Cole (1983) using pressure-sensing cannulas. As two-thirds of the total airway resistance to breathing is created in the nose – one-third is provided by the open mouth (Swift et al. 1988) – the nasal valve region is the main resistor of the total airway. The narrow nasal valve region with the smallest cross-sectional area of the nasal cavum accelerates the inspired air, creating turbulence. Valve constrictions disrupt the laminar characteristics of the inspired air as it enters the body of the cavum and thereby enhance exchanges with the nasal mucosa of heat, water and noxious materials. In the widened nasal cavum, airflow decreases its speed (Cole 2003).
In clinical praxis the physician has to face several patients complaining of breathing problems which are caused by a disturbed anatomy of the nasal valve region, especially patients with a tension nose or a saddle nose. A patient with a tension nose presents with a prominent, curved, small dorsum due to a too large and too high septum. Other characteristics are the increased projection of the tip, slitlike nostrils with an elongated columella and elongated thin nasal alae. The feet of the medial crura are cranially shifted, thus broadening the base of columella. The nasolabial angle is enlarged and often the upper incisivi are showing. The valve angle is less than 10° which results in bilateral alar collapse during inspiration.
In the cartilaginous saddle nose, the septum is too short mostly due to fractures or perforations. The caudal septodorsal cartilage is depressed which causes an enlargement of the valve angle to more than 20°. The nostrils with ballooning alae are elliptically distorted; the columella is shortened and often retracted. Although the nasal cavities look very wide, the main complaint of the patients is insufficient breathing due to too much turbulence of the airstreams.
The fact that the nasal septum divides the nose in two parallel halves is the condition for cyclic activities of the erectile nasal mucosa. Kayser in 1895 (Kayser 1895) first reported on the spontaneous, cyclical congestion and decongestion in the nasal cavities. The anatomic conditions for this mucosal behaviour are mainly provided by its capacitance vessels. The ‘working phase’ of one nasal cavity characterised by decongestion of the cavernous tissues alternates with the ‘resting phase’ characterised by congestion of the mucosa (Cole 2003; Hasegawa and Kern 1978; Lang et al. 2003). Changes of flow and resistance during the nasal cycle have been studied using rhinomanometry (Hasegawa and Kern 1978; Stoksted 1953). By means of acoustic rhinometry, Fisher et al. (1993) measured the changes of the cross-sectional areas in the nasal cavities due to congestion and decongestion.
Using the combination of endoscopic imaging, rhinomanometry and acoustic rhinometry, Lang et al. (2003) observed a periodic change in turbulence behaviour in addition to the known cyclical changes of flow resistance and nasal width. In the resting phase, mainly laminar flow was found. During the working phase, the onset of turbulence occurred already at low velocities. The increase of turbulence during the working phase was created by the increase in cross-sectional area in the anterior cavum due to decongestion of the mucosa of the head of the inferior turbinate and the septal body.
The nasal cartilages are insofar involved in the process of the nasal cycle as they provide the anatomical trigger zones for the transition from laminar to turbulent airflow, namely, the anterior nasal cavum between the nasal valve region and the head of the middle turbinate.
Bruintjes et al. (1998) studied the kinematics of the lateral nasal wall which is made up of three parts: (a) the osseous-cartilaginous chain of nasal bone, triangular cartilage and the lateral crus of the alar cartilage; (b) the hinge area with the sesamoid cartilages between the lateral piriform aperture and the lateral margin of the lateral crus; and (c) the ala, the part between piriform aperture and alar cartilage, not supported by cartilage. While part (a) is relatively stable and part (b) much more compliant, they found part (c) being the most compliant part of the lateral nasal wall. They also studied the muscles influencing the lateral nasal wall and its compliance. With this knowledge of the mechanical properties of the lateral nasal wall, they were able to analyse pathological clinical conditions, which may occur at the level of the nasal valve and at the level of the vestibule or nostril. Thus, they could explain, for instance, the physiological alterations caused by facial nerve palsy where muscle denervation may lead to alar collapse (May et al. 1977).
Loss of alar stability with nasal obstruction during inspiration is often caused by inadequate surgical procedures for the nasal lobule. Mostly the continuity of the osseous-cartilaginous chain, ‘nasal bone-triangular cartilage-lateral crus of alar cartilage’, is destroyed (Kasperbauer and Kern 1987). Detailed knowledge of these anatomical connections could help to reduce such adverse surgical sequelae.
The alar collapse associated with the drooping tip of elderly people may result from nasal muscle atrophy, a change in cartilage resilience and stretching of the intercartilaginous fibrous tissue with loss of cartilage overlap in the intercartilaginous junction (Krmpotic-Nemanic et al. 1971). The surgical procedure of the so-called rhinolift could reduce the breathing problems of the elderly people. Via intercartilaginous incisions in the limen nasi, the cephalic margins of the lateral alar crura are bilaterally partly resected. After elevating the dorsal skin of the nasal pyramid and excision of an oval piece of skin in the nasal root, both mobilised alar cartilages can be lifted and fixed, thus widening the angles of the nasal valves.
The elastic nasal cartilages are useful elements for protective functions. The 9-year-old boy in Fig. 34.12 is a good example to explain the function of the septal cartilages as a ‘crumpled zone’ in case of severe anteroposterior load. Between 1970 and 1972, I performed septorhinoplasty according to the techniques of Cottle, Goldman and Masing in 92 children with a mean age of 10.5 years (Pirsig and Knahl 1974). The indication was obstructed nasal breathing due to established post-traumatic nasal deformities. Intraoperatively, fractures and defects were visible in the anterior septodorsal cartilage with involvement of the nasal valves and sometimes of alar cartilages in 44/92 noses. These noses were damaged by loads from frontal and/or below. 23/92 noses showed fractures in the septodorsal cartilage like the previous group and in addition fractures of the nasal bones and perpendicular plate caused by mainly anteroposterior load. This means that 67/99 or 72 % of the children suffered nasal obstruction due to damaged nasal cartilages in the anterior nose.
The build-up of the septal cartilage in regions of different thickness (van Loosen et al. 2000) through the whole life is one component to react more elastically to front load. A second component of more compliance is the vaultlike construction of the septodorsal and alar cartilages with their joint-like fibrous interconnections (Bruintjes et al. 1998). Note the distortion of the caudal septodorsal cartilage of the boy in Fig. 34.12 (3): a 90° angle of the caudal septum with fractures in the caudal edge and depressed edges of the triangular cartilages. Van Velzen et al. (1997) published the prepared septal cartilage of a 4-year-old boy who died in a frontal accident. The fracture lines in the cartilaginous septum followed the thin regions of the cartilage as sites of minor resistance in reaction to load.
In idealised and patient-specific models, Lee et al. (2010) recently published the reactions of the human septal cartilage exposed to anteroposterior load. They found the maximum stress areas in the nasal septum in the vicinity of the bony-cartilaginous junction and the anterior nasal spine, which are consistent with clinical experience. The findings of their study also suggest that the septum does function as a ‘crumpled zone’, absorbing a significant amount of stress before it is transmitted to the skull.
The extreme variant of a damaged crumpled zone nose is the classic boxer’s nose, the so-called rubber nose, mainly formed by the alar cartilages. The septodorsal cartilage is shrunken to a minimum; nasal bones and anterior nasal spine are pressed down to the level of the piriform aperture or resorbed.
34.3 34.3 Part III: Alterations of Nasal Cartilages
The functions of the nasal cartilages can best be recognised in children and adults with a disturbed cartilaginous framework. The growing nose is influenced by genetic and epigenetic influences such as oxygen supply, nutrition, hormones, medication, infections and injuries including nasal surgery as a controlled trauma, to name a few. In this part, examples are presented describing some long-term influences on the nasal cartilages citing literature and own case reports.
34.3.1 34.3.1 Lacking Septodorsal Cartilage
Already in utero, the septodorsal cartilage, being composed of the septal and both triangular cartilages, develops as the dominating structure for nasal and midfacial growth. This was shown in 1791 by Soemmerring (Soemmerring 1791) who published a newborn’s skull with a lacking septodorsal cartilage and the skull of a healthy newborn for comparison (Fig. 34.4). In the deformed newborn, the nasal bones and the incisive parts of the maxilla are not developed. The size of the piriform aperture is reduced to one-third in width compared with the healthy newborn. Height and width of the maxilla are reduced, while the contours of the orbital cavities are distorted compared with the healthy newborn.
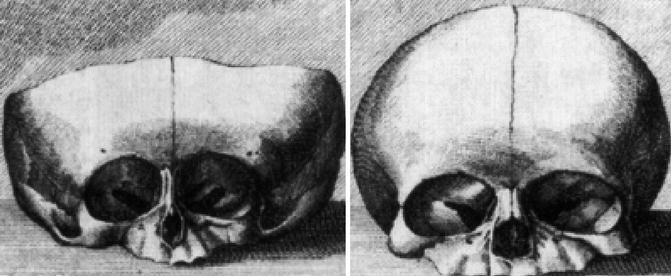
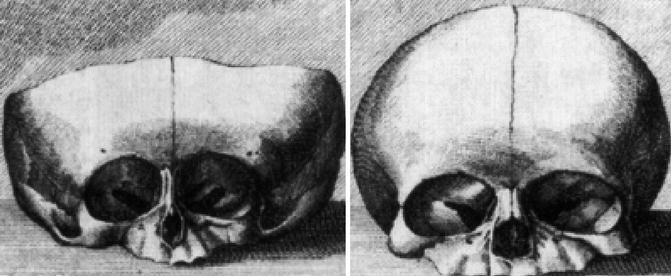
Fig. 34.4
Skulls of newborns with lacking septodorsal cartilage (left), with normal midface (right) (Soemmerring 1791)
34.3.2 34.3.2 Lacking Alar Cartilages
The following case shows that congenitally lacking of both alar cartilages did not impede the growth of nose and midface apart from the complete lacking nasal lobule (Fig. 34.5). The 15-year-old boy came from a family with no genetic nasal disorders and an uneventful pregnancy of his mother. There was a complete absence of both alar cartilages. The pseudo-columella was formed by skin covering the caudal septal cartilage. The caudal margins of the triangular cartilages were covered by thick skin on the right and thin skin on the left side. All the other nasal structures were inconspicuous. The boy’s breathing was normal (Pirsig 1989).
34.3.3 34.3.3 Physiological Septal Deviation
The anatomical term ‘physiological septal deviation’ was introduced by Zuckerkandl (1882) who defined this type of septal deformation as a bended septum within the asymmetrical human skull. He published several examples on beautiful lithographs (Fig. 34.6). Comparable histologic sections of his own cases were published by Gray (1978) who kindly left me a few of them like in Fig. 34.3. In more recent publications (Gogniashvili et al. 2011; Mlynski 2005) on this topic, the incidence of the physiological septal deviation is reported between 30 and 75 % for children and between 13 and 96 % in adults, with strikingly less patients reporting to suffer from subjective symptoms (Hanif et al. 2003). From the physiological point of view, it is practical to characterise the physiological septal deviation by normal endonasal resistance (Gogniashvili et al. 2011). Many of these physiological septal deviations develop during the fetal life in connection with the asymmetrical maxillary growth. Often the non-pathological role of this deviation is not recognised and a septal operation is indicated, although it is useless or even worsens the nasal symptoms. A septal body may be mistaken as pathological septal deviation or the real cause of nasal obstruction such as a problem of the nasal valve or an inspiratory ala collapse is failed to be recognised.
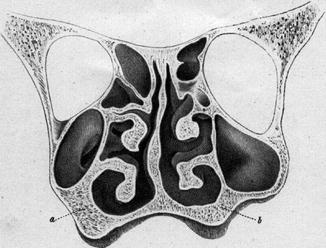
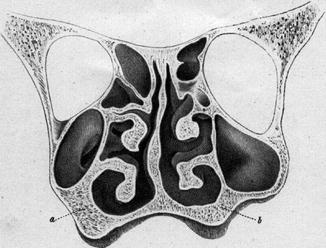
Fig. 34.6
Physiological septal deviation in an asymmetrical skull; The thicker spongiosa (a) compared to the thinner one (b) narrows the right maxillary sinus (Zuckerkandl 1882)
Until now, the diagnosis of the ‘physiological septal deviation’ has mainly been made by nasal endoscopy and the subjective history of the patient to be free of nasal breathing problems. In a prospective cohort study, Gogniashvilli et al. (2011) investigated 158 patients between 18 and 40 years using rhinoresistometry, acoustic rhinometry, endoscopy and visual analogue scales for subjective complaints. They defined the normal one-sided nasal resistance as less or equal to 0.35 sPalcm3 at a flow velocity of 250 cm3/s. Applying this benchmark, the unselected group of non-rhinological patients was differentiated into 144 subjects with physiological nasal resistance and 44 with pathological septal deviation. This means an incidence of 72.2 % physiological septal deviation in an unselected cohort which fits well with the data mentioned above.
34.3.4 34.3.4 Congenital Nasal Deviations
The histological depiction of a slight septal deviation in a newborn without signs of an acute injury is documented in Lindsay Gray’s Fig. 34.3. In neonates, two types of nasal deviation are observed: a septal dislocation that can easily be replaced in the midline and a nasal deviation that cannot be replaced by manipulation. The first type is considered as nasal trauma during delivery. The second type had been published by several authors since the end of the nineteenth century (Pentz et al. 1994) and more detailed by Cottle (1951) who generally concluded: ‘For these, expectant waiting is recommended unless there is complete inability to breathe and eat. One sees remarkable improvement in the appearance and development of these noses without surgical intervention. During the first decade, however, some will require surgical aid’. To find out the incidence of these two types of nasal deviation, we investigated 3,425 children, born in 2 years between 1980 and 1981, in the Obstetric Department of the University of Ulm, Germany. A total of 110 (3.23 %) of these neonates, belonging to the Caucasian population, showed deviated nasal structures. 81 (2.37 %) of these dislocated septa could easily be replaced by a closed reposition. In 29 (0.86 % of 3,425) newborns, reposition of the deviated nasal structure was impossible, thus leaving the baby with a deviated septum and bony pyramid like the neonate in Fig. 34.7 (left) who presents nasal deviation to the left, oblique columella and asymmetrical nostrils.
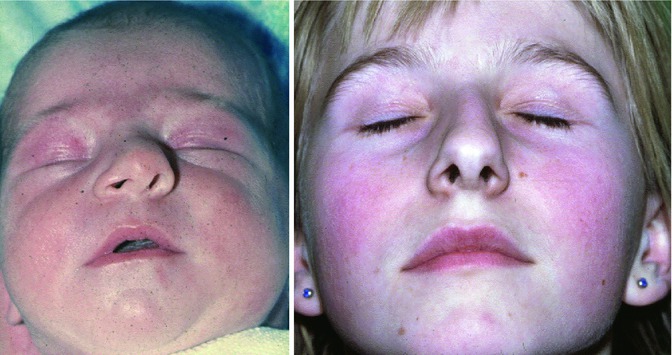
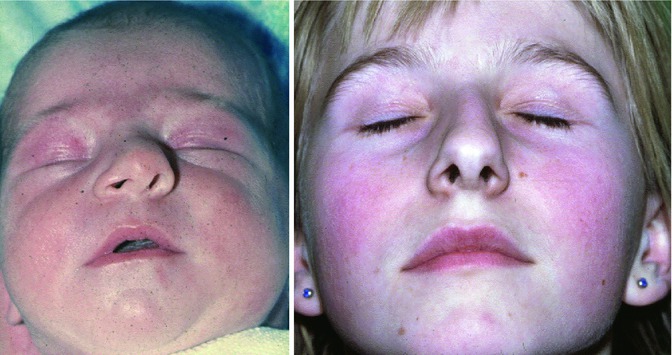
Fig. 34.7
Female newborn with a non-replaceable nasal deviation (left). 12 years later, the girl presents with an inverted C-shaped nasal deviation and nasal obstruction (right) (Pirsig 1992; unpublished)
Over a period of 11–12 years, 14 children out of 29 newborns with the non-replaceable nasal deviation were prospectively followed by the author and a second otorhinolaryngologist (Pentz et al. 1994). No child had a history of nasal trauma in the meantime. The results show that the newborns’ noses, deviated intrauterinely due to some unknown reasons, did not spontaneously restore in each case. Nine children had a completely straight bony pyramid, symmetric nostrils and reported of subjectively normal breathing. Four children had proven nasal allergy and presented bilateral hypertrophy of the inferior turbinates. Eight of the nine septa showed slight deviations, spurs or crests. Eight of these nine children had malocclusion, two of them having been treated orthodontically. We considered these eight children as having a ‘physiological septal deviation’ in an asymmetrical skull, as described by Zuckerkandl in 1882 (Zuckerkandl 1882). However, five girls of the 14 children showed a deviation of the nasal pyramid to the same side as found at birth, in one girl markedly (Fig. 34.7 – right), in a second girl only minor and in three cases slightly. In four of these five girls, a longitudinal deviation, corresponding to the deviation of the bony pyramid, was evaluated by nasal endoscopy and measured by acoustic rhinometry. One of the five girls complained of moderate obstructive breathing, which was caused by allergic rhinitis. All five girls showed a malocclusion, two of them being under orthodontic therapy.
34.3.5 34.3.5 Acromegaly
Acromegaly is an endocrine disease due to growth hormone excess originating from somatotrophic adenoma of the pituitary gland. Patients complain about the coarsening of the facial contours caused by a bony proliferation of the skull and mandible and by an excessive nasal growth. Our groups in Ulm and Zurich (Vetter et al. 1984) investigated the growth mechanism of the septal cartilage in six acromegalic patients. Small strips of septal cartilage were obtained during septoplasty from healthy adults or during transnasal hypophysectomy from acromegalic patients. Growth activity in five different areas of the septal cartilage was measured by in vitro incorporation of 35 S-labelled sulphate and 3 H-labelled thymidine. The growth activities in the posterior area, which is situated anterior to the septoethmoidal junction, were significantly enhanced compared to a control group of hormonally normal adults. Growth activities in the anterior caudal end and in the suprapremaxillary area were not different in both groups. This indicates that growth hormone excess in acromegaly enhances human septal growth by stimulating the growth activities in the posterior area.
In another study (Vetter et al. 1985), five different enzymatic pathways were analysed in these septal areas. Cathepsin D, an acid proteinase, was not influenced by the augmented growth hormone level in acromegaly, whereas cathepsin B, a neutral proteinase, showed its highest activity in the caudal prolongation and the posterior area and was significantly increased in all areas in acromegaly. Beta-hexosaminidase activity was highest in the central and posterior area and caudal prolongation of the septum. In acromegaly, a significant increase of its activity was found in the suprapremaxillary and posterior area. Acid phosphatase activity was highest in the caudal prolongation of the septum, but its activity was significantly increased in all tested areas in acromegaly. Alkaline phosphatase activity could only be found in the posterior area and the caudal prolongation in healthy adults. However, in acromegaly this enzyme could be detected in the central area and the posterior end of the suprapremaxillary area, suggesting an altered process of mineralisation. Thus, a distinct local pattern of enzymes related to intercellular substance metabolism and mineralisation can be demonstrated in the septal cartilage of healthy adults and acromegalic patients.
34.3.6 34.3.6 Damaged or Lacking Triangular Cartilage
What happens when parts of the septodorsal cartilage are damaged or removed? Verwoerd and Verwoerd-Verhoef (2010) found that the behaviour of hyaline cartilage of the human nose appeared to be comparable to that of other mammals, especially of rabbits. Their results can be supported by the following own observation. Because of histologically suspected sarcoma, the left nasal bone and triangular cartilage in a 6-year-old boy had to be resected in 1973 (Pirsig 1992). Fortunately, a curable circumscribed osteomyelitis was diagnosed. Following this boy 7 years later, we found a shortened left nostril with the bony pyramid deviating to the left and the nasal tip deviating to the nonoperated side. The left piriform aperture was positioned higher and the left nasal process of the maxilla was reduced. The left inferior turbinate was smaller than the right-sided one, the caudal end of the septal cartilage slightly deviated to the right (Fig. 34.8). Thus, the resection of one triangular cartilage and the nasal bone in connection with an infection affected the growth of nasal and midfacial structures. These findings are similar to Poublon’s results from the Rotterdam group after unilateral resection of the triangular cartilage in growing rabbits (Poublon 1987).
