Fig. 20.1
Flow paths in a tube with laminar flow (ΔP = friction-related pressure loss,
= flow velocity)

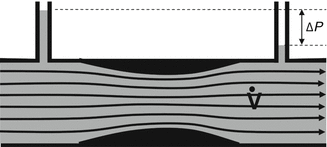
Fig. 20.2
Flow paths in a tube with a constriction with laminar flow (ΔP = friction-related pressure loss,
= flow velocity)

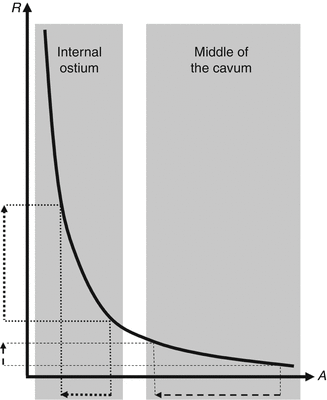
Fig. 20.3
Changes in nasal airway resistance (R) depending upon the cross-sectional area (A) in the area of the internal ostium and the middle of the nasal cavum
This helps to explain why even minor constrictions located in the ostium internum (nasal valve) have such a pronounced effect on nasal airway resistance, whereas in the center of the nasal cavity, septal deviations have hardly any impact on airway resistance (Masing 1967; Hess et al. 1992; Dinis and Haider 2002). This means:
That if there is concurrent narrowing in the internal nasal valve area and in the middle nasal cavum, it is almost always the valvular constriction that is critical for nasal obstruction.
That in the presence of a deviation in the middle cavum, one must always search in addition for valvular constriction. These valvular constrictions often turn out to be the actual cause of the elevated resistance (Dishoeck 1942; Cole et al. 1988; Bachmann 1989; Mlynski et al. 2001), but they can easily be overlooked; as a result, “physiological septal deviations” (Zuckerkandl 1882; Gogniashvili et al. 2011) are subjected to surgical correction in the cavum (see Sect. 20.3.2).
Turbulence also leads to increased airway resistance, since the flowing particles hit up against each other and against the wall through lateral movements, and this leads in the same way to energy loss (Fig. 20.4).
During turbulent flow, the increased resistance does not depend solely on the flow velocity but also on the friction coefficient λ. The friction coefficient, which is a dimensionless number, describes the aerodynamic characteristics of the internal surface of a structure through which air passes. This is comparable to the c w–value, characterizing the external surface of a structure, where the air circulates around (e.g., for automobiles or airplanes).
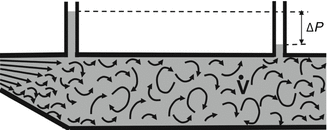
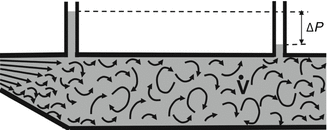
Fig. 20.4
Flow paths in a flow channel with turbulent flow (ΔP = friction-related pressure loss,
= flow velocity)

20.3 Correlation of the Structure and Respiratory Function of the Nose
From a functional and fluid dynamic perspective, the nose is a very complicated structure. Researchers have been attempting to investigate the nasal flow channel for more than 100 years (Paulsen 1882; Kayser 1889; Rethi 1900; Burchardt 1905; Mink 1920; Takahashi 1922; Hellmann 1926; Dishoek 1936; Scheideler 1938; Tonndorf 1939; Masing 1967; Fischer 1969; Bachmann 1982a, b; Naito et al. 1989; Hess et al. 1992; Mlynski and Loew 1992a, b; Simmen et al. 1999; Mlynski 2000a, b; Mlynski et al. 2001; Gruetzenmacher et al. 2005, 2006).
For simplifying our understanding of its function, it is helpful to divide the nose into a few different sections. These sections can then be compared with mechanical form elements, whose impact on flow is known from fluid physics (Table 20.1).
Table 20.1
Fluid dynamic effects of structural form elements that may be compared to anatomical structures in the nose
Form element | Effect on flow dynamics |
---|---|
Bend | Altering the direction of flow |
Nozzle | Decreasing turbulence, accelerating flow |
Diffuser | Creating turbulence, decelerating flow |
Concave opening | Divergence of flow paths |
Slit space | Favors exchange of heat, humidity, and cleansing between the mucosa and the particle stream |
The schema presented in Fig. 20.5 goes back to Bachmann (1982a, b) and was then enhanced after we conducted extensive flow-experimental studies (Mlynski 2000a, b; Mlynski et al. 2001). The functional area is the principal location of the nasal turbinates. Here, the air is warmed, moistened, and cleansed. In the inspiratory direction, the inflow area is made up of the nasal vestibulum, the nasal isthmus, and the anterior cavum, with the head of the inferior turbinate and the erectile tissue of the septum. The nasopharyngeal meatus, the choanae, and the epipharynx constitute the outflow area.
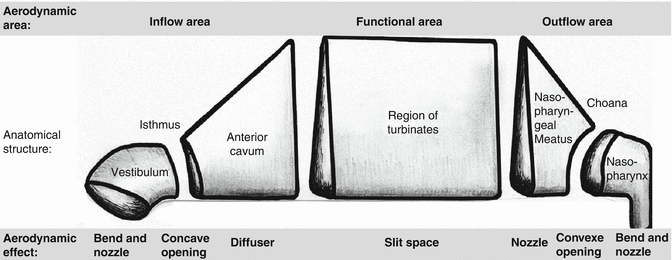
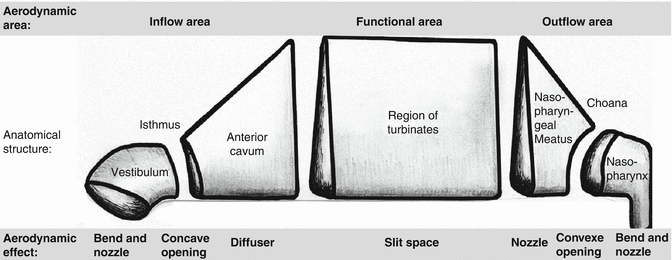
Fig. 20.5
Schematic representation of dividing the nose into regions and comparative form elements in the inspiratory flow direction
20.3.1 Inflow Area
The inflow area consists of the nasal vestibulum, the nasal isthmus (“internal valve”), and the anterior cavum. The function of the inflow area is to configure the airstream so as to facilitate sufficient contact with the mucosa in the functional area. Figure 20.6 illustrates the course and character of flow in the inspiratory airflow direction in the inflow area of a nasal model (Mlynski et al. 2001).
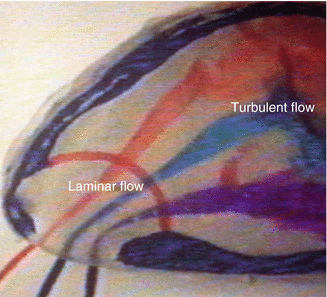
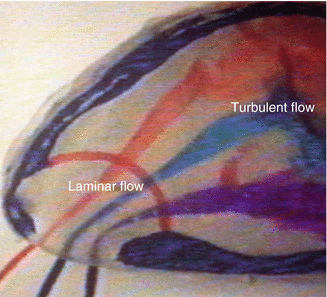
Fig. 20.6
Flow-experimental presentation of inspiratory flow in the inflow area The flow is directed towards the turbinate region (bend effect) and turbulent flow portions become laminar (nozzle effect). Laminar flow can be recognized from the sharp demarcation between the flowing medium and the colored particles provided for better visualization. With increasing turbulence, a diffuse coloration develops as a result of lateral movements of the flowing particles
20.3.1.1 Vestibulum Nasi
The vestibulum nasi is shaped like a short bent tube and thereby is equivalent to a bend. The curvature is created by the relative position of the external and internal nasal openings: the ostium externum (inflow opening of the bend) is more horizontal, and the ostium internum (outflow opening of the bend) is more vertical. The fluid dynamic effect of this bend redirects the inspiratory airstream from anterior and inferior towards the area of the turbinates (Fig. 20.6).
In this process, the relative position of the vestibulum to the cavum is of significance. In a drooping nose with a small nasolabial angle, the vestibulum is rotated with the alar cartilage pointed downwards. During inspiration, this leads to a very high flow course in the cavum (Fig. 20.7b). As a result, the mucosa in the inferior turbinate cannot contribute to the respiratory function. If the nasolabial angle is too large, the vestibulum and the alar cartilage are rotated upwards. As a consequence, the airstream runs very low through the cavum, and thus, the mucosa of the superior turbinate is no longer capable of contributing to respiratory function (Fig. 20.7c).
When the vestibulum is malpositioned, it is not possible to make use of the entire nasal flow channel, and this functional constriction, similar to an anatomical constriction, results in increased airway resistance.
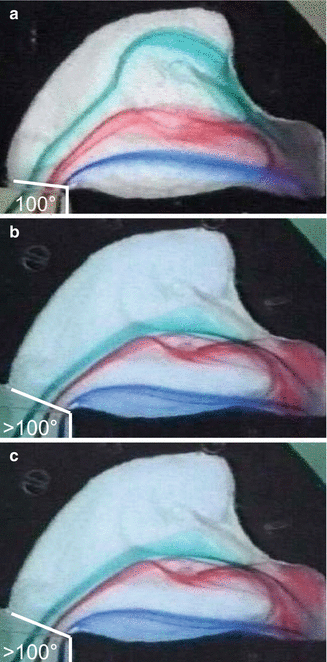
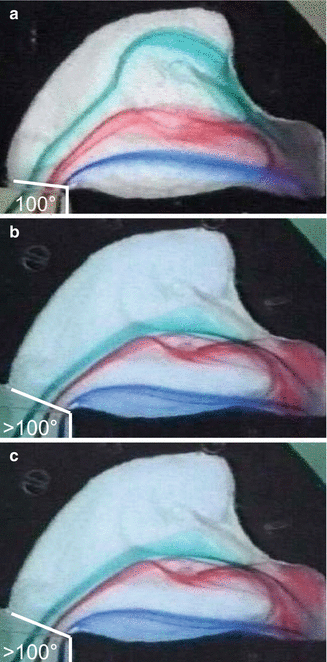
Fig. 20.7
Course of inspiratory flow in nasal models with various positions of the vestibulum to the cavum (a) Normal position: air is distributed over the entire region of turbinates (b) Vestibulum rotated downwards in the presence of a drooping nose with a pathologically diminished nasolabial angle: air flow runs only through the upper region of the nasal cavum (c) Vestibulum rotated upwards in the presence of a nose with a tip rotated excessively upwards: airflow runs only through the lower part of the nasal cavum
In cases presenting with an abnormal nasolabial angle, the rhinosurgical challenge is to reconstruct the normal position of the alar cartilage. This means that the cephalic portions of the alar cartilage must be positioned on the caudal edge of the lateral cartilage. Therefore, correction of an inadequate or excessive nasolabial angle is necessary not only for aesthetic reasons but also from a functional point of view. Excessive elevation of the tip of the nose motivated by aesthetic considerations should be avoided.
Since the ostium externum is larger than the internal valve area, the vestibulum also has a nozzle effect in the inspiratory direction. In a nozzle, progressive constriction of cross-sectional area serves to reduce turbulent flow areas. As a consequence, the flow character of inspired air in the next and physiologically narrowest area of the nose, the nasal isthmus, is predominantly laminar (Fig. 20.6). This is of importance, since turbulent flow in this narrowing would result in very high levels of resistance to flow.
The nozzle effect of the nasal vestibulum must be preserved in rhinosurgical procedures. This means that one must not overexpand the isthmus in an effort to reduce resistance. The ostium internum must remain proportionately smaller than the ostium externum. Excessive expansion leads to a ballooning phenomenon that creates highly turbulent flow in the cavum as a result of the elimination of the nozzle effect in the vestibulum.
The function of the vestibulum as a nozzle also results in an acceleration of the local flow velocity, which may cause portions of the mobile lateral vestibular wall to be sucked in, as a consequence of the Bernoulli effect. Typically, this suction phenomenon (also referred to as “nasal valve collapse”) only begins to occur with forced breathing (flow >500 ml/s). When suction occurs in this situation, the effect is known as “physiological nasal valve collapse,” which can be understood as protecting the mucosa against excessive nasal air perfusion. By contrast, “pathological nasal valve collapse” begins to arise at low flow velocities and in extreme cases even at rest. Thus, it leads to symptoms of nasal obstruction.
Most cases of pathological nasal valve collapse result from constrictions of the vestibulum and the ostium internum. This is because negative pressure in a flow channel depends upon the velocity of flow, which depends in turn upon the width of the channel: the narrower the channel, the greater the local flow velocity. The rhinosurgical implication in these cases is the physiological need to expand the area of constriction.
20.3.1.2 Isthmus Nasi
The isthmus nasi is the narrowest region along the nasal flow channel and thus the site with the highest resistance to airflow (Dishoeck 1942; Masing 1967; Fischer 1969). The effects of a constriction on flow and the relationship between cross-sectional area and resistance were presented in Sect. 20.2.
In the inspiratory flow direction, concave curvature of the internal nasal ostium, which serves as the aperture for air passing from the vestibulum to the cavum, can also affect the dynamics of flow. An aperture with a concave curvature has the same impact on flow paths as a concave lens does on light rays: it creates divergence of the flow paths, thereby making a contribution to distributing flow over the entire surface area of the turbinate region (Mlynski 2000b; Mlynski et al. 2001) (Figs. 20.6 and 20.8).
In view of these functional considerations, resections of the caudal edge of the lateral cartilage must be performed in such a way as to preserve the concave shape of the nasal ostium internum.
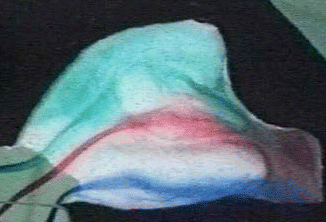
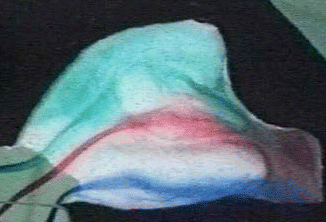
Fig. 20.8
Flow-experimental presentation of inspiratory airflow in a nose model without a vestibulum. The concave curved surface of the internal nasal orifice causes divergence of the flow paths in the cavum
20.3.1.3 Anterior Nasal Cavum
In the inspiratory direction, due to the expansion in cross-sectional area distal to the ostium internum, the anterior nasal cavum shows the fluid dynamic characteristics of a diffuser. This is the location of the principal structures for regulating turbulence, the head of the inferior turbinate, and the erectile tissues of the septum, the intumescentia septi. Depending on the expansion in cross-sectional area and the flow velocity, a diffuser generates portions of turbulent flow (Mlynski et al. 2001). The operating principle of the nasal diffuser in relation to respiratory function is presented in Sect. 20.4.
The second positive effect of the expansion in cross-sectional area in the anterior cavum is deceleration of flow. The slow velocity of airflow in the turbinate region results in a prolongation of contact time with the mucosa, thus favoring the exchange of heat and moisture.
20.3.2 Functional Area
The functional area in the nose is the turbinate area. In this region, the nasal cavity is constricted into a narrow space by the turbinates. As a result, the nasal cavity is actually not a cavity at all, but a slit space. This slit space is necessary for the proper respiratory function of the nose (see Sect. 20.3.2). Analysis of CT images through the nose (Fig. 20.9 shows a few examples) reveals that the width of the slit remains quite constant across the entire cross section of the cavum, even though it is extremely rare for the septum to be straight. The lateral walls of the cavum are always asymmetrical. The septum divides the nose into two unequally wide but fully separate structural cavities. The turbinates adjust themselves in shape and size to the space determined by the lateral wall of the cavum and the septum, resulting in the creation of a continuous, uniform slit space.
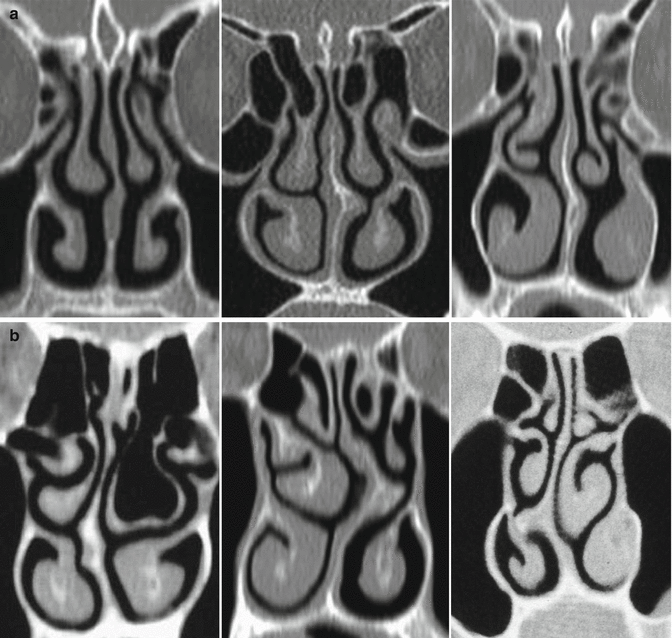
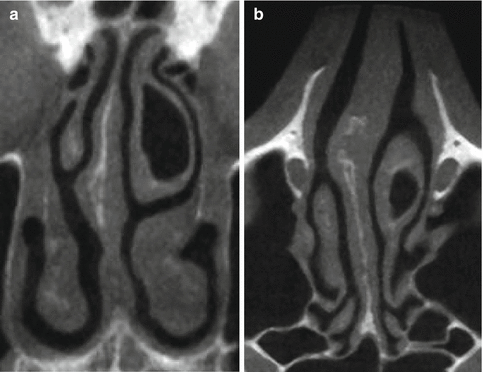
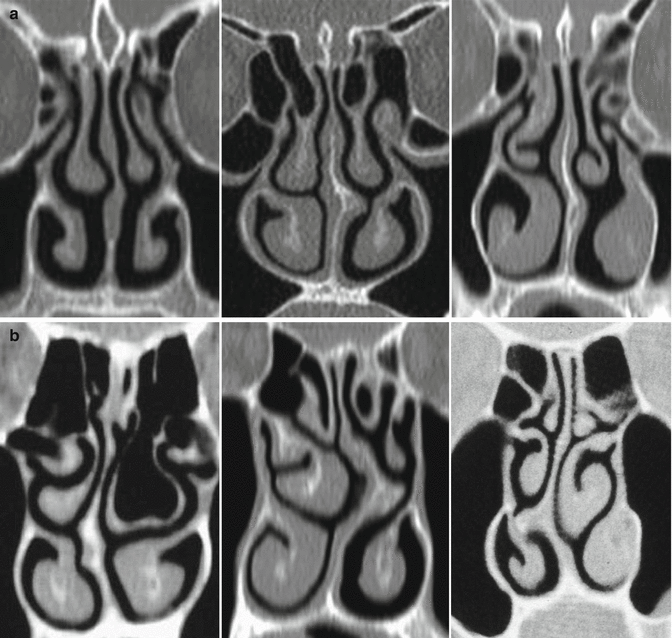
Fig. 20.9
Coronal CT images through the functional area of different noses. The turbinates adapt to the space provided by the lateral wall of the cavum and the septum in the case of slight asymmetry of the cavum and physiological septal deviation (a), and even in the case of marked asymmetry with pathological septal deformities (b), so that whenever possible, a continuous slit space is maintained
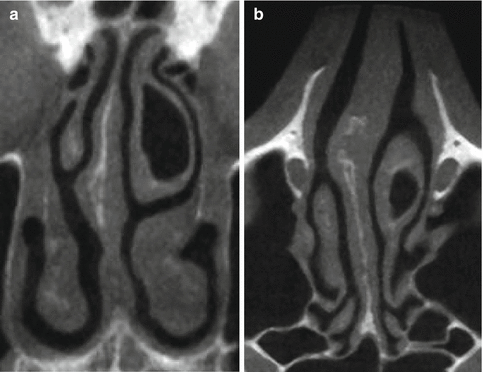
Fig. 20.10
(a) coronal and (b) axial CT images through the nose As a result of the interplay between the shape and size of the nasal turbinates and the width of the septum with its erectile tissues, the asymmetrical nasal cavity is narrowed down to form a possibly uniform slit space
Even the septum makes a contribution to the creation of a constant slit space through its shape and variable thickness (Fig. 20.10).
By the end of the nineteenth century, Zuckerkandl (1882) discovered using his large collection of skulls that in the ubiquitously asymmetrical human skull, the septum is not usually straight, but instead displays “physiological deviations.” The common observation on the part of ENT specialists that not every septal deviation causes nasal obstruction (Altissimi et al. 1992; Hanif et al. 2003) attests to the validity of physiological deviation. In fact, the literature suggests up to 90 % incidence of septal deviation in a normal population (Sooknundun et al. 1986; Podoshin et al. 1991; Uygur et al. 2002; Gola et al. 2002; Gogniashvili et al. 2011).
We define physiological deviation of the septum as a bend in the nasal septum in the absence of significant narrowing of the slit space, that means without elevation of nasal airway resistance.
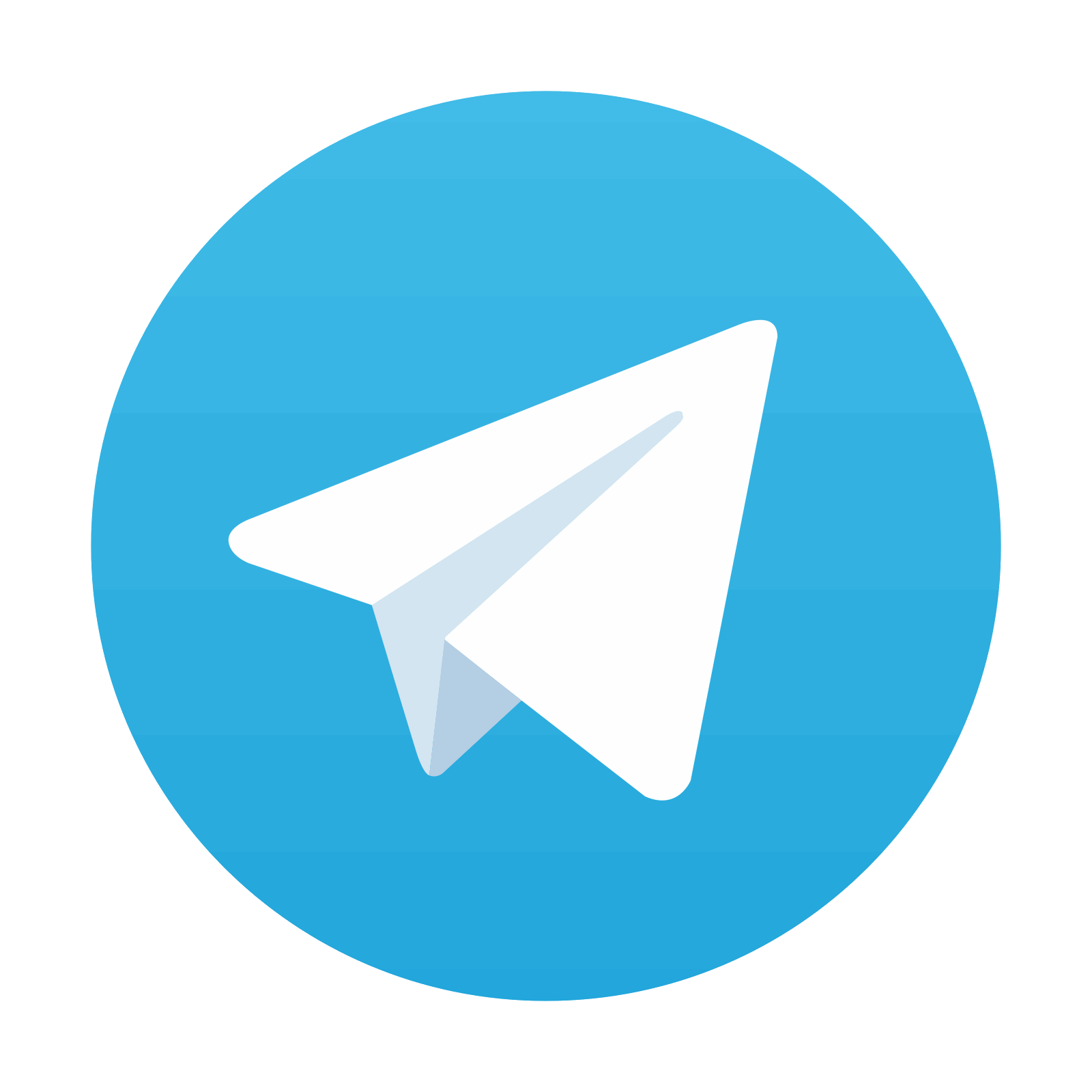
Stay updated, free articles. Join our Telegram channel

Full access? Get Clinical Tree
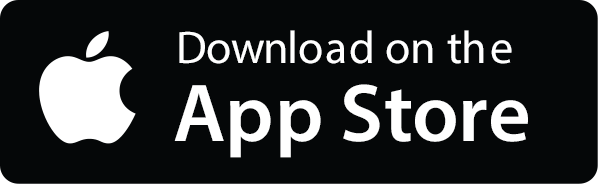
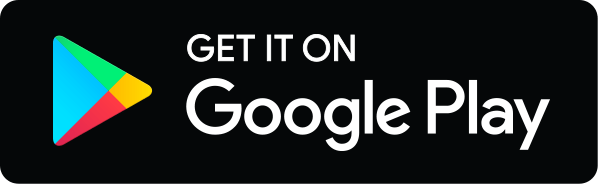