Fig. 36.1
Report from CNN (Cable News Network) Food and Health May 1996 claiming that one in five “nose jobs” (rhinoplasty operations) needs to be “fixed” again because of a postoperative breathing disturbance.
Realizing that the range of complications after rhinoplasty presented in literature varies somewhere from 6 to 10 % (approximate numbers) in experienced hands, after viewing web sites and contacting plastic, facial plastic, and cosmetic societies by phone, I estimate that the number of rhinoplasty operations performed in the USA is about 500,000 operations per year
36.2 Anatomy of the Nasal Valve Area
Since my primary physiologic concern during cosmetic rhinoplasty centers on the breathing function and the nasal valve is the most critical area for breathing, a review of both the anatomy and physiology of the nasal valve area is imperative. Regarding the anatomy, an arbitrary subdivision of the nasal valve for practical clinical thinking has been forwarded in the literature, suggesting that we think of the nasal valve area as having two component parts. First, the internal nasal valve (most of our discussion will center around the internal nasal valve as it is composed of a number of diverse anatomic parts) and an external nasal valve, which is primarily composed of the lower lateral cartilages and surrounding soft tissues, which are covered both dorsally and ventrally by skin. Both valves (internal and external) are still subjected to the laws of fluid (air is the fluid) dynamics (Barelli et al. 1987; Bridger 1970; Bridger and Proctor 1970; Cole 2000, 2003; de Wit et al. 1965; Eccles 2000; Haight and Cole 1983; Hilberg et al. 1989; Hinderer 1971; Huizing and de Groot 2003; Kasperbauer and Kern 1987; O’Neill and Tolley 1988; Van Dishoeck 1942, 1965; Wexler and Davidson 2004).
36.2.1 Internal Nasal Valve
First, let’s look at the anatomy of the external nose. The component parts of the external nose include a nasal skeletal structure (bone and cartilage) conveniently divided into three distinct and separate anatomic parts including:
1.
Upper bony portion (paired nasal bones).
2.
Middle third composed of the single upper lateral extension of the septal cartilage (the upper lateral cartilage is one cartilage and should not be thought of as two separate cartilages); this upper lateral cartilage is also termed the roof or triangular cartilage (Fig. 36.2). At its distal end (caudal end), the upper lateral cartilage is usually separated by a narrow cleft from the nasal septum and projects beneath the paired lower lateral cartilages.
3.
Lower third of the external nose is composed of paired lower lateral cartilages (also called lobular, rim, alar, or great alar cartilages).
For emphasis, remember that this single upper lateral cartilage is attached proximally beneath the paired nasal bones and is attached distally beneath the lower lateral cartilages (Barelli et al. 1987; Hinderer 1971; Huizing and de Groot 2003). At its distal (caudal) end, the upper lateral cartilage frequently curls back upon itself forming a portion that is called the “scroll” also termed “returning” which is in contact with proximal (cephalic) end of the lower lateral cartilages (Barelli et al. 1987; Gray 1970; Hinderer 1971; Huizing and de Groot 2003). The upper lateral cartilage also extends laterally out to the bony margin of the piriform aperture formed, in part, by the frontal (ascending) process of the maxilla. This external nasal skeletal structure (bone and cartilage) is actually covered externally by the soft tissues of skin, muscles, perichondrium, periosteum, and neurovascular bundles. Internally (intranasal) these structures have mucocutaneous (skin and mucosa) coverings.
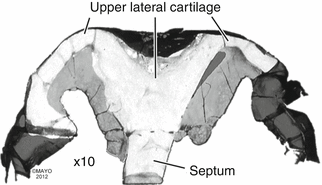
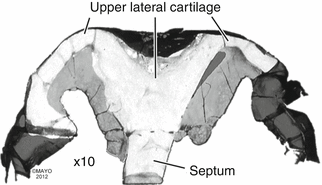
Fig. 36.2
Photograph showing that the upper lateral cartilage (roof cartilage) is an upper lateral extension of the septal cartilage in human. Photograph was taken after a rhinectomy that the author performed for a patient with an invasive nasal cancer (By permission of Mayo Foundation for Medical Education and Research. All Rights Reserved)
The entire internal nasal valve is more than the relationship between the distal end of the upper lateral cartilage and its angle formed with the nasal septum. Because of the complexity of the anatomy and the various papers on the subject, I think, for practical and functional reasons, the nasal valve should be thought of as an area. I think of the nasal valve area as a three-dimensional inverted cone-shaped structure that extends from the region of the distal (caudal) end of the upper lateral cartilage in its relationship to the nasal septum medially, including the premaxillary wing region, floor of the nose, and laterally to the bony piriform aperture including the soft fibrofatty areolar tissue of this region of the internal nasal valve and is bounded posteriorly by the head of the inferior turbinate (Figs. 36.3 and 36.4).
Various workers have investigated the internal nasal valve and determined the approximate site using various methods, but the overwhelming consensus is that most of the upper airway resistance is provided by the internal nasal valve and about one third of that resistance is due to the cartilaginous vestibule and approximately two thirds of that nasal resistance is due, in large measure, to the congestive capabilities of the anterior end (head) of the inferior turbinate. This turbinal “swell” body has a counterpart on the septum (“septal turbinate, swell body, or septal body”) located on the nasal septum approximately adjacent to the anterior end (head) of the inferior turbinate (Miman et al. 2006; Wexler and Davidson 2004). The finding of the septal turbinate (swell body) was first described in the 1600s, and these findings have been reported and supported by investigations in humans (Barelli et al. 1987; Miman et al. 2006; Wexler and Davidson 2004). This entire internal nasal valve area ranges somewhere between 55 and 64 square millimeters.
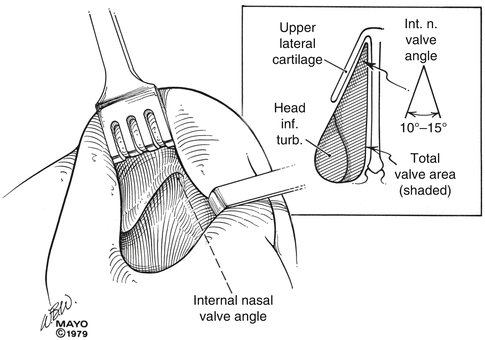
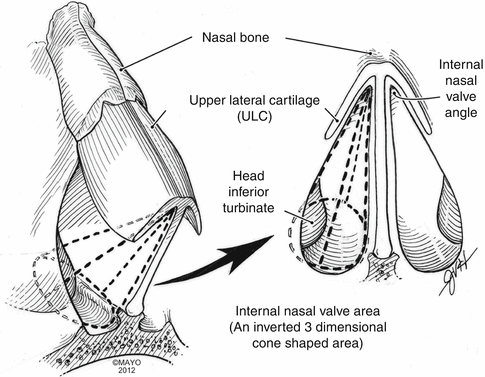
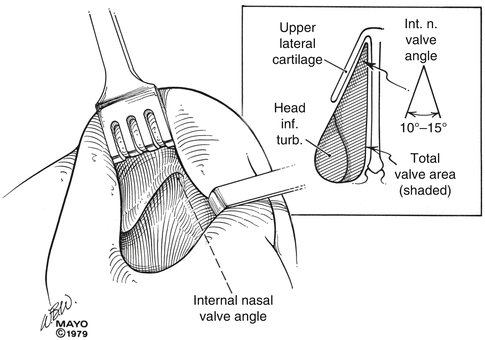
Fig. 36.3
This illustration demonstrates the clinical internal nasal valve angle and in the upper right corner of the illustration a representative view of the total internal nasal valve area which includes the upper lateral cartilage, the septal cartilage (the septal turbinate “swell body” is not shown here), the floor of the nose, the piriform aperture including the frontal (ascending) process of the maxilla, and the head of the inferior turbinate are all shown. The internal nasal valve angle is represented as ranging from 10 to 15 ° although some authors have different findings (Miman et al. 2006) (By permission of Mayo Foundation for Medical Education and Research. All Rights Reserved)
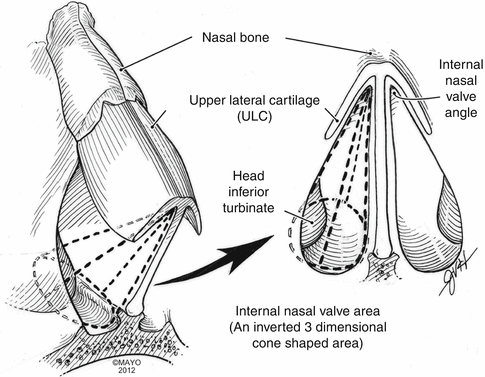
Fig. 36.4
Illustration demonstrates that the internal nasal valve area can be thought of as a three-dimensional structure (an inverted cone) with triangular portion (bottom) of cone fitting into the apex of the internal nasal valve angle (By permission of Mayo Foundation for Medical Education and Research. All Rights Reserved)
The narrowest portion of the nasal airway is the apex of the internal nasal valve area at the nasal valve angle which is the specific triangular slit-like portion between the caudal (distal) end of the upper lateral cartilage and the nasal septum (Figs. 36.3 and 36.4). This nasal valve angle was first described over 100 years ago by Mink and is continuously re-reported to be approximately 10–15 ° in the leptorrhine (Caucasian) nose, as pointed out by Miman and coworkers (Miman et al. 2006). In their prospective comprehensive study of 248 nasal cavities using direct endoscopic examination along with acoustic rhinometry and rhinomanometry, they discovered different types of nasal valve angles, ranging “between 22.5 and 52 °” (Miman et al. 2006). Miman et al. studied normal asymptomatic control subjects and proposed a classification related to the upper lateral cartilage’s caudal border stated as convex, concave, or twisted and the valve angle as blunt, sharp, or occupied by the septal body. The septal body has been noted and reported before with a prevalence ranging from 52 to 66 % of individuals (Arbour and Kern 1975; Wexler and Davidson 2004). Miman et al. also discussed another study whose authors used computed tomography to study the nasal valve angle and that was reported to be 11.4 ° +/− 2.6 °. After closer study of the other data obtained from computed tomography (while Miman et al. used direct endoscopic examination along with acoustic rhinometry and rhinomanometry), Miman et al. realized that positioning and placement of the lines for measurement are subjective and even a slight alteration of line placement could significantly alter the results by at least 15–20 °. In light of their findings, Miman et al. concluded that the literature’s emphasis on a normal nasal valve angle of 10–15 ° needs challenge and must be open for further study and discussion.
It is the entire internal nasal valve area that is the primary airflow inflow regulator; accounting for the majority of the inspiratory resistance to inspiratory airflow and the head of the inferior turbinate is the posterior portion of the internal nasal valve area. The head of the inferior turbinate has a significant and at times a dominant role (think acute viral or acute allergic rhinitis) as an inflow regulator in the Caucasian (leptorrhine), Black (platyrrhine) nose, and in the Asian (mesorrhine) nose. Remember that the functional airway unit is the entire internal nasal valve area and any component anatomic part including the upper lateral cartilage; the anterior nasal septum, including the premaxillary wing region, floor of the nose, the piriform aperture, lateral fibrofatty areolar tissue, frontal (ascending) process of the maxilla and the head of the inferior turbinate, or the skin or mucosa (mucocutaneous coverings); or any combination of those structures when anatomically or pathologically altered from “normal” may contribute to dysfunction of the entire internal nasal valve area. In other words, any anatomic part of the internal nasal valve area may be surgically altered during primary rhinoplasty and therefore vulnerable to surgical alteration that could have negative consequences for breathing function postoperatively (Adamson et al. 1990; Araco et al. 2007; Becker et al. 2008; Beekhuis 1976; Berry 1981; Bruno et al. 2005; Constantian and Clardy 1996; Courtiss and Goldwyn 1983; Fischer and Gubisch 2006; Goode 1985; Hinderer 1970; Jenssen et al. 1988; Kern 1977a; Kern 1978, 1988b, 1991; Kern and Wang 1993; Kocer 2001; McCaffrey et al. 1983; McKee et al. 1994; Teichgraeber and Wainwright 1994).
36.2.2 External Nasal Valve
The external nasal valve is that portion of the nasal airway (ala of the nose) that is sprung open by the tensile strength of the paired lower lateral cartilages and the covering soft tissues and skin of the ala. The external nasal valve becomes a problem for the patient if the lower lateral cartilage is resorbed secondary to nonsurgical trauma, surgical trauma with over-resection of the lower lateral cartilages or changes in the tensile strength of the cartilage due to the atrophy of aging or loss of muscle tone secondary to facial palsy or other neurologic disorders (Constantian 1994; Huizing and de Groot 2003; Kern 1980b; Rizvi and Gauthier 2011; Schlosser and Park 1999).
36.3 Physiology of the Nasal Valve Area
Today, 40 years after Williams merely speculated that the nasal valve functions as a type of inflow device controlling the rate and depth of respiration, the complete function of the nasal valve is still unknown (Williams 1972). Hinderer suggested that the nasal valve controls inspiratory air currents changing them from a column to a sheet of air, thereby giving shape, velocity, direction, and resistance to the inspired air (Hinderer 1970, 1971). It has been previously suggested that the head of the inferior turbinate (Gray 1970) and the septal turbinate (Barelli et al. 1987; Cottle et al. 1958; Wexler and Davidson 2004) work in concert and both are important components of the internal nasal valve area because they provide the necessary resistance to breathing during inspiration. The nasal valve has been considered (Bridger 1970; Bridger and Proctor 1970) to function as a Starling resistor influenced by Bernoulli forces so that when the airflow increases (accelerates), the interior nasal pressure decreases allowing the lateral nasal walls to collapse inward (towards the nasal septum). Both the nose and a Starling resistor consist of a semirigid tube with a short collapsible segment (the internal and external nasal valve). The portion of the internal nasal valve at the head of the inferior turbinate can be considered the upstream segment, whereas that portion posterior to the head of the inferior turbinate and the septal body is the downstream segment (Fig. 36.5). In other words, the semirigid tube conducts pressure changes to the collapsible segment, which is influenced by several factors including the conducted pressure, the extramural pressure, Bernoulli forces, and the elasticity of the collapsible cartilaginous segment. In the nose, negative inspiratory pressure is transmitted from the nasopharynx to the internal nasal valve area, which then narrows. The degree of narrowing depends on three variables:
1.
The pressure difference between the internal nasal pressure during breathing and the atmospheric pressure (transmural pressure)
2.
The flexibility of the valve area’s collapsible cartilaginous segment
3.
The size of the valve area
In the normal nose, the nose does not collapse during quiet breathing because the tensile strength of the cartilage (upper lateral cartilage, lower lateral cartilage, and septal cartilage) support of the nose offsets the closing forces of the transmural pressure and the Bernoulli effect. The resistance to airflow is dependent on the skeletal (cartilage and bone) and mucocutaneous nasal structures that are anterior (upstream) and posterior (downstream) to the head of the inferior turbinate. At maximal inspiratory flow rates, a greater inspiratory effort (increased negative pressure) fails to increase airflow because the external nasal valve (ala) is collapsed (Fig. 36.6). The external nasal valve does not remain collapsed as the process is reversed during expiration.
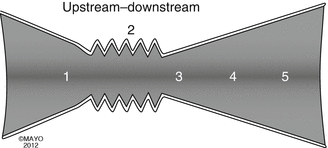
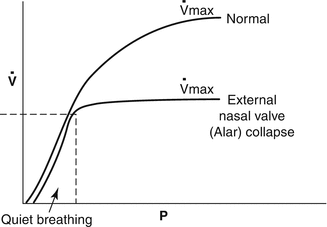
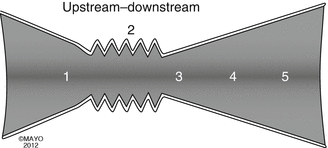
Fig. 36.5
Illustration is a graphic representation forwarding the idea that the nasal valve (internal and external) can potentially function as collapsible segment dividing the nose into upstream – cartilaginous portion (Cottle areas 1 and 2) – and downstream “bony portion” (Cottle areas 3,4, and 5). According to Cottle, the nasal septal areas are as follows: area 1 refers to the nasal septum at the caudal end of the nasal septum. Area 2 refers to the nasal septum at the valve area (now comprising the internal valve (including the anterior head of the inferior turbinate) and external valve (including the lower lateral cartilages)). Area 3 refers to the nasal septum at the attic (the region of the septum beneath the nasal bones). Area 4 refers to the nasal septum and its relationship to the turbinates (except for the most anterior head of the inferior turbinate, which is certainly part of the internal nasal valve and at the same time is the most posterior part of the internal nasal valve). Area 5 refers to the nasal septum and its relationship to the choana (Barelli et al. 1987; Cottle et al. 1958; Hinderer 1971; Huizing and de Groot 2003) (By permission of Mayo Foundation for Medical Education and Research. All Rights Reserved.
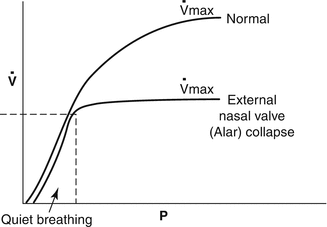
Fig. 36.6
Nasal pressure (x-axis) and nasal flow (y-axis) seen on idealized rhinomanometric curve in two situations.
A normal functioning nose. From a patient with external nasal valve (alar) collapse demonstrating limitation of flow at high inspiratory (negative to atmospheric) pressure.
At maximal inspiratory flow (Vmax), the nose collapses and no further increase in pressure (negative inspiratory pressure) can cause further increase in airflow through the nose. In other words, when the valve (internal and/or external) collapses, there is plateauing of the curve since no matter how hard a subject tries to increase the inspiratory effort (negative pressure during inspiration), there is a cessation of airflow secondary to (internal and/or external) nasal valve collapse
The external nasal valve (ala) is really the airway opening supported by the paired lower lateral (great alar or alar) cartilages. The critical sites for collapse are either at the external nasal valve (lower lateral cartilage) or at the internal nasal valve (nasal valve area composed of the upper lateral cartilage, nasal septum, including the premaxillary wing region, floor of the nose, piriform aperture, and the head of the inferior turbinate). Some observers believe that the septal turbinate (septal “swell” body) is part of the nasal valve area and I tend to agree, but these findings tend to support the notion that total understanding the valve area and its function is still far from fully understood (Haight and Cole 1983; Huizing and de Groot 2003; Miman et al. 2006; Wexler and Davidson 2004). Nonetheless, it is necessary to have some practical understanding of the nasal valve area and its critical sites in order to perform surgery without disturbing nasal breathing function.
These critical sites are seen in Fig. 36.7. A clinical un-instrumented view of many of the structures of the internal and external nasal valve can be seen in Fig. 36.8. The alar muscles are also involved in valvular function and aid in preventing collapse of the valve at high inspiratory flow rates. Rigidity (scar) or flaccidity (over-resection) of cartilage structure including both the upper and the lower lateral cartilages and soft tissues would affect valvular function and breathing. Even minor changes in the diameter of the airway can significantly increase nasal airway resistance in concert with Poiseuille’s laws where the airflow is proportional to the pressure changes times the radius to the fourth power divided by the length. In other words, small changes in the radius to the fourth power are extremely significant.
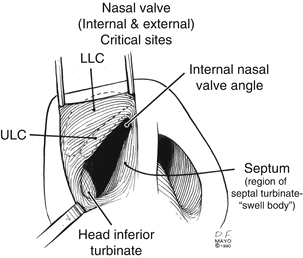
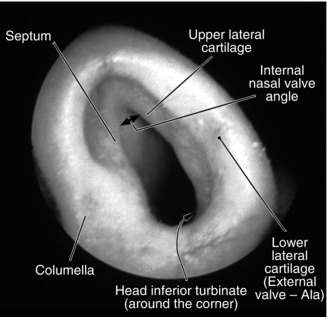
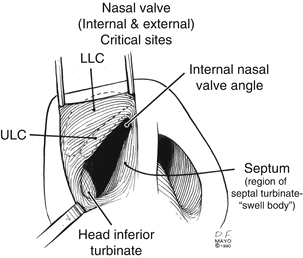
Fig. 36.7
Illustration depicts the most “critical sites” of “PHYSIOLOGIC CONCERN DURING RHINOPLASTY” that the surgeon must understand and avoid sacrificing structural support in these “critical sites”; otherwise breathing dysfunction will most probably occur postoperatively.
These “critical sites” include the following: the lower lateral cartilage (LLC), the major component of the external nasal valve; the internal nasal valve including the upper lateral cartilage (ULC), the septum, and the valve angle between the upper lateral cartilage (ULC) and the septum; and the head of the inferior turbinate.
If any of these “critical sites” are sacrificed for esthetic reasons, then the reconstruction must follow at the time of surgery; otherwise, a postoperative breathing dysfunction will often follow. If the internal nasal valve angle is blunted or scarred, then postoperative breathing dysfunction will almost invariably follow (By permission of Mayo Foundation for Medical Education and Research. All Rights Reserved)
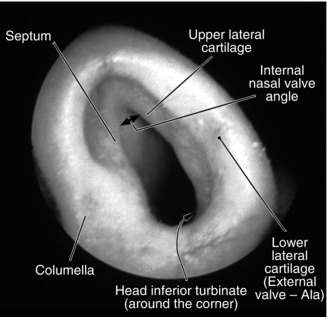
Fig. 36.8
Photograph is an un-instrumented clinical view of the left side of a person with a normal nose. Many structures of the internal and external nasal valve are seen and labeled (By permission of Mayo Foundation for Medical Education and Research. All Rights Reserved)
Using acoustic rhinometry and active anterior rhinomanometry, Zambetti and associates (Zambetti et al. 2001) proved and supported the long-held clinical observation that small deformities in the anterior portion of the nose (upstream) like a scar in the valve angle can more profoundly and adversely effect nasal breathing than larger deformities in the interior of the nose (downstream) when they said, “Modest nasal cross-sectional area reductions posterior to the nasal valve do not cause substantial variations in nasal resistance…” (Zambetti et al. 2001).
The nasal valve area must function in concert with the structures upstream and downstream to provide nasal pulmonary respiratory balance over the wide range of physiologic demands. Since the head of the inferior turbinate is part of the internal nasal valve, it is affected by the vascular changes of the capacitance vessels in the stroma, which is termed the nasal cycle (Arbour and Kern 1975; Hasegawa and Kern 1977; Kern 1981). Actually the nasal cycle is a normal physiologic phenomenon that includes the alternating congestion and decongestion of the turbinates with changes in uninasal resistance while the total nasal resistance remains relatively constant (Fig. 36.9).
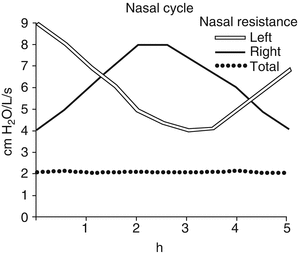
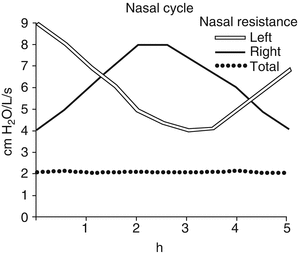
Fig. 36.9
The nasal cycle occurs in approximately 80 % of the adult population. It can be described as the normal physiologic alternating congestion and decongestion of the nasal turbinates producing changes in one-sided nasal (uninasal) resistance. Nasal resistance is calculated from the measurements of the trans-nasal pressure changes during breathing (inspiration and expiration) divided by the airflow during breathing (inspiration and expiration). In other words, resistance = pressure divided by flow. Note that each side (uninasal resistance right and left side) usually alternates (resistance values) with the other side, after the passage of time, while the total nasal resistance remains relatively constant. The patient experiences one side of the nose as obstructed (plugged) and after some period of time the other side becomes obstructed (plugged) while breathing through both sides (both noses) feels unobstructed
36.4 Pathophysiology of the Nasal Valve Area
It is critical that nasal surgeons particularly rhinoplasty surgeons understand the pathophysiology of internal and external nasal valve since any disturbance in any portion of the critical sites in the nasal valve area (external valve and/or internal valve) can produce disturbed nasal breathing during exercise and/or at rest resulting in a very unhappy patient. The abnormalities responsible for nasal airway dysfunction include:
1.
The external nasal valve: dysfunction may be due to a flaccid external valve which collapses at low inspiratory (negative pressures) airflow rates which can occur when there is a loss of cartilage (lower lateral cartilage) support due to surgical or nonsurgical trauma, the effects of aging with atrophy of the lower lateral cartilages. A flaccid external nasal valve can also occur secondary to facial palsy. Avoid “over-resection” of the lower lateral cartilages since over-resection may lead to loss of structural support with early collapse during inspiration and postoperative breathing difficulties (Table 36.1).
2.
The internal nasal valve: dysfunction may be due to valve area narrowing due to structural changes in the valve angle (upper lateral cartilage and/or septal cartilage) or any one of the structural components of the valve area (Table 36.2).
3.
Combinations of structural skeletal (cartilage) changes and/or the absence of skeletal support (especially secondary to surgery) including avulsion of the upper lateral cartilage (Parkes and Kanodia 1981) which may be associated with early collapse of the valve during nasal airway breathing; however, often the airway obstruction is so severe that the patient is unable to breathe through the nose and becomes a mouth breather.
Table 36.1
Structures of the external nasal valve
1 | Lower lateral cartilage (LLC) |
2 | Fibroareolar soft tissues of ala |
Table 36.2
Structures of the internal nasal valve area
1 | Nasal septum (including premaxillary wings) |
2 | Upper lateral cartilage (ULC) |
3 | Piriform aperture |
4 | Fibroareolar lateral soft tissues |
5 | Frontal (ascending) process of the maxilla |
6 | Head of the inferior turbinate |
7 | Mucosa and skin coverings of these structures |
After blunt trauma (Barrs and Kern 1980), the second most likely cause of nasal valve abnormalities (with breathing problems) is nasal surgery (Barelli et al. 1987; Beekhuis 1976; Helal et al. 2010; Huizing and de Groot 2003; Nunez-Fernandez D 2999; Parkes and Kanodia 1981; Sheen 1983). Narrowing of the internal nasal valve angle by a medially displaced upper lateral cartilage after “hump” removal and infracture, additionally over-resection of the “hump” leaving an incomplete infracture, results in the “open roof” deformity (Barelli et al. 1987; Hinderer 1971; Javanbakht et al. 2012) and early collapse during inspiration in addition to posttraumatic neurogenic pain syndromes. Uncorrected septal pathology or over-resection of septal cartilage resulting in a “flaccid” septum with “flutter” is another cause of a collapsed internal nasal valve. Over-resection of the lower lateral cartilages may also result in “flaccid” collapse during inspiration. This “flaccid” collapse is also seen with loss of muscular action (as in facial paralysis) or with the atrophy of aging secondary to decreased tensile strength of the cartilages and soft tissues producing a “droopy tip” (Aksoy et al. 2010).
36.5 A Brief Word About the Preoperative Evaluation and Discussion
When rhinoplasty is considered, I take a considerable amount of time to develop a personal relationship (rapport) with the patient while obtaining a general medical history, supplemented with a rhinologic questionnaire (Kern 1972) which succinctly covers the essentials including a history of smoking and alcohol use, attention to allergic conditions (including seasonal and perennial allergic rhinitis, allergies to medications, soaps, surgical solutions, latex, tape), bleeding tendencies, use of steroids (topical and/or systemic), diabetes, hypertension, current and recent medications (including aspirin, aspirin-like drugs – nonsteroidal anti-inflammatory drugs), peripheral vascular disease, and previous operations of any kind.
In addition to physical nasal examination (looking for a Cottle sign Heinberg and Kern 1973) also use the endoscope (0 ° 4 mm) for an intranasal examination before and after topical decongestion to study the mucosa and the interior of the nose back to the nasopharynx. I usually obtain the following tests and consultations (when medically indicated):
1.
Minnesota Multiphasic Personality Inventory (MMPI) is obtained on all patients prior to surgery looking for evidence of personality disorders, schizophrenia, and psychosis (Goin and Goin 1981).
2.
Olfaction testing is performed on all patients.
3.
Rhinomanometry (anterior mask technique) is also performed on all patients prior to surgery based on techniques previously described (Broms 1982; Gordon et al. 1989; Kern 1973, 1977b; McCaffrey and Kern 1986; Pallanch et al. 1985). Other surgeons suggest the use of acoustic rhinometry (first introduced by Hilberg and associates in 1989) as an objective “evaluation of the nasal cavity geometry by acoustic reflections” (Corey 2006; Grymer 1995; Roithmann et al. 1997).
4.
CT scanning (direct coronal) is ordered when indicated.
5.
Preoperative photographs are ordered on ALL patients even prior to sinus surgery as the patients can occasionally “forget” how their face and nose appeared prior to surgery.
6.
Blood studies and other medical consultations (including allergy evaluation) are ordered when indicated.
I always ask for the patient’s parent(s) or significant other to be present before surgery, and I always welcome questions from the patient and family before ending the interview. The preoperative discussion is critical to establishing the necessary rapport with the patient and the family to better manage complications should they occur postoperatively. Succinctly, for cosmetic rhinoplasty, I ALWAYS discuss the following in detail:
1.
Goal(s) of the operation
2.
Risk(s) (to life) of the operation and the anesthesia
3.
Possible complications of the operation
4.
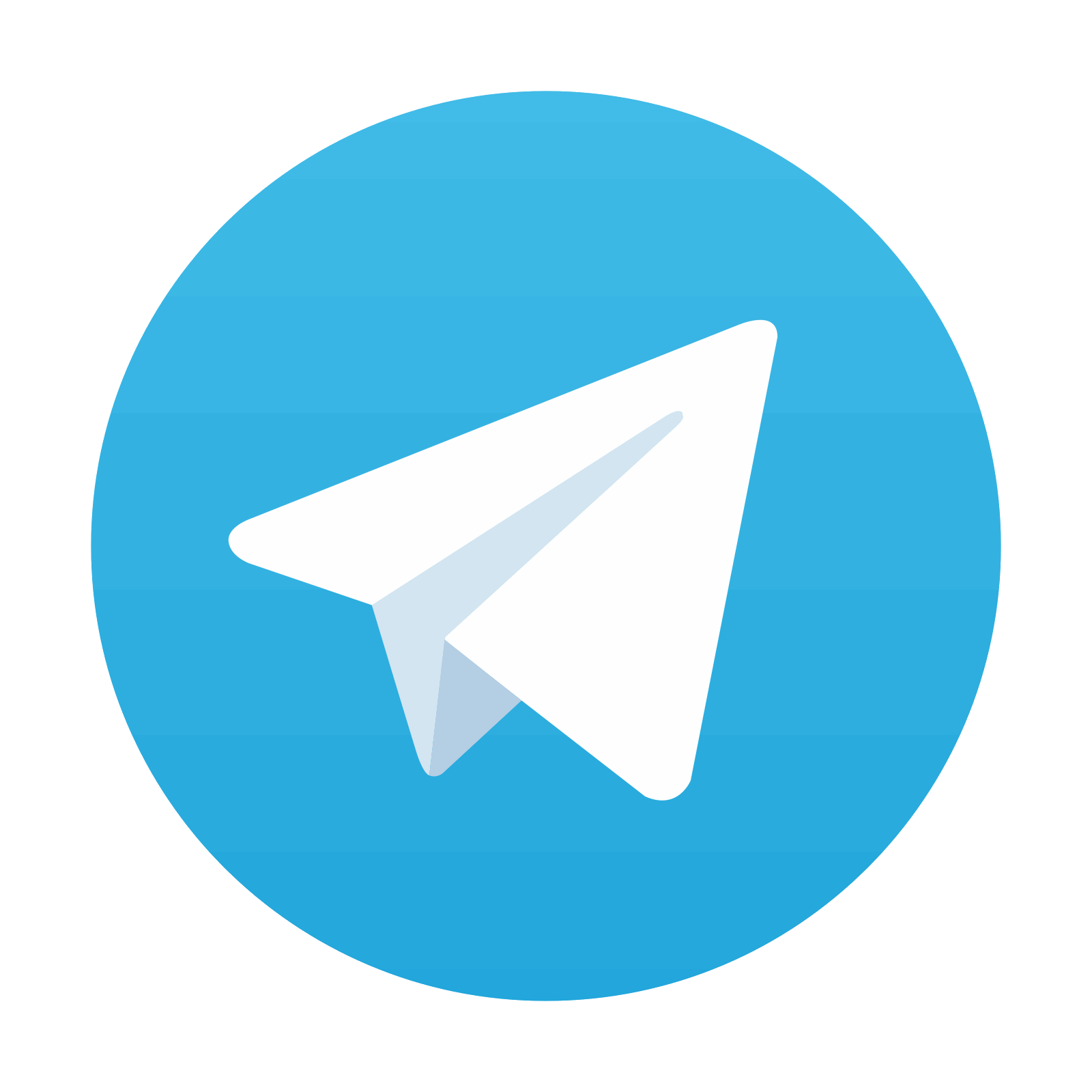
Possible need for nasal septal surgery (Kern 1980; Lipton and Kern 1990) to treat a current breathing disorder or the possible need for obtaining graft material from the patient such as cartilage and/or bone from the septum, ear, or rib for reconstruction and grafting (Breadon et al. 1979; Pirsig et al. 2004; Sherris and Kern 1998; Slavit et al. 1995)
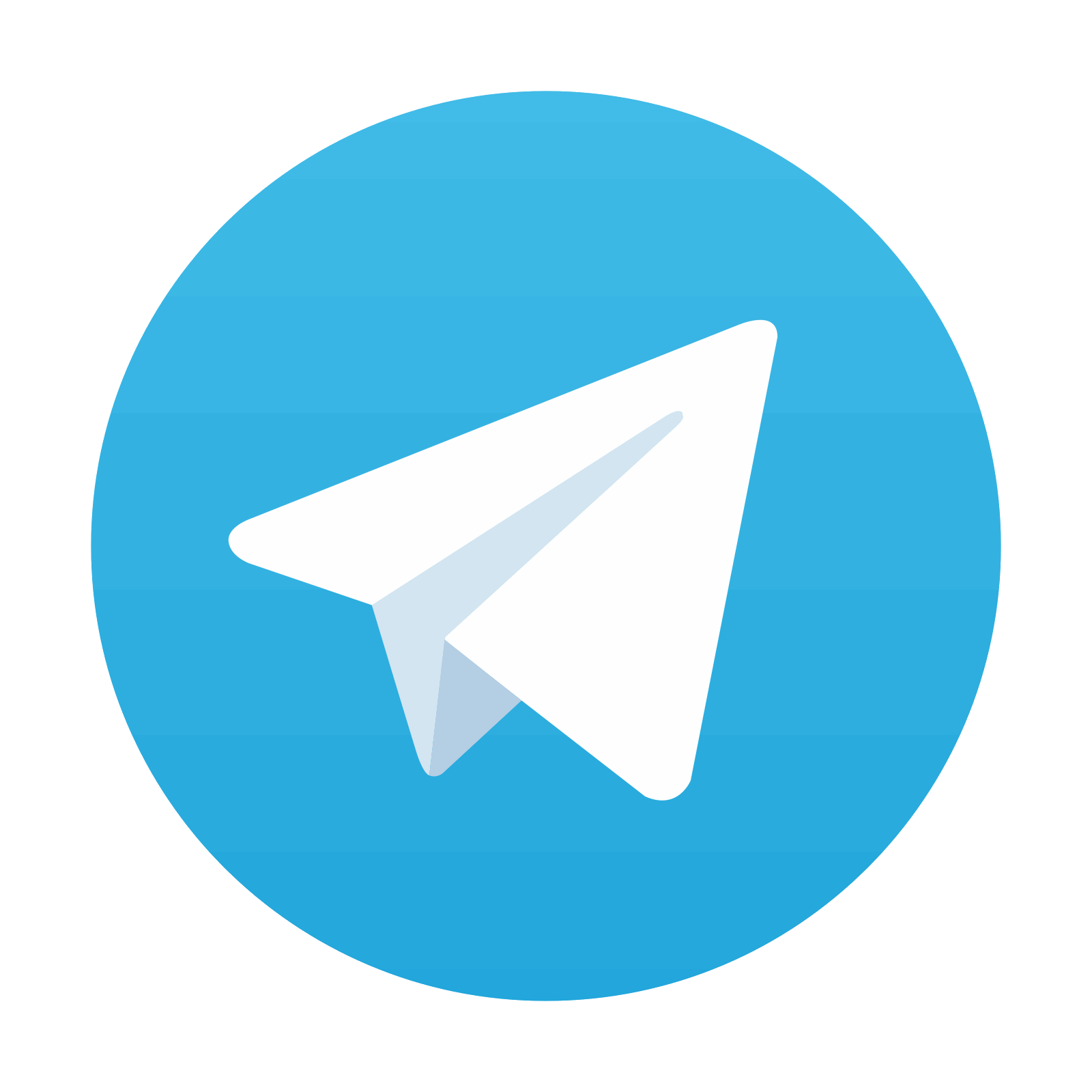
Stay updated, free articles. Join our Telegram channel

Full access? Get Clinical Tree
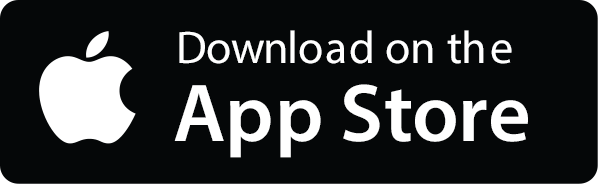
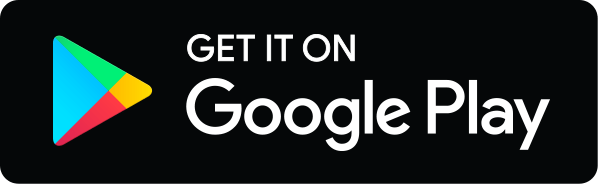
