34
Pharmacotherapy of Choroidal Neovascularization
Wayne W. Wu
Franco M. Recchia
Paul Sternberg Jr.
MECHANISM OF CHOROIDAL NEOVASCULARIZATION
Angiogenesis is the formation of new blood vessels by the sprouting and splitting of existing vessels, a process that is implicated in both normal physiological processes such as the female reproductive cycle, hair growth, and wound healing, and in diseases such as cancer, psoriasis, rheumatoid arthritis, and various ocular conditions (1). Retinal angiogenesis is central to such conditions as proliferative diabetic retinopathy, advanced retinopathy of prematurity, neovascular glaucoma, and proliferative sickle cell retinopathy (2). Neovascular (exudative or “wet”) age-related macular degeneration (AMD) arises most often from choroidal neovascularization (CNV) or choroidal angiogenesis. While the most common cause for visual impairment from retinal neovascularization is vitreous hemorrhage, CNV most commonly leads to subretinal hemorrhage and only rarely leads to vitreous hemorrhage. Similarly, while retinal neovascularization can lead to preretinal fibrous proliferation and traction retinal detachment, CNV frequently leads to subretinal fibrous proliferation with disciform scar formation.
However, the molecular mechanism underlying retinal neovascularization and CNV share similarities, and much of our knowledge of the pathophysiology and therapeutics of CNV arises from experimental models of retinal angiogenesis. Normally, endothelial cells lining the blood vessels are “silent” as a result of an intricate balance of pro-angiogenic and anti-angiogenic (angiostatic) factors. Pathological conditions such as hypoxia and ischemia perturb this balance and lead to an increased expression of pro-angiogenic factors and/or a down-regulation of angiostatic factors (3–5). The resulting angiogenic drive “activates” endothelial cells and precipitates a complex cascade of cellular events: proliferation of endothelial cells, migration of endothelial cells, tube formation, and ultimately formation of blood vessel loops from endothelial cell tubes (Fig. 34-1). Many stimulatory and inhibitory molecules are thought to be involved in this complex cascade: growth factors, adhesion molecules, extracellular matrix components, proteinase enzymes, upstream and downstream regulators, and others (4). Therapies can be targeted at one or more steps of the angiogenic process.
Figure 34-1. The resulting angiogenic drive “activates” endothelial cells and precipitates a complex cascade of cellular events: proliferation of endothelial cells; migration of endothelial cells; tube formation; and ultimately formation of blood vessel loops from endothelial cell tubes. (From http://angio.org/understanding/understanding.html. © 2000 The Angiogenesis Foundation, Inc. All rights reserved.).
Angiostatic factors include thrombospondin, angiostatin, endostatin, and pigment epithelium-derived factor (PEDF) (3, 4). Identified pro-angiogenic factors include vascular endothelial growth factor (VEGF), fibroblast growth factor (FGF) families, transforming growth factors-alpha and -beta (TGF-alpha and TGF-beta), angiopoietin-1 and -2 (3, 4). Members of the VEGF gene family include VEGF-A, VEGF-B, VEGF-C, VEGF-D, VEGF-E, and placental growth factor (PIGF) (6). VEGF-A has been shown to play a key role in normal vasculogenesis and in angiogenesis in animals and humans, while the role of other members of the VEGF family appears more limited (6, 7). VEGF-A was first described in 1983 as a “tumor vascular permeability factor” (VPF) that induced vascular leakage in skin (8). VPF was subsequently found to be identical to an endothelial cell mitogen named VEGF (9). Therefore, VEGF-A has two important functions: stimulation of angiogenesis and enhancement of vascular permeability. Both these properties are important in the pathogenesis of CNV in neovascular AMD.
As a result of alternative splicing and cleavage of signal sequences, multiple isoforms and cleavage products of VEGF-A exist. There are six major isoforms consisting of 121, 145, 165, 183, 189, and 206 amino acids (10, 11). VEGF121 is the highly diffusible isoform, while VEGF165 is the most abundantly expressed in humans with only a portion of it being diffusible (12). Both VEGF189 and VEGF206 are almost completely sequestered in the extracellular matrix (12). While all isoforms are important in angiogenesis, VEGF165 has been purported to be the isoform most allied with neovascularization (10, 13). All isoforms can be mobilized through extracellular cleavage by plasmin to generate the diffusible VEGF110 (14).
The biological effects of VEGF-A are mediated through the VEGF-specific tyrosine-kinase receptors VEGFR-1 (Flt-1), VEGFR-2 (Flk-1), and VEGFR-3 (Flt-4), and the neuropilins (NP1, NP2) (Fig. 34-2) (6, 15). VEGFR-2 appears to be the main receptor responsible for the angiogenic effects of VEGF-A. NP1 may enhance the effectiveness of VEGFR-2-mediated signaling by presenting isoform VEGF165 to VEGFR-2 (16). In humans, VEGFR-3 is thought to play a role in lymphangiogenesis (17).
Figure 34-2. The biological effects of vascular endothelial growth factor (VEGF)-A are mediated through the VEGF-specific tyrosine-kinase receptors VEGFR-1 (Flt-1), VEGFR-2 (Flk-1), and VEGFR-3 (Flt-4), and the neuropilins (NP1, NP2).
Laboratory and clinical findings implicate VEGF-A in the pathogenesis of neovascular AMD. VEGF-A expression can be induced in cultured retinal pigment epithelial (RPE) cells under hypoxic conditions (18, 19). Increased VEGF-A concentrations are found in surgically excised CNV membranes (20), in vitreous samples from patients with submacular hemorrhage and AMD (21), and in RPE cells in the early stages of AMD (22).
There is increasing evidence supporting the role of inflammation in both nonneovascular and neovascular stages of AMD (23, 24). The complement system is part of the innate immune system. Polymorphisms in the gene encoding complement factor H, one component of the native immune system, are strongly associated with subtypes of neovascular AMD (25). An illustration of current understanding of the pathogenesis of neovascular AMD is depicted in Figure 34-3.
TREATMENTS DIRECTED AGAINST VASCULAR ENDOTHELIAL GROWTH FACTOR AND ITS RECEPTORS
The primary target for pharmacologic treatment of CNV in AMD has been VEGF, and strategies have been devised to interrupt different steps along the VEGF signaling pathway (Fig. 34-2). Blockage of VEGF effect may be achieved by extracellular inactivation of VEGF-A by direct binding or “trapping,” by intracellular inhibition of VEGF gene transcription, or by inhibition of VEGF tyrosine receptor kinases.
INACTIVATION OF EXTRACELLULAR VASCULAR ENDOTHELIAL GROWTH FACTOR
Pegaptanib
Pegaptanib sodium (Macugen, OSI/Eyetech Pharmaceuticals, New York, NY, USA) was the first anti-VEGF agent approved by the United States Food and Drug and Administration (FDA) for the treatment of neovascular AMD. Pegaptanib is a ribonucleic acid (RNA) aptamer, a sequence of 28 ribonucleotides generated through a systemic evolution of ligands by exponential enrichment (SELEX) to achieve high specific affinity for VEGF165 (26). The attachment of a polyethylene glycol (PEG) moiety increases the half-life of the compound to provide a desired intraocular concentration in excess of minimal inhibitory concentration for VEGF165 for approximately 6 weeks (27, 28). Unlike peptides and monoclonal antibodies, an important feature of aptamers such as pegaptanib is that they are essentially nonimmunogenic even when administered in excess of therapeutic doses.
The VEGF Inhibition Study in Ocular Neovascularization (VISION) involved two concurrent, prospective, randomized, double-masked, sham-controlled, dose-ranging Phase III clinical trials of intravitreal pegaptanib for the treatment of neovascular AMD (Table 34.1) (29). A total of 1,186 patients were randomized 1:1:1:1 to receive pegaptanib (0.3 mg, 1.0 mg, or 3.0 mg) or sham injection every 6 weeks for 48 weeks. The primary endpoint was the proportion of patients losing fewer than 15 letters of visual acuity at 54 weeks. Compared with those receiving sham injection, patients treated with 0.3 mg pegaptanib were significantly more likely to lose fewer than 15 letters of visual acuity (70% vs. 55%), more likely to maintain or improve visual acuity (33% vs. 23%), and less likely to lose 30 or more letters of visual acuity (10% vs. 22%). However, only 6% of treated patients gained 15 letters or more in visual acuity. Serious adverse events were primarily those associated with intravitreal injection: endophthalmitis, in 1.3% of patients; lens trauma, in 0.7%; and retinal detachment, in 0.6%. These events were associated with a severe loss of visual acuity in 0.1% of patients. Two-year results of the VISION study confirmed the benefit of 0.3 mg pegaptanib in reducing moderate visual acuity loss and progression to legal blindness. Advantages of pegaptanib over the prevailing standard of care, photodynamic therapy (PDT) with verteporfin, included its equal efficacy for all angiographic types of CNV and the relative ease and portability of the treatment (30). However, the treatment only demonstrated moderate improvement in visual outcome for patients and requirement of treatment at 6-week intervals versus 3-month intervals for PDT.
Figure 34-3. The current understanding of the pathogenesis of neovascular age-related macular degeneration (AMD). FGF, fibroblast growth factor; HIF, hypoxia inducible factor; MMP, matrix metalloproteinases; TNF, tumor necrosis factor; VEGF, vascular endothelial growth factor.
Ranibizumab
Approximately 18 months following the release of pegaptanib, the FDA approved ranibizumab (Lucentis, Genentech, Inc., South San Francisco, California, USA), for the intravitreal treatment of neovascular AMD on June 30, 2006. Ranibizumab is the active portion (antibody fragment, or Fab) of a full-length humanized murine monoclonal antibody directed against all isoforms and degradation products of human VEGF-A (31). A closely related molecule, bevacizumab (Avastin, Genentech, Inc., South San Francisco, California, USA), had previously been approved for the treatment of metastatic colorectal cancer (32). Ranibizumab was developed because it was thought that its smaller size would afford better retinal penetration and more precise targeting of subretinal CNV (33, 34). Additionally, its lack of a fragment crystallizable (Fc) region should eliminate binding of ranibizumab to Fc gamma receptors and avoid a complement-mediated immune response.
Ranibizumab was studied in two Phase III, multicenter, double-masked, randomized, sham-controlled clinical trials of patients with subfoveal CNV from AMD (Table 34-1) (35, 36). In the Anti-VEGF Antibody for the Treatment of Predominantly Classic Choroidal Neovascularization in AMD (ANCHOR) study (36), 423 patients were randomly assigned patients in a 1:1:1 ratio to receive monthly intravitreal injections of ranibizumab (0.3 mg or 0.5 mg) plus sham PDT with verteporfin or monthly sham injections plus active PDT. At 12 months, 94.3% of those in the 0.3 mg group and 96.4% of those in the 0.5 mg group lost fewer than 15 letters, as compared with 64.3% of those in the verteporfin group. Mean visual acuity increased by 8.5 letters in the 0.3 mg group and 11.3 letters in the 0.5 mg group, as compared with a decrease of 9.5 letters in the verteporfin group. Perhaps the most exciting result was that visual acuity improved by 15 letters or more in 35.7% of the 0.3 mg group and 40.3% of the 0.5 mg group, in contrast to 5.6% of the verteporfin group. A subgroup analysis of 12-month data from the ANCHOR study showed ranibizumab to be superior to PDT in all evaluated subgroups (37).
TABLE 34-1 PUBLISHED PHASE III CLINICAL TRIALS OF PHARMACOLOGICAL TREATMENT OF CHOROIDAL NEOVASCULARIZATION SECONDARY TO AGE-RELATED MACULAR DEGENERATION
Similar results emerged from the Minimally Classic/Occult Trial of the Anti-VEGF Antibody Ranibizumab in the Treatment of Neovascular AMD (MARINA) study (35), in which 716 patients were randomly assigned to receive 24 monthly intravitreal injections of ranibizumab (either 0.3 mg or 0.5 mg) or sham injections. At 12 months, 94.5% of the patients in the 0.3 mg group and 94.6% of those in the 0.5 mg group lost fewer than 15 letters, as compared with 62.2% of patients in the sham injection group. It is noteworthy that 24.8% of the 0.3 mg group and 33.8% of the 0.5 mg group experienced visual acuity improvement by 15 or more letters, as compared with 5.0% of the sham injection group. Mean increases in visual acuity were 6.5 letters in the 0.3 mg group and 7.2 letters in the 0.5 mg group, as compared with a decrease of 10.4 letters in the sham injection group. The benefit in visual acuity was maintained at 24 months. Retrospective, subgroup analyses of 24-month data from the MARINA study and 12-month data from the ANCHOR study demonstrated that the most important predictors of improved visual acuity outcome were, in decreasing order of importance, worse baseline visual acuity score, smaller CNV lesion size, and younger age (37, 38).
The primary ocular adverse events associated with intravitreal ranibizumab were presumed to be endophthalmitis and uveitis, each occurring with a frequency of roughly 1%. The incidence of systemic thromboembolic events (0.7% to 2.2%) was approximately equal among all groups, but the studies were not sufficiently powered to detect significant differences in uncommon events.
Ranibizumab is the first treatment for neovascular AMD to improve visual acuity in a significant number of patients regardless of lesion size or angiographic subtype. What remains unclear is the optimal frequency and duration of treatments. The pivotal MARINA and ANCHOR studies employed monthly injections over 2 years. On the basis of collective clinical experience, however, there is wide variability in patient response to treatment, as some patients require monthly injections to maintain their level of visual acuity, while others may require only bimonthly or quarterly injections. It is hoped that this ambiguity may be clarified by ongoing studies of variable dosing regimens (39).
Bevacizumab
Encouraged by the results of the ranibizumab treatment and awaiting its FDA approval, Rosenfeld and other researchers (40–42) explored the off-label use of bevacizumab. The higher molecular weight and presence of two antigen-binding domains as well as the longer systemic half-life of bevacizumab suggested that its intravitreal half-life may be longer than that of ranibizumab. An open-label, uncontrolled clinical study showed short-term efficacy of systemic bevacizumab, with elevated blood pressure as the primary adverse effect (40). Subsequently, a few small, uncontrolled trials and retrospective studies suggested that an intravitreal injection of bevacizumab (typically 1.25 mg) was well tolerated and associated with improvement in visual acuity, reduction in macular thickening, and reduction in angiographic leakage in most patients (41, 42). The primary advantage of bevacizumab over ranibizumab is its significantly lower cost, since approximately 40 intravitreal doses can be obtained from a single vial of bevacizumab. However, questions regarding the efficacy and long-term safety of bevacizumab remain and will only be answered definitively by controlled clinical trials. The first of the Comparison of AMD Treatment Trials (CATT), a pivotal clinical trial involving comparison of both fixed and interval dosing regimens of bevacizumab versus ranibizumab, is presently underway.
Vascular Endothelial Growth Factor Trap
VEGF-trap R1R2 (Regeneron, Tarrytown, New York, USA) is a recombinant soluble receptor protein in which the immunoglobulin (Ig) domain 2 of the VEGF receptor VEGFR-1 and Ig domain 3 are combined with the Fc portion of human IgG1 (43). The receptor-binding domains confer high specific affinity (Kd < 1 pm) for all isoforms of VEGF-A, all other forms of VEGF (B, C, D), and PIGF (placental growth factors 1 and 2). Therefore, VEGF-trap differs from ranibizumab because of its high potency to neutralize all VEGF family members. The Fc portion slows the clearance of the drug by conferring a long circulating half-life of an Ig molecule to the fusion protein. Subcutaneous injection of VEGF-trap R1R2 (25 mg/kg) has been shown to effectively neutralize VEGF in mice with VEGF-secreting tumors (44). Intravenous or intravitreal administration of VEGF-trap into mice has also shown that VEGF-trap suppresses CNV and VEGF-induced breakdown of the blood-retinal barrier (45). The Clinical Evaluation of Anti-angiogenesis in the Retina (CLEAR) AMD-1 study was a randomized, multicenter, dose-ranging placebo-controlled Phase 1 clinical trial of intravenous VEGF-trap (46). Despite reduction of macular thickening, a dose-dependent increase in blood pressure among treated patients led to the discontinuation of this trial and to the development and clinical study of an intravitreal formulation. Phase 2 and 3 studies of intravitreal VEGF-trap are ongoing.
INHIBITION OF GENE TRANSCRIPTION OF VASCULAR ENDOTHELIAL GROWTH FACTOR AND ITS RECEPTORS
Small Interfering RNA-Based Therapies
Small interfering RNA (siRNAs) are double-stranded sequences of 21 to 23 ribonucleotides that assemble intracellularly into a multiprotein complex, termed RNA-induced silencing complex (RISC), to induce RNA degradation through a natural gene-silencing pathway called RNA interference (RNAi) (Fig. 34-4) (47). siRNAs have rapidly become the most widely used approach for gene knockdown because of their high potency. Chemically modified short siRNAs targeting VEGF-A or VEGFR-1 were shown to inhibit neovascularization in several preclinical models (48–50). Encouraging results from Phase I trials have been observed with siRNAs targeting VEGF-A (Cand5 [Acuity Pharmaceuticals, Philadelphia, Pennsylvania, USA] and ALN-VEG01 [Alnylam, Cambridge, Massachusetts, USA]) and with siRNA targeting VEGFR-1 (SiRNA-027, Sirna Therapeutics, Boulder, Colorado, USA).
INHIBITION OF VASCULAR ENDOTHELIAL GROWTH FACTOR RECEPTOR TYROSINE KINASES
VEGF signals through two major tyrosine receptor kinases: VEGFR-1 (flt-1) and VEGFR-2 (Flk-1 or KDR) (6). Some evidence suggests that flt-1 serves as a “decoy receptor,” regulating the amount of free VEGF available in the extracellular space, while VEGF binding to KDR causes receptor dimerization and autophosphorylation, and activation of downstream pathways essential for endothelial cell proliferation and angiogenesis (51, 52). Although VEGF clearly plays a central role in the development of neovascular diseases, other growth factor pathways acting through additional tyrosine kinases, such as platelet-derived growth factor receptor (PDGFR), fibroblast growth factor receptors (FGFRs), and Flt-4, have all been implicated in neovascularization and ocular disease (53, 54). Studies, which demonstrate that inhibition of these receptor kinases leads to more potent decreases in angiogenesis, suggest that inhibition of multiple tyrosine kinase receptors may be a desirable approach in treating ocular neovascularization (55).
Such compounds that have progressed to Phase I and I/II clinical trials include vatalanib (PTK787; Norvartis, East Hanover, New Jersey, USA), a potent inhibitor of all known VEGF receptor tyrosine kinases; AG-013958 (Pfizer, San Diego, California, USA), a selective inhibitor of VEGFR and PDGFR and; a series of novel indolyl quinolinones (Merck Inc., Whitehouse Station, New Jersey, USA) with activity against KDR and several other closely related tyrosine kinases.
Figure 34-4. Small interfering ribonucleic acids (SiRNAs) are double-stranded sequences of 21 to 23 ribonucleotides that assemble intracellularly into a multiprotein complex, termed RNA-induced silencing complex (RISC), to induce RNA degradation through a natural gene-silencing pathway called RNA interference (RNAi).
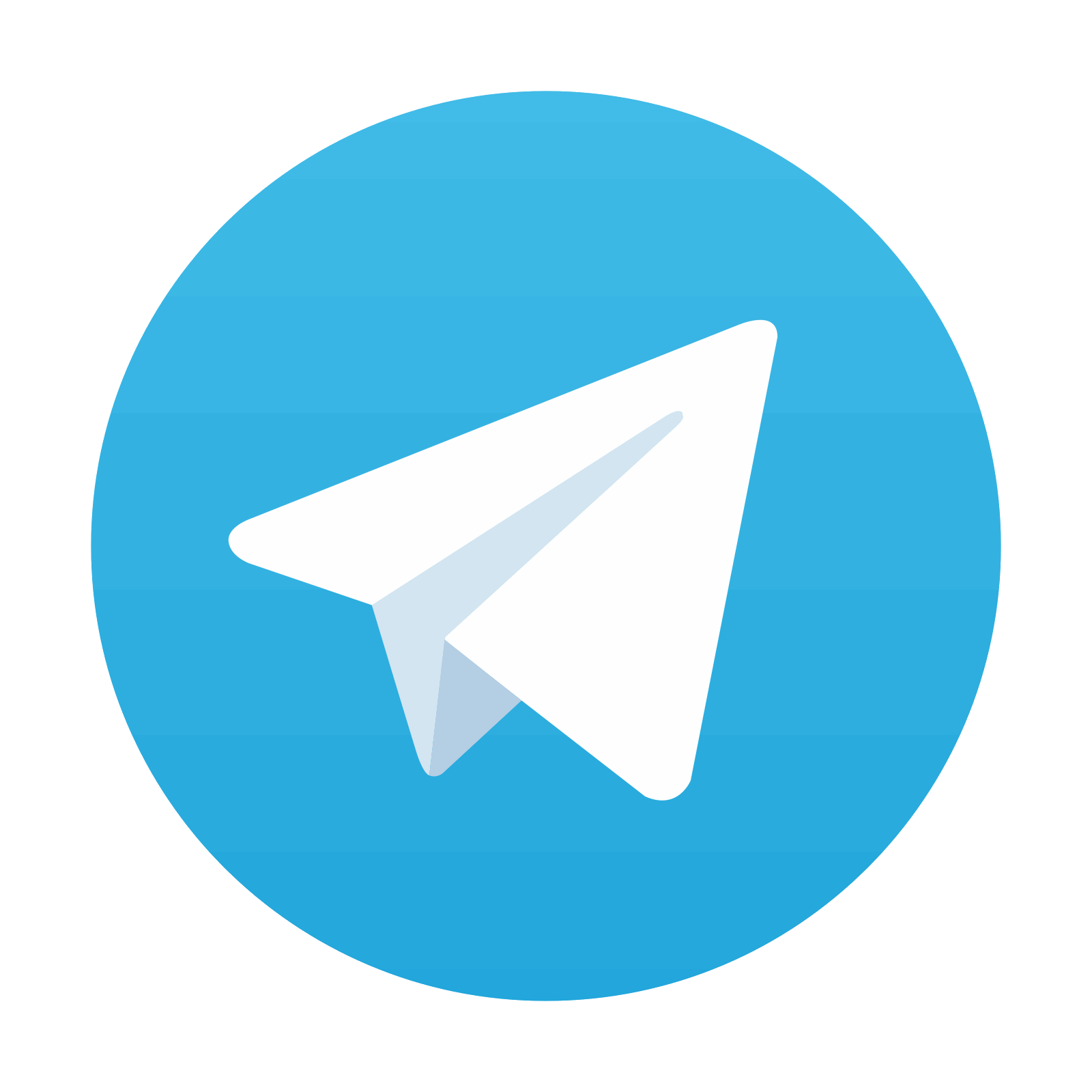
Stay updated, free articles. Join our Telegram channel

Full access? Get Clinical Tree
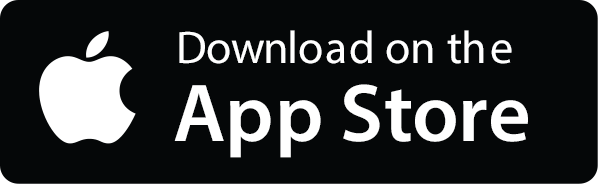
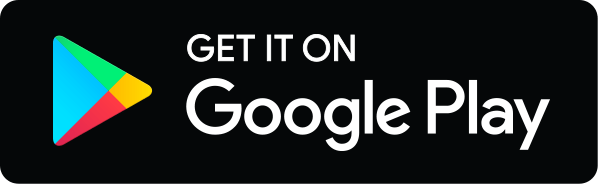