Introduction
Sensory nerves normally convey signals of pain to the brain. Yet, the overall experience and behavioral manifestations of pain are determined by mechanisms within the peripheral and central nervous system (CNS) that are complex and inadequately understood. The International Association for the Study of Pain defines pain as a “ subjective unpleasant sensory and emotional experience associated with actual or potential tissue damage, or described in terms of such damage .” In this context, pain is not strictly a mechanistic or biological phenomenon, but the experience of pain is influenced by complex emotional, behavioral, and psychosocial processes. The conscious experience of pain and its behavioral manifestations are also driven by environmental, social, and cognitive factors, which dictate the need for a “ holistic ” understanding of painful symptoms and for patient-centered, comprehensive, and multimodal therapies for combating pain.
There is no unique “pain,” but rather painful sensory and behavioral manifestations in various forms, driven by variable causes and pathophysiological mechanisms and thus requiring variable therapeutic approaches. Acute pain tends to originate from direct injury, which can be intentional (e.g., secondary to laser-assisted in situ keratomileusis (LASIK) or other ocular surgery) or unintentional (ocular trauma, infections, disease, noxious environmental exposure, actual dryness). Acute pain usually resolves with the healing phase (∼3–6 months), but in certain conditions can become recurrent or chronic. In contrast, chronic pain is persistent, by convention extending beyond the expected healing time, and is confounded by a complexity of emotional, behavioral, and psychosocial factors. Peripheral and central sensitizing mechanisms are one cause of chronic pain, and for most patients, cure is unlikely; this often leads to quality of life being negatively impacted for years on end (self-care and well-being, work, socialization, pleasure, relationships). Chronic pain may originate from prolonged application of noxious (tissue-damaging) stimuli causing persistent activation of specific receptors on nociceptive peripheral sensory fibers, which subsequently wind up the downstream pathways (nociceptive pain). On the other hand, it may originate from dysfunction within sensory nervous system itself, whether due to injury, disease, or other alterations (neuropathic pain). In either case, the pain sensation is amplified by overreactivity of peripheral or central nerves (e.g., peripheral and central sensitization, respectively), leading to persistent symptoms. ,
Anatomically, the sensory pathways that convey signals of pain from the periphery to the CNS consist of a chain of three afferent neurons. , Pain transmission from the oculofacial region involves afferent trigeminal pathways. The first-order (e.g., primary afferent) neuron responds to noxious stimuli applied to the peripheral oculofacial tissues, which sense noxious stimuli at the periphery (e.g., cornea) and convey the pertinent pain signals to the spinal trigeminal nucleus in the medulla (first-order neuron). The axons of the second-order neurons decussate and reach the thalamus (second-order neuron) via the contralateral trigeminothalamic tracts; at the thalamus, they synapse to third-order neurons, which project to subcortical and cortical centers of the brain (third-order neurons) that form part of the primary somatosensory cortex, where the sensation of pain is perceived. , Other pathways that are involved in modulation of pain simultaneously deliver inhibitory or excitatory signals from the brain to the periphery and thus influence how the pain is perceived, representing valuable therapeutic targets as pharmacological or electrical manipulation of the inhibitory circuits may be clinically useful in providing analgesia.
Dry Eye Disease
Dry eye disease (DED) constitutes “a multifactorial disease of the ocular surface characterized by a loss of homeostasis of the tear film, and accompanied by ocular symptoms, in which tear film instability and hyperosmolarity, ocular surface inflammation and damage, and neurosensory abnormalities play etiological roles.” Its pathogenesis is complex, involving a cycle of tear osmolarity changes, inflammatory damage, and tear film instability. Importantly, there exists heterogeneity in signs and symptoms of DED, thus DED may be understood as an “ umbrella ” term, characterized by multiple phenotypes with different symptoms, signs, and nerve findings. This variability is best exemplified by the lack of consistent relationships between symptoms and signs of disease, by the lack of a “ gold standard ” definition, and the overlap of corneal nerve parameters in individuals who are healthy compared to those with DED. , Despite this heterogeneity, the most common symptom of DED is ocular pain or discomfort; in fact, ocular surface pain is the leading cause of clinic visits and ophthalmic healthcare costs in patients with DED, and has deleterious effects on quality of life via impaired social functioning and decreased productivity. , As such, it is a large source of morbidity in the patient population of DED, and an important symptom to work-up when considering a new case of DED.
Ocular pain in DED falls into two categories, as introduced above—nociceptive and neuropathic pain. Nociceptive pain refers to pain that originates from activation of nociceptors secondary to a noxious stimulus at a peripheral nonneural tissue, implying an intact somatosensory nervous system. Conversely, neuropathic pain often arises secondary to a lesion or functional alteration along the somatosensory nerve supply to the cornea that originates from the trigeminal nerve. , Not infrequently, these two types of pain may coexist. While categorizing the type of pain can be difficult, one can glean this information (which may guide mechanism-specific treatments) by analyzing presenting signs and symptoms. For example, neuropathic-type ocular pain may be spontaneous pain, or may be evoked pain, triggered by wind and/or light (the ocular equivalents of tactile allodynia, and photophobia), and is often described with specific descriptors (burning, shooting, lancinating, stabbing). Besides this, pain out of proportion to ocular surface findings, persistence of pain despite treatment of potential nociceptive origins, and presence of specific comorbidities (fibromyalgia, irritable bowel syndrome, vulvodynia, interstitial cystitis, atypical facial pain disorders) may suggest a neuropathic cause.
Corneal Nerves and Pain Receptors
The cornea is the most densely innervated tissue in the body, containing nerves that function in mediating sensation as well as ocular protection and homeostasis. Corneal subbasal nerves originate from the ophthalmic branch of the trigeminal nerve, travel within its nasociliary and long ciliary nerve branches, and branch into nerve fibers that penetrate and innervate the cornea. These nerve fibers form thick bundles that approach the cornea radially to form the limbal plexus. At 1–3 mm away from the limbus, the nerves lose their perineurium and myelin sheath, aiding in corneal transparency. These stromal nerves, encased in only Schwann cells, run at a mean depth of approximately 300 μm from the corneal surface. Within the stroma, they extend laterally and anteriorly, running parallel to the collagen lamellae. , Branches form the anterior stromal plexus continue anteriorly to form the subepithelial nerve plexus (between Bowman’s layer and the anterior stroma), and then penetrate Bowman’s layer to form the subbasal nerve plexus, terminating near the corneal epithelium. , The total surface area of the subbasal nerve system is estimated at 90 mm 2 with 5400–7200 nerve bundles, with each bundle then separating into side branches containing three to seven individual axons each. Overall, this accounts for an estimated 19,000–44,000 total axons. Each nerve fiber houses 10–20 nerve terminals, and thus it is estimated that there are 315,000–630,000 terminals in the cornea, or approximately 7000 per mm. ,
The nerves that make up the subbasal nerve system also function in mediating sensation. The cornea contains several different nociceptive fibers (nociceptors) that are specialized to sense pain due to a variety of stimulus types, including mechanoreceptors (second most prevalent; ∼20% of total corneal sensory nerves), polymodal receptors (most prevalent; ∼70%), and cold receptors (least prevalent; ∼10%). Mechanoreceptors transmit sharp pain due to mechanical stimulation (e.g., object contacting the cornea) and are the fastest conductors of the three. Polymodal receptors respond to multiple modalities, including mechanical, thermal, and chemical stimuli, as well as local pH changes. Finally, cold nociceptors sense decreasing temperature and alterations in tear osmolarity. Pricking pain is sensed by mechanoreceptors, burning and stinging pain by polymodal receptors, and dryness by cold receptors. ,
Ascending Pathways of Pain
The somata of corneal nociceptors are located within the trigeminal ganglion, with centrally projecting axons synapsing in the trigeminal subnucleus caudalis/upper cervical transition zone (Vc/C1-2) and the subnucleus interpolaris/subnucleus caudalis (Vi/Vc). , Second-order axons travel from the spinal trigeminal nuclear complex, decussate, and terminate in the thalamus alongside contralateral spinothalamic fibers. , It should be emphasized that several neurons in the spinal trigeminal nucleus (mainly within the caudal subnucleus interpolaris, the transition zone between interpolaris and caudalis, and the rostral subnucleus caudalis) receive converging input from the cornea and from adjacent eyelid and facial skin, and can thus be activated by mechanical stimulation from either the ipsilateral cornea or from the periorbital skin. Their receptive fields expand after prolonged noxious stimulation , ; this altered sensory processing manifests as a hyperalgesic state, with enhanced pain to both normally nonnoxious and noxious stimuli across a wide area surrounding the eye and face. ,
This expansion of the receptive field is mediated centrally, and is partly due to attenuation of central inhibitory signals onto activated second-order neurons, to the extent that these neurons can be activated by subliminal inputs from the cornea or periorbital region. Clinically, this sensitization may account for chronic periocular pain that often accompanies corneal pain, as a result of increased excitability of second-order neurons and an expanded periorbital receptive fields with loss of central inhibition. Furthermore, it has been shown that not only nerves directly subjected to injury but uninjured nerves situated adjacent to those that are injured are involved in the pain experience ; as such, blockade of periorbital nerves may induce analgesia by suppressing ectopic firing and decreasing incoming convergent nociceptive traffic to the spinal trigeminal level. Finally, third-order neurons extend from the thalamus to areas of the primary somatosensory cortex (e.g., trigeminal ganglion, bilateral trigeminal nucleus caudalis, contralateral ventroposteromedial thalamus, anterior cingulate cortex, and middle frontal gyrus), where the sensation of pain is finally processed. , ,
Central-level processing of pain signals includes interactions between various subcortical and cortical centers involved in the pain response. , These include the somatosensory SI and SII regions, anterior insula, and anterior cingulate cortex. , , , On the other hand, affective-motivational responses to pain are mediated by the limbic system, including the hypothalamus, which normally regulates autonomic and neuroendocrine responses to pain. , , Several other projections involved in autonomic, homeostatic, and behavioral responses after tissue‐damaging stimuli have been identified, such as those that extend to the parabrachial nuclei. , It has even been speculated that pain originating from the peripheral cornea may be preferentially conveyed via a nonthalamic ascending pathway via corneal afferents that activate parabrachial-projecting second-order trigeminal neurons rather than trigeminothalamic ones, although it is also possible that ascending transmission is polysynaptic, with projections at both sites. These polysynaptic pathways may explain why corneal pain is not infrequently poorly localized, and is often referred to adjacent periocular regions, and may also offer some explanation of the strong emotional and aversive dimensions related to corneal pain.
Interestingly, these sensory pathways also interact with other pathways involved with processing the sensation of light, explaining why photophobia frequently accompanies ocular pain ( Fig. 16.1 ). These pathways involve projections from intrinsically photosensitive retinal ganglion cells to thalamic neurons, which also receive converging input of pain signals from the trigeminal nucleus caudalis, and subsequently project to the cortex. At this level, the thalamus not only relays signals to the cortex but also dynamically integrates and amplifies all these signals thus constituting a neurophysiological substrate that can both generate pain amplification and also contribute to photophobia. ,

Of note, these denoted pathways of pain are not hardwired signal relays, and are capable of signal modulation. Dynamic plasticity within the periphery, medulla, thalamus, and higher centers of the brain allows for dramatic alterations in the relation between the intensity of the painful stimulus and the responses to this pain. The interplay between incoming signals, excitatory signal modulation, and inhibitory signal modulation eventually determines the intensity of perceived pain, as well as the physiologic response to it. , Depending on circumstances, the response to the incoming messages may be attenuated or amplified compared to the original signal. Amplification of the pain signal, or onset of spontaneous pain, often occurs in DED as a result of peripheral or central sensitization of nociceptive neurons.
Ascending nociceptive signals can be modulated by descending inhibition from the CNS, leading to attenuation of the incoming afferent input and thus the perceived intensity of pain. For example, the periaqueductal gray contains opioid peptides and receptors which serve as a mediator for opioid-induced analgesia; their release directly decreases the sensation of incoming pain. On the other hand, other higher centers (e.g., cerebral cortex, limbic system, nucleus raphe magnus, locus coeruleus) also involved in analgesia in response to incoming nociception similarly activate descending pathways that instead lead to analgesia via inhibition of nociceptive processing at the trigeminal/spinal dorsal horn. This inhibition occurs via serotoninergic or noradrenergic fibers that release serotonin and norepinephrine which act as inhibitory neurotransmitters, a process that can be targeted pharmacologically (via tricyclic antidepressants, selective norepinephrine serotonin reuptake inhibitors, or tramadol). Finally, nociceptive stimuli drive activation of inhibitory interneurons leading to release of encephalin (endorphins), GABA, and glycine, which also work to modulate the incoming signal. ,
Nociceptive Pain
In general, the workup of a new painful case of DED begins with consideration of nociceptive sources of pain, and is usually carried out in the ophthalmologic clinic setting. Nociceptive pain is defined as “ pain that arises from actual or threatened damage to non-neural tissue and is due to the activation of nociceptor ,” and is thus due to direct noxious stimulus to the ocular surface. Implied in this definition is that underlying corneal nerves are healthy and functional, an important distinction from neuropathic ocular pain. The noxious stimuli trigger a nocifensive response which aims to protect the integrity of affected tissues by eliciting withdrawal responses or behavioral modifications. Different noxious stimuli at the level of the cornea will activate specific subsets of nociceptors, leading to discharges of impulses relayed to the CNS to encode the characteristics of the stimulus, to evoke pain sensations referred to the eye, and to mobilize defensive stimulus-avoiding behavioral responses. ,
Several types of noxious triggers to nociceptive pain exist, each corresponding to activation of a particular type of nociceptor as described above. Commonly observed stimuli include direct traumatic or surgical injury, tear film abnormalities (e.g., decreased tear production, high or unstable tear osmolarity, presence of inflammatory mediators), abnormal ocular anatomy of the surrounding eyelid and/or cornea (e.g., pterygium), or direct ocular surface toxicity by an external agent (air pollution, topical glaucoma medications). Importantly, nociceptive ocular pain tends to be acute and self-limited—once the exposure is removed, healing can begin, after which pain tends to subside—in comparison to chronic nociceptive pain, which is more persistent. It is important to note, that under specific circumstances, prolonged exposure to a nociceptive stimulus can manifest as a more chronic type pain via sensitization, explored further below.
Acute Nociceptive Pain
In normal states, noxious stimuli generate an acute nociceptive pain response, characterized by a pain sensation proportional to the severity of the stimulus (although genetic and other factors may result in variability across individuals) and subsequent nocifensive behavioral responses aimed toward withdrawal and protection from the insult. These are “healthy,” physiologically adaptive responses, aimed at recognizing harmful stimuli and eliciting reflexive avoidance behaviors in order to avoid further exposure to the stimuli. , ,
In the cornea, some nerves respond selectively to cold stimuli, while others respond selectively to mechanical forces, but the majority (e.g., the most abundant neurons in the trigeminal system, classified as “polymodal nociceptors”) respond to a wide variety of stimuli such as heat, chemical irritants, and presence of heightened inflammatory mediators. , Pain receptors are specialized receptors coupled to cation channels, the opening of which generates electrical signals in the form of action potentials that successively propagate proximally toward central nerve terminals wherein they elicit neurotransmitter release and synaptic transmission of ascending pain signals. , ,
Several of such receptors, like transient receptor potential vanilloid 1 (TRPV1) and transient receptor potential melastatin member 8 (TRPM8), which respond to a variety of noxious stimuli, have been identified on both nociceptive fibers in the cornea as well as on nerves of the conjunctiva and eyelid. , The TRPV1 receptor responds to thermal, acidic, and chemical stimuli, generating nociceptive signals in response to activation that are perceived as burning or sharp painful sensations. , , , Other receptors transduce noxious heat, cold, mechanical, or chemical stimuli , and initiate the signals that will enable the nervous system to distinguish these stimuli as painful. For example, TRPM8 thermoreceptors expressed in both the cornea and the eyelids are coupled to an ion channel which is activated by cold stimuli and/or osmolarity increases, and they mediate reflexive blinking as well as tear production. , TRPV1 coexist with TRPM8 in a high proportion of cold receptors and act to enhance neuronal firing to cold; interestingly, their expression in DED may be upregulated by inflammation, and may contribute to observed cold allodynia seen in certain cases of DED. Because activation of TRPM8 receptors may inhibit other aberrant nociceptive signals, their targeting by specific agonists, such as the topical agonist cryosim-3, has therapeutic potential in DED with related neuropathic pain. Yet, the opposite may also be the case, since TRPM8 antagonists may suppress acute and chronic pain including cold allodynia, , at the cost of worsening dryness.
The action potentials that are generated in response to noxious stimulation are conveyed to the CNS via synaptic neurotransmission from Aδ fibers and C fibers. Neurotransmitters involved in this synaptic transmission of pain include excitatory amino acids (e.g., glutamate or aspartate) and neuropeptides (e.g., calcitonin gene–related peptide [CGRP] and substance P [sP]). These proteins act on cognate postsynaptic receptors: glutamate mediates signaling by activating cation channels (e.g., α-amino-3-hydroxy-5-methyl-4-isoxazolepropionic acid [AMPA] receptor and N-Methyl- d -aspartate [NMDA] receptor), or by inducing secondary cytosolic messenger signaling (metabotropic receptors). Substance P binds to the neurokinin receptor 1 (NK 1 ) which regulate various mediators of cellular excitability and function. , , Acute nociceptive pain is usually signaled via synaptic release of excitatory glutamate acting at AMPA receptors, and minimally via sP acting at the NK1 receptor. CGRP is abundant in trigeminal neurons and secondary sensory neurons in trigeminal nucleus caudalis (TNC), and elicits postsynaptic responses via G protein–coupled receptors that drive multiple processes, including neurogenic inflammation (by recruiting immune cells, and sensitizing sensory neurons) in peripheral tissue, vasodilation, and signaling processes driving central sensitization. ,
Postsynaptic responses lead to brief discharge of action potentials and subsequent activation of discriminative-sensory ascending pathways. , , This is also the case in the trigeminal pain transmission, wherein TRPV1 induces release of CGRP and sP from trigeminal nerve terminals. The expression of sP and CGRP in trigeminal nociceptors is rapidly upregulated in response to inflammation, thus CGRP and sP contribute to the development of peripheral hyperalgesia, while CGRP receptor activation promotes activation of AMPA receptors, leading to increased firing of neurons and central sensitization.
Chronic Nociceptive Pain and Sensitization
In some instances, acute nociceptive pain may transition into a chronic pain state. Specifically, prolonged or intense nociceptive stimulation, or loss of normal sensation, may alter the properties of the somatosensory nerve pathways to such an extent that incoming pain signals are amplified to an inappropriate level, leading to spontaneous pain upon nonnoxious stimuli. , In contrast to acute pain, this occurs because tissue injury or persisting inflammation that is spatially or temporally extensive induces plasticity changes in neural pathways, generating chronic pain. This also applies to the cornea, wherein prolonged noxious stimuli and/or direct injury to corneal nerves may lead to altered nerve morphology and function, which is also associated with chronic pain.
Specific injuries or extensive inflammation can mobilize and activate immune cells, leading to release of numerous inflammatory mediators (e.g., prostaglandins, protons, potassium ions, bradykinin, cytokines, growth factors, purines, and amines; sometimes described as an “inflammatory soup”). , , This also includes generation of nitric oxide (NO) and proinflammatory cytokines (interleukins, tumor necrosis factor) that induce the enzyme COX2 synthase, which further contribute to the release of prostaglandins, and so-forth. Some of these mediators may function to activate nociceptors directly and evoke chronic pain, while others sensitize the nociceptors, promoting easier activation (by lowering their thresholds of activation) or enhanced responsiveness (by heightened membrane excitability) of receptors. ,
Sensitization refers to the phenomenon of ‘‘ increased responsiveness of nociceptive neurons to their normal input, and/or recruitment of a response to normally subthreshold in puts.” Prolonged or extensive tissue injury (due to noxious stimuli, inflammatory damage, hyperosmolarity, environmental exposures, postsurgical changes) can sensitize nociceptors and make them more responsive to stimuli. The sensitized sensory neural apparatus in turn fosters a hyperresponsive state, generating spontaneous pain and/or pain evoked by normally nonnoxious stimuli (allodynia), or responding with aberrantly excessive pain to a normally painful stimulus (hyperalgesia) which are interestingly shared symptoms of neuropathic ocular pain. , Some sensitization mechanisms can be rapid, subsequent to posttranslational modification (phosphorylation) of signal effectors such as membrane ion channels, while others are more delayed because they depend on altered transcription, translation, and transport of newly synthesized proteins to either distal or proximal terminals. , ,
Peripheral sensitization involves release of cytokines, prostaglandins, nerve growth factor, and bradykinin all of which act to increase TRPV1 expression and/or activity within sensory neurons. This is also the case in corneal pain and DED, wherein TRPV1 is upregulated in corneal nerves and within the trigeminal ganglion, in a fashion that correlates with various hyperalgesic responses. Then, ongoing nociceptive input from peripherally sensitized nerves as a result of persistent inflammation provides a sustained input to the second-order neurons and to higher centers. Sensitized C fibers may then release CGRP and sP, which promote prolonged excitatory postsynaptic responses and firing. On the other hand, central sensitization also involves a synergistic activation of both glutamate and sP or CGRP receptors, which if of sufficient magnitude and duration, can excite the NMDA receptor. , NMDA receptors are linked to Ca 2+ channels, and their activation results in prolonged release of cytosolic Ca 2+ , leading to Ca 2+ -dependent hyperactivity of secondary messenger cascades (e.g., activation of phospholipase A2 and subsequent mediators such as NO, inositol triphosphate (IP 3 ), diacylglycerol (DAG), activation of protein kinase C (PKC), and phosphorylation of various targets), leading to a series of events that range from genomic alterations to “burst” firing of spinal cord neurons and amplified transmission of nociceptive processing. , , Central neurons may then reach a state of heightened neuronal excitability that results in amplified sensation (hyperalgesia, allodynia, photophobia), spontaneous firing, and subsequently spontaneous pain.
Peripheral nociceptor activity at the level of the trigeminal ganglion processes centripetally to the trigeminal nucleus, leading to a sustained input at the central level. Downstream, this heightened traffic of incoming pain signals due to peripherally sensitized corneal nociceptors will in turn significantly enhance the responsiveness of central nociceptive pathways as well, by inducing a series of neuroplastic changes. Central sensitization often involves permanent changes in the CNS (at the level of neurons, interneurons, ventral horn neurons, thalamus, cortex, and other brain areas), leading to pain that persists after the original injury has healed. , , In contrast to physiologically adaptive acute pain, central sensitization is considered a process of maladaptive neuroplasticity, because it facilitates an amplified, exaggerated synaptic response to pain signaling as a result of enhanced excitatory neuronal activity and downregulation of inhibitory neuronal pathways. Pain and other neuro-behavioral manifestations of central sensitization do not serve any physiologically adaptive role, but rather contribute to ongoing dysfunction and suffering. This maladaptive pain is commonly seen in DED, specifically when symptoms do not mirror ocular surface findings.
Neuropathic Pain and Photophobia
In certain circumstances, eye pain is not a result of nociception from the ocular surface per se, but is rather caused by an injury or alteration to the nerves involved with processing of nociceptive pain, or to adjacent nerves. Several elements can be affected in this manner: nerve terminals, axons of nociceptive sensory neurons, sensory ganglia, and higher-order neurons (e.g., spinal cord, brain stem, thalamus, and cortical structures) involved with processing or responding to nociceptive input. This is termed neuropathic pain, defined as “ pain caused by a lesion or disease of the somatosensory nervous syste m.” In pure neuropathic pain, there is no association between pain and damage; pain often exists in the absence of any injury or stimulus, yet in other situations neuropathic pain may coexist with nociceptive pain, making it difficult to distinguish one from the other. Various diseases that may lead to lesions encompassing corneal nerves (LASIK or other ocular surgery, trauma, infections such as herpes zoster or acanthamoeba), or to the second- and third-order somatosensory system within the CNS (multiple sclerosis, stroke, tumor) can thus manifest as neuropathic pain. ,
The predominant abnormality that drives neuropathic pain is aberrant electrical hyper-excitability and generation of ectopic firing of sensory neurons after injury to somatosensory nerves, at any region along the nociceptive pain pathway. Subsequent alterations in translation and posttranslational modification of neuronal sodium channels and other channels , secondary to nerve injury have been implicated in the onset of neuropathic pain. Interestingly, given the underlying role of hyperexcitability, drugs that modulate channels involved in nerve excitation (e.g., sodium channel–blocking drugs) may have roles as analgesics; suppressing the initial afferent barrage by using a local anesthetic, for example, may attenuate the development of subsequent hyperalgesia. ,
Aberrant firing generates action potential that propagate centrally and these elicit spontaneous pain, and/or drive central sensitization, thereby contributing to abnormal pain perception, such as allodynia, hyperalgesia, and—additionally in the case of the corneal pain—photophobia. Inflammation or injuries affecting corneal nerves may lead to emergence of neuropathic pain and photophobia as a result of peripheral and central sensitization subsequent to persistent ongoing afferent trafficking that induces synaptic reorganization and functional alterations, both of which contribute to chronicity of pain. These alterations include neuroinflammation, astrocyte and microglial activation, and enhancement of excitatory synaptic transmission, resulting in sensitization of ocular-responsive neurons of the caudal trigeminal brainstem and also those in higher centers. This may explain several manifestations of central sensitization and centralized pain states, such as photophobia, migraines, fibromyalgia, and accompanying neurocognitive and emotional disorders. Deficient supraspinal or descending mechanisms may also be involved, such that excitatory input predominates over descending inhibitory pathways because pain-modulating tracts become weak. , Finally, other mechanisms have been also implicated, including neuroma formation at the sites of damaged peripheral nerves, which exhibit abnormal responsiveness and spontaneous discharges (ectopic firing) and thus driving neuropathic pain and sensitization. Activation of satellite glial cells that surround trigeminal ganglia may be another contributor to neuropathic ocular pain and hyperalgesia, as well as glial activation in the brain which is upregulated by CGRP-dependent mechanisms.
As brought up above, a common symptom of corneal neuropathic pain is the presence of light sensitivity, e.g., photoallodynia and photophobia. , The thalamic nuclei mediate the transmission of nociceptive pain from the periphery to the CNS as aforementioned, but some of these same areas overlap with areas that mediate the sensory processing of light. Specifically, incoming light is sensed by rods and cones within the retina, which synapse upon retinal ganglion cells that feed into the optic nerve; branches of this nerve stretch to the parabrachial nuclei, and from there the information eventually is relayed into the thalamic geniculate nucleus and the occipital cortex where the information is processed. Yet, some of these afferent fibers instead project to the lateral geniculate nucleus and eventually to the olivary pretectal nucleus, which activates the superior salivatory nucleus and sphenopalatine ganglion located downstream. This parasympathetic overdrive causes activation and sensitization of trigeminal afferents from the eye (via uveal vessel dilation as well as release of neuroinflammatory mediators (e.g., CGRP, vasoactive intestinal peptide, and NO)) which sensitize the corneal trigeminal afferents and higher-order neurons within the nucleus caudalis, thalamus, and higher brain centers, thus generating pain out or proportion. This overlap of light and pain pathways in sensory processing areas of the brain explains how light sensation may lead to increased pain signals, resulting in the manifestation of photophobia and photoallodynia. This would also explain noted observations that patients with DED and photophobia who protect their eyes with sun glasses and avoid intense light and wind tend to report less painful symptoms. ,
Distinguishing Nociceptive and Neuropathic Pain
As suggested, mechanisms of neuropathic pain have shared features with nociceptive pain; though these disorders manifest by vastly differently processes, they are tied to one-another by a common pathophysiology involving sensitization. , Central sensitization has been implicated in both nociceptive pain and neuropathic pain, directly leading to the presence of evoked pain over the initial site of injury and around the surrounding area (e.g., expansion of the receptive field). Despite the similarities, it is important to distinguish neuropathic from nociceptive pain in a case of DED, as their therapeutic ladders differ greatly. Several clinical features can be used to distinguish neuropathic from nociceptive pain. First, if ocular pain persists after nociceptive sources have been evaluated and treated, neuropathic pain should be considered. Besides this, if individuals display specific symptoms gleaned through the use of validated questionnaires (e.g., burning, tingling, wind and light sensitivity) and/or have comorbidities suggestive of corneal nerve damage (migraine, fibromyalgia, recent refractive surgery), neuropathic pain should also be considered. Finally, hyperalgesia (augmented pain response) and allodynia (pain due to a nonnoxious stimuli, e.g., to light [ photoallodynia and photophobia ], to cutaneous touch [ cutaneous allodynia ], to wind) are features also generally seen in individuals with neuropathic pain, , and directly occur as a result of central sensitization leading to pain from innocuous stimuli.
Conclusion
The ascending pathways of ocular pain are complex, consisting of multiple circuits of nerves stretching from the periphery (cornea) to the CNS (spinal cord and higher cortical centers of the brain), and back. The processing and eventual perception of ocular pain is equally as complex, being influenced by external (environment, cognition, mood) and internal (modulation by higher cortical centers) factors. Nevertheless, ocular pain is an important part of the DED experience, and clinicians should take great care in understanding the differences in pathophysiology and presentation of the types of pain seen in DED (e.g., nociceptive, neuropathic, or both) and how these types of pain may change over time (e.g., sensitization). This is especially important as these factors have implications on how one should therapeutically approach ocular pain in DED.
References
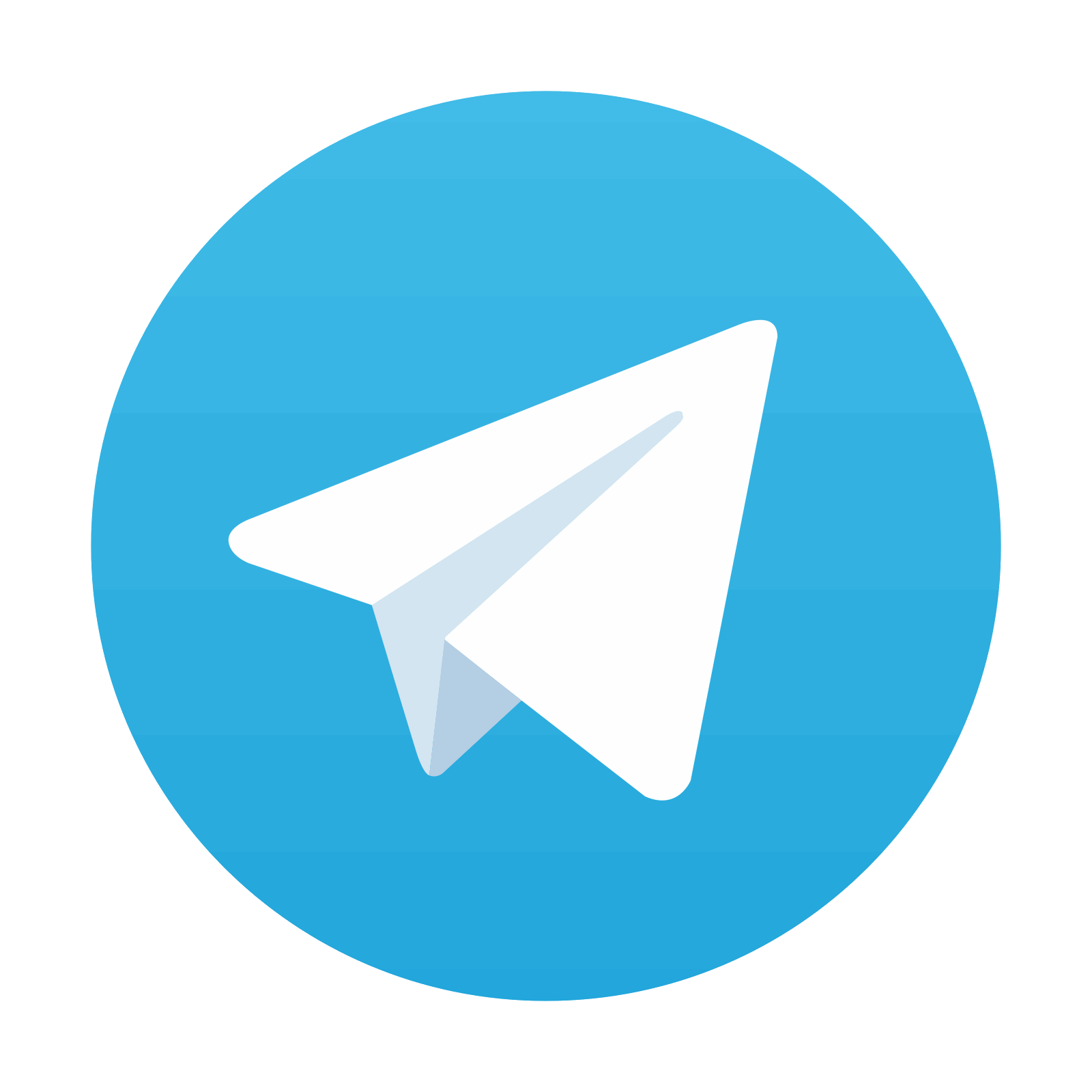
Stay updated, free articles. Join our Telegram channel

Full access? Get Clinical Tree
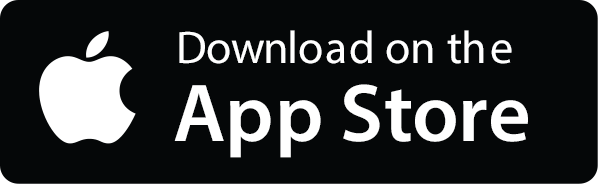
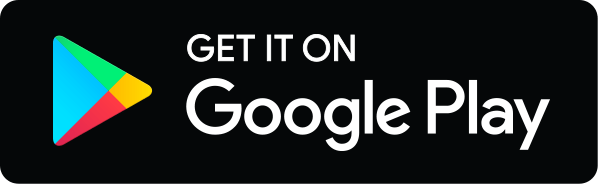
