Chapter 45 Pathophysiology of Recurrent Laryngeal Nerve Injury
Introduction
Laryngeal nerve injury is a severe and frequently encountered complication of thyroid surgery, and it is a source of considerable morbidity. The specific symptoms vary greatly over time and among patients. Patients with unilateral paralysis usually complain of hoarseness. Swallowing problems also occur, but less frequently. Both of these symptoms are due to inadequate glottal closure. These symptoms can sometimes improve over time. In contrast, bilateral nerve injury has its greatest impact on breathing, as the vocal folds fail to open during inspiration. Often airway distress increases over time, and patients who initially have an adequate airway may develop severe distress a few months later. The severity of symptoms is influenced by the degree of the insult, regeneration of the nerve, and compensatory functional reserve of the larynx. This chapter deals with the pathophysiology of recurrent laryngeal nerve (RLN) paralysis. Please also see chapter on clinical RLN paralysis management (see Chapter 46, Management of Recurrent Laryngeal Nerve Paralysis).
Variations in Symptoms
Just as in unilateral nerve injuries, there is a range of residual and regenerated activity of laryngeal muscles in patients with bilateral RLN injury. Patients frequently have some appropriate adduction with phonation, but abductor function is rare. Inappropriate adduction with inspiration can also occur, sometimes because of a passive inward collapse of flaccid paralyzed musculature during inspiration (i.e., the Bernoulli effect) or often the result of inappropriate contraction of adductor muscles (i.e., not normally activated during inspiration). Electromyography (EMG) can document this activity. It is recruited with increasing inspiratory effort, which suggests that it is due to reinnervation of adductor muscles by motor axons that previously supplied the posterior cricoarytenoid muscles (Figure 45-1).
Configuration of the Paralyzed Vocal Fold
A gradual shift of the paralyzed vocal fold toward the midline in the first several months after nerve injury is a phenomenon that has been recognized clinically for many years.1 This change in position is associated with improvement in the voice. However, in patients with bilateral laryngeal paralysis, the result is a diminishing airway (Figure 45-2).
Traditionally, the terms median and paramedian have been used to describe a paralyzed vocal fold that lies at or near the midline. A more abducted position is referred to as cadaveric. In general, vocal symptoms are directly related to the distance of the paralyzed vocal fold from the midline. The voice is weak when the glottis cannot close completely during phonation, and it becomes breathy or aphonic when the glottic gap is large. Surgical procedures such as thyroplasty and vocal fold injection move the anterior portion of the paralyzed vocal fold nearer the midline. This effectively restores vocal function in patients with a paramedian vocal fold position. But when the arytenoid is externally rotated, the paralyzed vocal process is too far lateral to permit approximation by the mobile contralateral vocal process (Figure 45-3). In such cases, adequate closure of the glottis requires surgery that addresses the posterior portion of the vocal fold as well.
An alternative to using the terms paramedian, cadaveric, or flaccid is to measure the angle formed between the vocal folds during inspiration. A line is drawn from the anterior commissure to the vocal process on each side. Together these lines form the anterior glottic angle. This parameter is a quantitative measure that is useful in preoperative assessment and in tracking the results of treatment. Figure 45-4 illustrates the glottic angle in a two patients: one with a vocal fold paralyzed in the paramedian position and the other in a more lateral position.
Over the years, many explanations have been postulated for variation the appearance of the larynx in unilateral paralysis and the shift in position over time. The two theories with the most historical significance are Semon’s law and the Wagner-Grossman hypothesis. Semon postulated that in the progression of nerve injury, nerve fibers to the abductor muscles were affected first, so that the glottal opening would be impaired first.2 Later, adductor muscles would also become paralyzed, and the vocal fold would move laterally. However, Semon’s law actually predicts that the affected vocal fold would shift laterally over time. This is exactly opposite to the gradual adduction that has been so widely observed for many years. The Wagner-Grossman hypothesis asserts that after a recurrent laryngeal nerve injury, the vocal fold is adducted by the cricothyroid muscle, the only intrinsic laryngeal muscle that is not supplied by the recurrent laryngeal nerve. After a high lesion of the vagus nerve injury, both the recurrent and superior laryngeal nerve fibers are affected. Therefore, the Wagner-Grossman hypothesis predicts that without the adductor force of the cricothyroid, the vocal fold would lie in the cadaveric position.3 Because it is more consistent with clinical observations of vocal fold position in RLN and vagus nerve injuries, the Wagner-Grossman hypothesis has been widely accepted for many years.4 However, the flaws of the Wagner-Grossman hypothesis have gradually become apparent.
The preponderance of experimental evidence does not support the notion that the cricothyroid muscle adducts the vocal fold.5–9 This muscle does behave as an accessory muscle of inspiration, recruited by respiratory demand, and particularly by upper airway obstruction.6 However, blockade of the nerve to the cricothyroid muscle had no influence on glottic area or transglottic resistance, even during stimulated breathing, with maximal cricothyroid activity.7 An independent laboratory has confirmed these results.8 Simulated cricothyroid muscle contraction in cadaver larynges results in vocal fold lengthening, but no adduction.9
Many clinical series have failed to confirm a correlation between the position of the vocal fold and innervation of the cricothyroid muscle.10–12 Moreover, systematic studies of electromyographic activity in laryngeal muscles have determined that in the majority of patients with clinical laryngeal paralysis, laryngeal muscles are not completely denervated. In a study of 114 patients with a fixed vocal fold, Hirano found some EMG activity in 65%. He also noted that that vocal fold position was correlated with the number of motor units detected by EMG and not with the status of the cricothyroid muscle.10 In another study of patients with laryngeal paralysis, resulting from known sites of nerve injury, the position of the paralyzed vocal fold was quantified by measuring the angle formed by the vocal folds during prephonatory inspiration. Vocal fold position was correlated with EMG of the thyroarytenoid muscle.11 In patients with lesions of the recurrent laryngeal nerve, vocal fold position ranged from paramedian to cadaveric. All of these patients had some EMG activity in the ipsilateral thyroarytenoid muscle. But in each patient with a vagus nerve lesion, the position of the paralyzed vocal fold was cadaveric and the ipsilateral thyroarytenoid muscle was flaccid or atrophic (see Figure 45-2). Thus, the clinical observation of differences in vocal fold position can be correlated with the degree of reinnervation of laryngeal muscles, rather than activity of the cricothyroid muscle. A totally denervated hemilarynx is flaccid, with the vocal fold lying in a lateral position.13 EMG activity in a clinically paralyzed vocal fold can represent residual innervation after an incomplete original injury. It may also represent reinnervation of the muscles, either from a regenerated recurrent laryngeal nerve or by sprouting from other nerve sources.
Biology of Laryngeal Nerve Injury and Regeneration
Although injuries to other tissues in the body promote local cellular proliferation and mitosis, a peripheral nerve injury also provokes a complex biologic response that involves not only local tissues but also the central nervous system.14 Within hours after transection, Wallerian degeneration begins and ultimately involves the entire length of the nerve distal to the injury and a variable portion of the axon proximal to the injury. Both axons and surrounding myelin degenerate and are cleared away by proliferating Schwann cells and mast cells. The Schwann cells also begin to secrete neurotrophins. Nerve growth factor (NGF), brain-derived nerve growth factor (BDNF), and NT4/5 are produced in the distal nerve stump after axotomy, and they are then taken up by the proximal axon stumps to be transported to the cell body, where they promote neuron survival and axon regeneration.15 The neural tubes that surrounded the axons remain, but over time they contract in diameter.
With nerve transaction, each involved motor neuron initially undergoes chromatolysis. The nucleus migrates to the edge of the motor neuron, and Nissl granules break up and disperse. These changes herald the shift of the axon from neural transmission to repair. With time, the motor neuron returns to the center of the cell, Nissl bodies reorganize, and the neuron begins to synthesize proteins and lipids that will be transported to reconstruct the distal axon. Not all motoneurons survive after transaction of a nerve. In the spinal cord, 20% to 50% of motor neurons die after axonotmesis.16 Survival is inversely correlated with the distance of the lesion from the neuron, presumably because after a proximal injury, there is a long segment that must be regenerated. It has also been reported that cell death is more frequent after injury to a cranial nerve.
Peripheral nerves, including the RLN, have a strong propensity for regeneration and reinnervation of muscles. However, functional recovery is usually poor. A nerve is not like a simple power cord that can be “unplugged” and then “plugged back in.” Each nerve is a complex collection of axons that supply specific muscular targets. Axons are organized into fascicles, and if this structure is disrupted, regenerating axons can lose direction and reinnervate the wrong sites. This is termed synkinesis.17
The risk of synkinesis increases with the degree of nerve injury. Sunderland’s classification of the degree of nerve injury is based on histologic structure, recognizing the differential vulnerability of different tissues in the nerve.18 A first-degree injury is interruption of impulse conduction in an axon, without anatomic disruption. This can be caused by compression or perhaps ischemia. In a second-degree injury, the endoneurial tube remains intact but the axon loses continuity. Endoneurium is more elastic than the axon itself and therefore more resistant to disruption by traction. When the endoneurium remains intact, the endoneurial tubules guide regenerating axons back to their original targets. But in third-degree injury, the intrafascicular structure is disorganized. Thus, regenerating axons, though confined to the same fascicle, are no longer constrained to the original course within that fascicle and may reinnervate the wrong target (no longer being guided by an intact endoneurial tube as in second-degree injury). Further, scarring within the fascicle can block axonal regeneration. In fourth-degree injury, the fascicles themselves are disrupted so that there is even more disorganization of regenerating axons and a greater propensity for scarring that blocks nerve regeneration. In fifth-degree injury, the nerve trunk is completely transected so that there is no longer a conduit to guide regeneration.
Sunderland’s degrees of nerve injury are widely accepted. However, he also noted that nerve lesions may be partial or mixed, and this concept is less commonly discussed. And partial or mixed lesions may, in fact, be more common than injuries that uniformly affect all axons in a nerve. In a recent study, stretching, without visible disruption, was the most common mechanism of RLN injury during thyroidectomy.19 After a stretch injury, with an intact epineurium, it is not possible to determine whether individual axons or their perineuria are still intact. Thus, the injury from stretch could range from being a temporary neuropraxia to varying degrees of partial to total axonotmesis, with corresponding variation in the prognosis for recovery. Moreover, there may be considerable heterogeneity of the degree of injury among axons within a nerve. Finally, the branching pattern of the recurrent laryngeal nerve is variable and injury to individual branches results in different patterns of weakness.
Another source of variation in functional recovery is the location of the injury. During thyroidectomy, the most vulnerable location for injury is near the cricothyroid joint, under Berry’s ligament.19 Injury can also occur at any location along the recurrent or superior laryngeal nerve or their branches. The prognosis for recovery of motor function is better when the nerve lesion is more distal, closer to the target muscle.16 Recent evidence from animal research suggests that the prognosis for recovery after recurrent laryngeal nerve transection may be better after a right-sided rather than a left-sided injury.20
The strong propensity for regeneration of the recurrent laryngeal nerve is well documented in the literature. In spasmodic dysphonia patients with recurrent symptoms after RLN section, the nerve has been found to regenerate, even after removal of a large segment and ligation of the stumps.21 In animal studies, the RLN consistently regenerated after transaction, even across a several centimeter gap.22 The position of the affected vocal fold also shifted toward the midline. Electrical stimulation of the regenerated nerve produced laryngeal twitching, confirming that the regenerated nerve had reinnervated muscle; however, no appropriate motion was detected with respiration. After transaction of the regenerated nerve, the vocal fold position of the vocal fold shifted laterally, indicating that the net effect of the activity in the reinnervated muscle was to adduct the vocal fold.
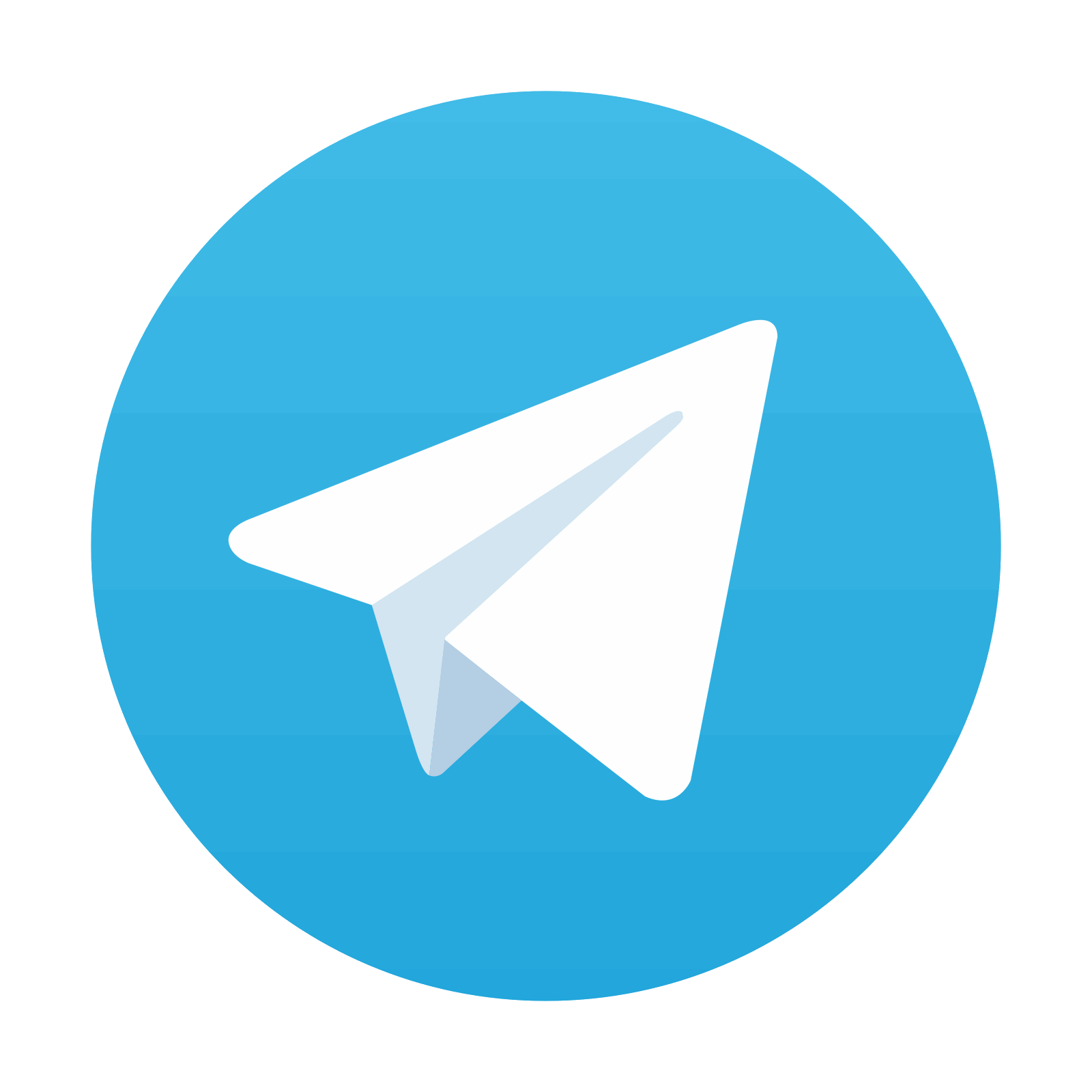