Fig. 8.1
Schematic representation of the sensory maculae and associated structures. Each hair cell (HC) is surrounded by supporting cells (SCs). Directly above the sensory epithelia, the otoconial membrane is composed of the subcupular meshwork, the gelatinous membrane, and the otoconia layer. Proteins that are revealed to influence the activity or structure of these layers are listed on the right
8.2 Otolith Formation
Proteins and genes relating to otolith and otoconium formation may be divided into four categories, as described below.
8.2.1 Formation of Normal Morphology
The formation of the otocyst and then the formation of sensory maculae are important steps in the development of otoliths and otoconia, as well as in the development of the normal morphology and function of the inner ear. Model animals in which Otx1, fgf3, Eya1, or Cdh11 genes have been deleted develop abnormal otoliths or otoconia [7–10].
8.2.2 Constituent Proteins
Otoconins are part of the inner core matrix composed of glycoproteins and proteoglycans [11]. Some otoconins are unique to the core of otoliths or otoconia. Otoconin 90 is the main core glycoprotein of mammals and birds [12, 13], and otoconin 22 is the main core glycoprotein of amphibians and reptiles [14]. Both otoconin 90 and otoconin 22 are homologous to secretory phospholipase A2 [13, 14]. There are other minor otoconins, such as osteopontin and calbindin-D28K. Osteopontin is a bone matrix protein that plays a role in the ossification of bone and teeth and in the formation of urinary stones and atherosclerotic plaques. In situ hybridization for the identification of osteopontin in the rat inner ear revealed osteopontin expression in sensory hair cells of the vestibular organs, suggesting its involvement in the maturation of otoconia [15]. Calbindin-D28K is a calcium-binding protein responsible for buffering the concentration of calcium ions [16]. In mammals, calbindin-D28K is expressed in the otolithic membrane of the sensory macula. Binding of calcium carbonate to calbindin-D28K is thought to be involved in the maturation process of otoliths and otoconia [16]. Otolin is a glycoprotein of less than 70 kDa expressed exclusively in the inner ear [17]. The C-terminus of otolin is structurally related to complement protein C1q and similarly enables the formation of oligomeric complexes. Zebra fish otolin morphants spontaneously develop decalcified and swollen otoliths. Otolin produced by supporting cells forms protein complexes with cerebellin-1 and otoconin 90. Alpha- and beta- tectorin have also been shown to be expressed in vestibular organs [18, 19]. Alpha-tectorin is strongly expressed in the peripheral area of the transitional zone and in the area where the accessory membrane originates in neonatal mice. Alpha-tectorin knockout mice display severe abnormalities in the extracellular matrix, leading to thinning of the gelatinous layer of the utricle and the saccule and agenesis or enlargement of otoconia in the saccule. In contrast, β-tectorin is expressed in the striola region. However, the contribution of β-tectorin to vestibular function is not well understood.
8.2.3 Regulatory Proteins
Plasma membrane calcium ATPase 2 (PMCA2) is the primary calcium ion pump of the endolymph, which serves to buffer the concentration of calcium ions. PMCA2 knockout mice have the agenesis of otoconia, despite normal sensory epithelium and gelatinous membrane [20]. The deletion of pendrin (Pds) leads to Pendred syndrome, an autosomal recessive hereditary disease characterized by deafness and thyroid goiter [21]. Pds knockout mice have large or no otoconia, in addition to other deformities including large vestibular aqueduct, dilated endolymphatic space, and degenerated stereocilia [22]. NADPH oxidase 3 (NOX3) forms compounds that will ultimately generate reactive oxygen species (ROS) in the inner ear. ROS are suggested to control the mitochondria and the endoplasmic reticulum, which are required to increase the concentration of calcium ions needed for mineralization of otoliths or otoconia [23]. Otopetrin-1 is a multi-transmembrane domain protein, the deletion of which results in severe balance disorder. Otopetrin-1 protein is localized in the granules secreted from supporting cells and in the otolithic membrane and the mutation of otopetrin-1 results in agenesis, deformation, misplacement of otoliths, and loss of a matrix [23].
8.2.4 Anchoring Proteins
Some proteins such as otogelin and otoancorin have been reported to provide instructions for the assembly and adhesion of matrix proteins at their right places in the inner ear. Otogelin is an N-glycosylated protein localized at the acellular membrane of the sensory patches such as the tectorial membrane in the cochlea, the gelatinous layer of the utricular and the saccular maculae, and the ampullary cupula, all of which affect mechanical transmission [24]. Otogelin knockout mice have both auditory and balance disorders. In the vestibule of otogelin knockout mice, the otoconial membrane is entirely detached from the sensory epithelium and found floating in the endolymphatic space. Otoancorin is an inner ear-specific protein, which is localized at the interface between the sensory epithelium and the gelatinous membrane [25]. In the vestibular systems, it is present on the apical surface of nonsensory cells beneath the otoconial membrane and cupulae. Otoancorin is thought to bind the gelatinous matrix to the underlying epithelial patches [25].
8.3 Factors Affecting the Structure of Otoliths
8.3.1 Drugs
The administration of streptomycin causes a decrease in the number of otoconia and changes the morphology of sensory cilia, which begins from the striola [26]. Moreover, streptomycin induces dark cells to absorb otoconia and to lower the calcium content of otoconia. The loss of otoconia was more prominently observed in the saccule, where no dark cells exist, suggesting a role for dark cells in maintaining the calcium concentration in otoconia. A previous study using guinea pigs showed that otoconia regeneration started at 6 weeks and was normalized at 10 weeks after administration of streptomycin [27].
8.3.2 Aging
Otoconia of the saccule start to degenerate in humans in the 6th decade of life [28]. It is known that otoconia of the saccule degenerate more remarkably than otoconia of the utricle. Low estrogen levels frequently detected in mid-aged and elderly women have been recognized to cause decalcification of otoconia, which in turn alters the electrolyte composition and pH value of both endolymph and perilymph. BPPV patients older than 50 years also present osteopenia or osteoporosis in 75 % of the cases [29]. Thus, altered hormone levels are suggested to induce vulnerability of otoliths and surrounding tissues.
8.3.3 Hypergravity
Anken et al. revealed that long-term exposure to 3 g hypergravity conditions causes a reduction in size and an increase in asymmetry of otoliths of cichlid fish compared to normal earth gravity controls [30]. Some of the animals subjected to hypergravity showed kinetotic behavioral abnormalities, such as spinning movements. Sondag et al. exposed golden hamsters to 2.5 g hypergravity conditions from uterine conception until 1–4 months after birth [31]. Hypergravity led to alteration of size distribution of otoliths, which persisted even after 8 months of exposure to normal gravity forces.
8.4 Otoliths and Semicircular Canals
Otoconia-deficient mutant mice are unable to swim and show impaired balance when challenged, but no disorientation [32]. “Head tilt” mutant mouse, which arose spontaneously at The Jackson Laboratory, have no phenotypic abnormality other than loss of otoconia [33]. Canal-only rotation stimuli produced attenuated vestibulo-ocular reflex responses in these “head tilt” mice. This suggests the interdependence of otoconia and semicircular canals and indicates the hitherto unrecognized importance of otoconia.
References
1.
Wersall J, Engstrom H, Hjorth S. Fine structure of the guinea-pig macula utriculi; a preliminary report. Acta Oto-Laryngol Suppl. 1954;116:298–303.CrossRef
2.
Schessel DA, Ginzberg R, Highstein SM. Morphophysiology of synaptic transmission between type I hair cells and vestibular primary afferents. An intracellular study employing horseradish peroxidase in the lizard, Calotes versicolor. Brain Res. 1991;544(1):1–16.PubMedCrossRef
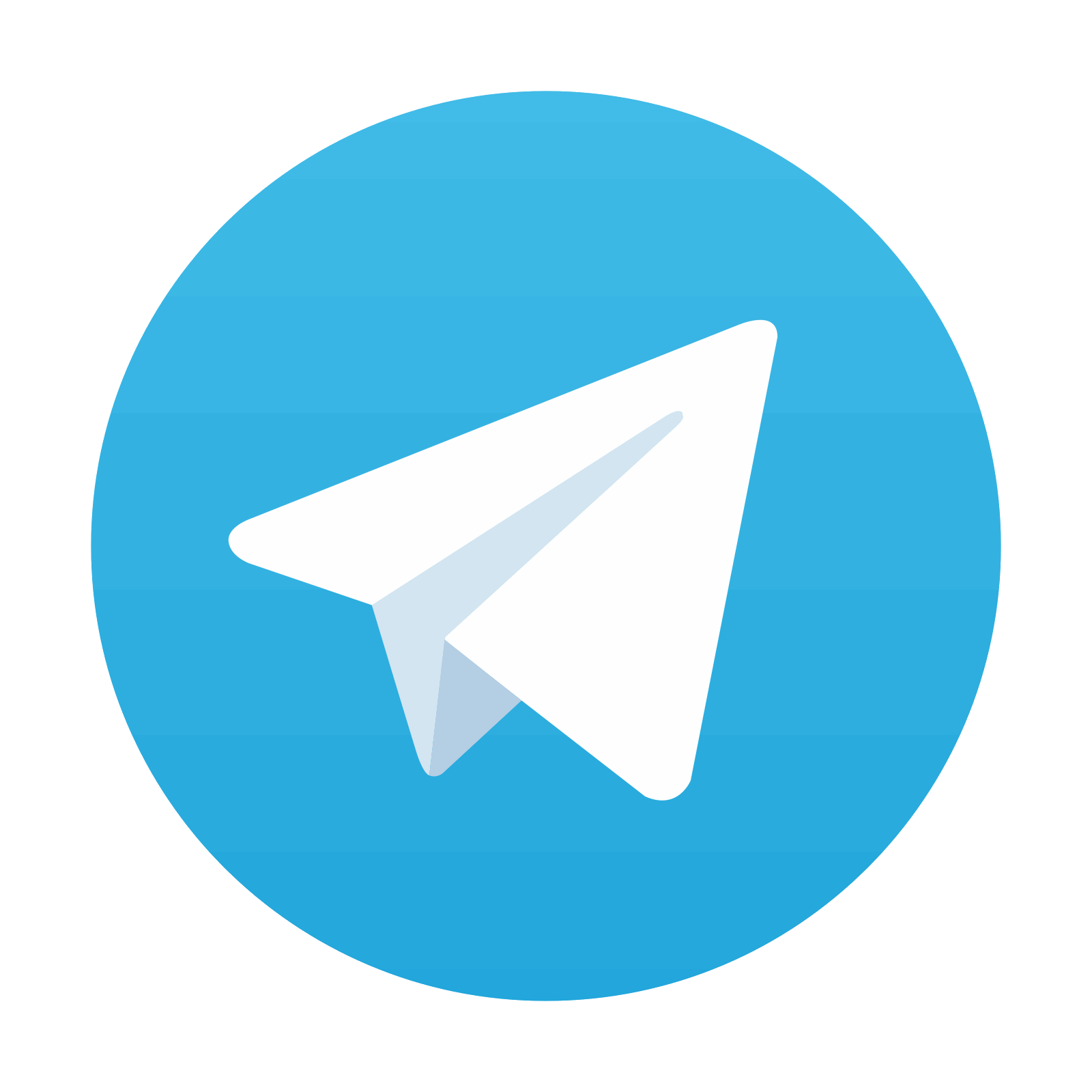
Stay updated, free articles. Join our Telegram channel

Full access? Get Clinical Tree
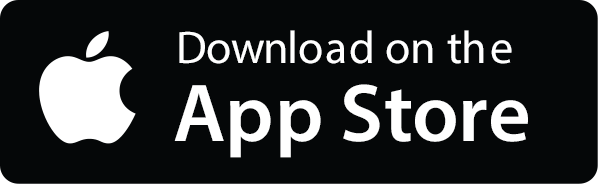
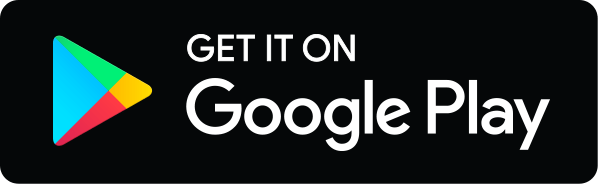