Fig. 11.1
Early inner ear developmental steps from the inner cell mass to the otic vesicle with morphogens and marker genes. ICM inner cell mass, RA retinoic acid
11.2 Formation of Epiblast and the Three Germ Layers
A fertilized egg divides several times and generates a trophoblast and an inner cell mass. The inner cell mass gives rise to the primitive endoderm (or hypoblast), which becomes the yolk sac, and the epiblast, which produces the embryonic tissues. In the epiblast, cells that delaminate from the primitive streak in the midline of epiblast give rise to the mesoderm and the endoderm. The remaining epiblast becomes the ectoderm.
For the in vitro differentiation of pluripotent stem cells, these processes are partly recapitulated by the formation of embryoid bodies (EBs). An EB is a pluripotent cell aggregate that is formed to induce stem cells to differentiate [9]. Generally, EBs are cultured in suspension or in nonadhesive culture dishes and are capable of forming resemblances of the three germ layers. EBs are also utilized for otic induction methods. Oshima et al. cultured EBs in medium supplemented with Dkk1 (a Wnt inhibitor) and SIS3 (a Smad3 inhibitor that inhibits TGF-β1-dependent Smad3 phosphorylation) to suppress mesoderm formation [2]. Koehler et al. also used EBs under conditions designed for the preferential development of ectoderm [4].
11.3 Differentiation into Neural and Nonneural Ectoderm
After three germ layers (ectoderm, mesoderm, and endoderm) are formed, the ectoderm differentiates into neural and nonneural ectoderm depending on the bone morphogenic protein (BMP) gradient [10, 11]. In the embryo, the ectoderm is a source of BMP, and the notochord, located in the midline of the mesoderm, is a source of BMP antagonists such as noggin, chordin, and follistatin [12]. The lateral part of the ectoderm that receives a high level of BMP signal gives rise to nonneural ectoderm, and the medial part of the ectoderm in which the BMP signal is suppressed by the antagonists gives rise to the neural ectoderm.
The membranous labyrinth of the inner ear is a derivative of nonneural ectoderm. BMP-4 supplementation has been used in various studies to induce nonneural ectoderm.
11.4 Induction of Preplacodal Ectoderm
In the head region, a morphologically indistinguishable horseshoe-shaped area of surface ectoderm next to the neuroectoderm called the preplacodal ectoderm (PPE) becomes competent to respond to placode-inducing signals at late gastrula or early neural plate stages [11]. The PPE and later on the placodes give rise to the lens, ear, olfactory epithelium of the nose, and trigeminal and epibranchial ganglia [11]. The PPE is characterized by the expression of Six1, Six4, and Eya2 [13–16].
BMP signaling from the lateral ectoderm, Wnt signaling from the trunk ectoderm and mesoderm, FGF signaling from the mesoderm, and Wnt/BMP antagonists from the neural plate act in concert to form the neural crest and the PPE [13]. Although BMP signaling is required for the induction of nonneural ectoderm, subsequent BMP inhibition in conjunction with active FGF signaling is required for the formation of the PPE [13, 17–19]. Most of the studies concerning the induction of PPE have been conducted on Xenopus and fish rather than birds and mammals.
In addition to BMP, Wnt, and FGF signals, Dlx3 and Gata2 are also essential for the induction of the PPE, at least in Xenopus [17]. In addition, Six1 is sufficient to induce PPE at the expense of the neural crest and epidermis in Xenopus [20]. Furthermore, BMP levels need to be altered for PPE formation to take place [18].
The complicated process of PPE determination requires further investigation and is difficult to apply in the otic induction of pluripotent stem cells. The use of BMP-4 and SB431542 (a TGF-β inhibitor) followed by LDN-193189 (a BMP inhibitor) and bFGF in Koeheler’s study may partly mimic this step [4].
11.5 Induction of the Otic Placode
The earliest visible primordium of the inner ear is the otic (or epibranchial) placode, which is visible as a thickening in the PPE. The otic placode becomes visible at 8.0–8.5 dpc in mice and at the 8/9 somite stage in chicks. Pax2 is a widely used marker for the otic placode. In chicks, Soho1 and Nkx5.1 are also typically used [21, 22].
In the PPE, mesodermal FGF (more specifically Fgf3 and Fgf19 in chicks) determines the Pax2-positive otic competent region, called the otic–epibranchial progenitor domain, followed by Wnt (Wnt8c in chicks) and the attenuation of FGF, which further specifies the development of the otic placode over the epibranchial placode [21, 22]. In mice, neural Fgf3 and mesodermal Fgf10 are required for the induction of otic placodes [23, 24].
A recent study showed that Pax2 regulates proliferation rather than cell specification in the otic–epibranchial progenitor domain [25]. In mouse, Pax8 is also an otic–epibranchial progenitor domain and otic placodal marker [26].
As in the embryo, FGFs, Wnt, and Pax2/8 are decisive factors for the formation of the otic placode from stem cells. For otic induction from pluripotent stem cells, bFgf as a universal Fgf or Fgf3/Fgf10 as more inner ear-specific FGFs are used. As Koehler et al. report, intrinsic Wnt in the cell aggregates in their method appears to function as an inducer for the otic fate [1]. The role of bFGF in the method developed by Oshima et al. may be attributed to the importance of FGF signaling in this step [2].
11.6 Induction of the Otic Vesicle and Axis Formation
The otic placode invaginates to form the otic pit or cup and pinches off from the surface ectoderm to form the otic vesicle. The otic vesicle further changes its morphology to form multiple inner ear substructures, including the semicircular canals, vestibule (the utricle and saccule), and cochlea. The process of the invagination and pinch-off to form the otic vesicle requires FGF, which was demonstrated by coculture of chick otic placode with FGF-soaked heparin beads [27].
During and after its formation, the otic vesicle is placed in the three-dimensional concentration gradient of various molecules, where it differentiates into several structures and cell lineages such as the endolymphatic sac, the membranous part of the cochlea, the vestibules, the semicircular canals, and the sensory areas and neurons of each substructure. The compartmentalization of the otic vesicle into these substructures is determined by three axes: the anterior–posterior axis, the dorsal–ventral axis, and the medial–lateral axis. Sensory organs and neurons are derived from the anterior region of the otic cup, and the posterior crista is derived from the posterior region. The dorsal region differentiates into the endolymphatic duct, semicircular canals, and utricle, and the ventral region differentiates into the saccule and cochlea. Neurons and most of the sensory epithelium are induced from the anterior region [28] (Fig. 11.2).
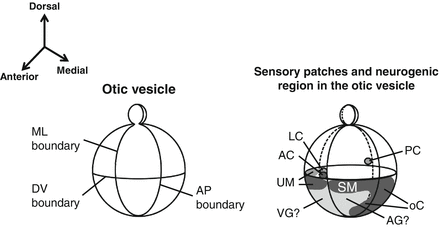
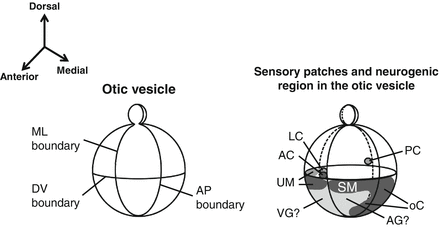
Fig. 11.2
The three axes in the otic vesicle and a possible distribution map of sensory organ primordia and neuroblasts. (Modified from Fekete and Wu [28].) AC anterior crista, AG auditory ganglia, AP anterior–posterior, DV dorsal–ventral, LC lateral crista, ML medial–lateral, oC organ of Corti, PC posterior crista, SM saccular macula, UM utricular macula, VG vestibular ganglia
11.6.1 Anterior–Posterior Axis
Retinoic acid (RA) is a morphogen that acts in anterior–posterior axis formation. One study using RA-soaked beads showed that cells in the anterior otic cup that were exposed briefly to RA gave rise to neurons and most of the sensory organs of the inner ear, whereas prolonged and higher level exposure of RA in the posterior otic cup promoted the formation of non-sensory structures [29]. In mouse, Tbx1 expression is localized in the posterior half of the otocyst [30]. In the Tbx1-null mutant mouse, expression of NeuroD1, Lfng, and Fgf3 disappeared from their normal expression domains in the anterior region of the otocyst and instead expanded into the posterior region; in addition, the expression of Otx1 in the posterior region of the otocyst disappeared [31]. These data suggest that Tbx1 is also involved in anterior–posterior axis formation.
11.6.2 Dorsal–Ventral Axis
Wnts secreted from the dorsal hindbrain and Sonic hedgehog (Shh) secreted from the ventral floor plate and notochord are implicated in the patterning of the inner ear dorsal–ventral axis. Lithium chloride, a Wnt canonical pathway agonist, is able to restore, in part, otic vesicle gene expression patterns altered by ablation of the dorsal neural tube in otic explants [32]. Wnt1/Wnt3a double-knockout mice show defects in the dorsal region and in part of the ventral region of the inner ear [32]. The otic vesicles of β-catenin conditional knockout mice show severe shrinkage. This result indicates that Wnt signaling is required for otic vesicle formation [33]. The Wnt signal acts via the regulation of Tbx1, Eya1, and Six1 [33].
One of the major downstream factors of Shh is the zinc-finger transcription factor Gli3. Based on the results of several studies on mice and chicks, Shh generates opposing gradients of Gli3 repressor and Gli2 and Gli3 activators in the otic epithelium along the dorsal–ventral axis [34]. One study using the Shh conditional knockout mouse showed that the absence of Shh results in the disappearance of Pax2, Otx2, and Gata3 and a morphological defect in the cochlea [35].
11.6.3 Medial–Lateral Axis
After the otic cup closes, the dorsal–medial region of the otic vesicle extends to form the endolymphatic duct. In contrast, the dorsal–lateral wall of the otic vesicle mainly gives rise to the vertical and horizontal pouches that form the three semicircular canals. The molecular mechanisms underlying the formation of the medial–lateral axis of the otic vesicle are not well understood.
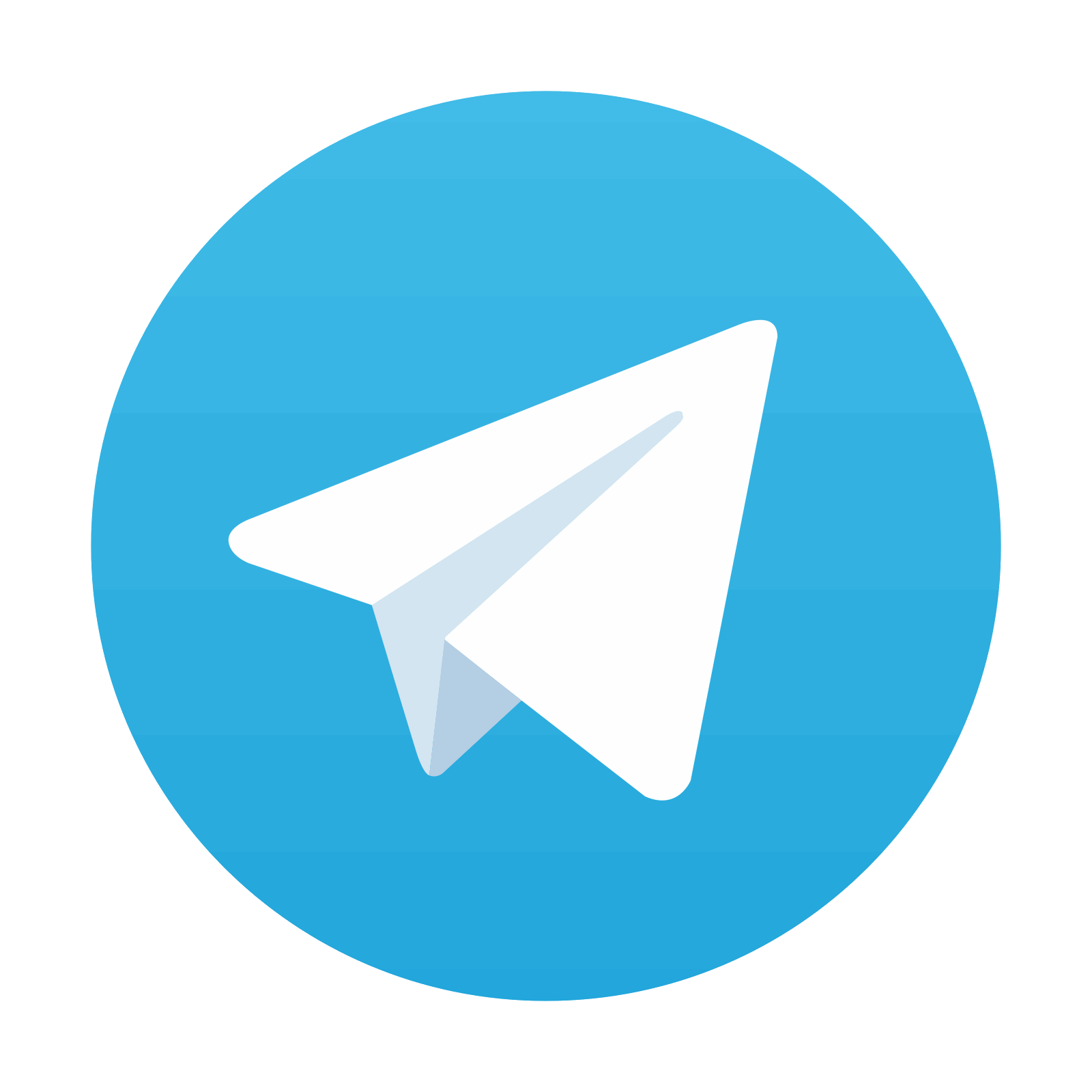
Stay updated, free articles. Join our Telegram channel

Full access? Get Clinical Tree
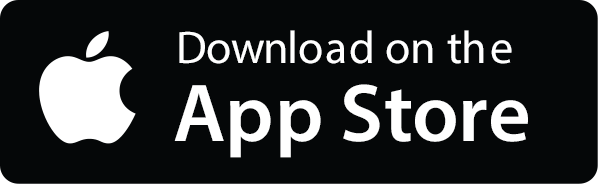
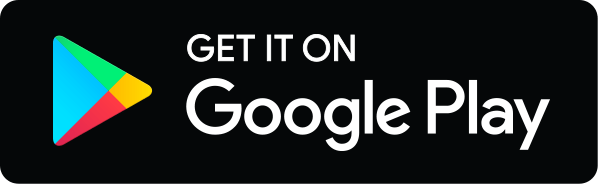