Fig. 4.1
Backscatter in Fuchs endothelial dystrophy (FED) corneas. (a) Confocal microscopy measures brightness of illumination at different depths of the cornea. The optical axis of the illuminator and detector are at equal angles to the normal, resulting in the detection of specular reflection (dominant) and backscatter [48]. (b) Corneal backscatter profiles generated from confocal images with the depth of the cornea scaled from 0 % at the anterior stromal boundary to 100 % at the endothelium [5]. Backscatter was standardized and measured in scatter units (SU), the concentration of a turbidity standard that produces the same image brightness. Profile of backscatter through the normal cornea and corneas with mild, moderate, and advanced FED show increasing anterior corneal backscatter with increasing severity of FED (left) [5]. After endothelial keratoplasty, anterior corneal backscatter that was high preoperatively in advanced FED corneas improved through 5 years, but did not return to normal (right) [83]

Fig. 4.2
Confocal microscopy of Fuchs endothelial dystrophy (FED) corneas. Representative confocal images of the anterior corneal stroma and the associated endothelium in a normal cornea, in mild, moderate, and advanced FED, and at 5 years after Descemet stripping endothelial keratoplasty (DSEK) for advanced FED. There is increased matrix reflectivity (backscatter) in FED associated with depletion of keratocyte nuclei. These changes persist after endothelial keratoplasty
Of importance, keratocyte depletion and increased backscatter from the anterior cornea can be detected in the early stages of FED, before guttae become confluent and before edema is clinically detectable (Fig. 4.2) [5, 85]. These early anterior anatomic changes raise questions as to whether or not the primary dysfunction in FED is solely in corneal endothelial cells. They also indicate that FED is a chronic disease that has subclinical and clinical phases. After endothelial keratoplasty, keratocyte depletion persists through at least 3 years [56], and although anterior corneal backscatter improves, it does not return to normal through 5 years (Fig. 4.2) [8, 83]. Early improvement in backscatter can be attributed to resolution of corneal edema, but late persistence of elevated backscatter suggests alterations of corneal architecture in response to chronic edema before keratoplasty.
4.2.1.3 Corneal Nerves and Sensation
The nasociliary ophthalmic branch of the trigeminal nerve densely innervates the cornea to provide sensation and neurotrophic factors to the epithelium and keratocytes [3, 91]. In FED, subbasal and stromal nerves visible by confocal microscopy are tortuous, form loops, and are decreased in number early in the course of disease (Fig. 4.3) [3, 58, 66]. These changes are associated with decreased corneal sensation, even in corneas without clinically obvious anterior disease [3]; although Fuchs described decreased sensation in his original report, his patients had advanced FED with obvious anterior pathology [24]. Corneal sensitivity remains lower than normal even 2 years after endothelial keratoplasty, an indication of slow or limited repair after restoring endothelial function [3].


Fig. 4.3
Corneal nerves in Fuchs endothelial dystrophy (FED). Confocal images of subbasal and stromal nerve fiber bundles in a normal cornea and before and after Descemet stripping endothelial keratoplasty (DSEK) for FED. Subbasal nerve fiber bundles appear as linear bright structures between the basal epithelial cells and anterior keratocytes in normal corneas. In FED, when the subbasal nerves are visible, they typically appear finer and sparser than normal. After DSEK for FED, subbasal nerve fiber bundles typically remain fine and sparse and, occasionally, have thickened and abnormal branching patterns. Stromal nerves in normal corneas typically appear as straight brightly reflective structures with well-defined branching points. In FED, stromal nerves are often tortuous with loops and are often associated with brightly reflective keratocyte nuclei. After DSEK, the tortuosity persists, and the nerves typically appear finer than before keratoplasty, though they are still often associated with brightly reflective keratocyte nuclei
The role of corneal nerves in FED is unclear, but it is likely that they help maintain the integrity and regularity of the anterior cornea [51]. Alterations of nerve density and function might be a response to changes in the microenvironment, created by chronic edema, with reduced autocrine production of vasoactive intestinal peptide and other protective neuropeptides by the altered corneal endothelium [20]. In turn, reduced production of neurotrophic factors might affect endothelial cells and keratocytes by disinhibiting apoptosis [20]. This condition would suggest that the nerves indirectly change the anterior corneal ultrastructure, and this would affect the regularity and integrity of the anterior surface and vision [5, 85].
4.2.2 Posterior Cornea
The definitive association of posterior corneal changes with FED came after the slit lamp was introduced in 1911 by Gullstrand when Koeppe noted dimples on the endothelial surface [37]. These dimples were later termed cornea guttata by Vogt [82], and they remain the defining diagnostic feature of FED. Although traditionally there has been a distinction between cornea guttata and FED, these labels represent a spectrum of the disease and will probably be replaced by genetic definitions in the future.
4.2.2.1 Descemet Membrane
In normal corneas, Descemet membrane, the basement membrane of the endothelial cells, becomes thicker throughout life [35]. This structure has two distinct layers, a uniform anterior banded layer approximately 3 μm thick and a posterior non-banded layer that thickens from 2 μm at age 10 years to approximately 10 μm at age 80 years. In FED, Descemet membrane is thicker than normal and has an irregular contour, and the non-banded layer becomes non-uniform [35, 43]. The change in the collagen banding pattern is assumed to indicate when abnormal endothelial cell function begins. The presence of a thinner than normal non-banded posterior layer in FED suggests that endothelial dysfunction begins early in the disease; abnormal posterior banded layers then develop and account for most of the increased thickness of the membrane, and focal collagenous excrescences develop as guttae [12, 34, 92]. Guttae tend to be distributed more horizontally than vertically [1] and more inferiorly [25, 59], often appearing in the interpalpebral zone. Disease severity is usually graded based on the presence and confluence of guttae as well as the presence of clinical edema (Fig. 4.4) [40, 44].


Fig. 4.4
Clinical grading of Fuchs endothelial dystrophy (FED). By using simple slit lamp retro-illumination and specular reflection, the endothelium can readily be graded in clinical practice and research settings. A modification of Krachmer’s grading system is commonly used and is based on the area and confluence of guttae and the presence of edema [40, 44]. Grades 1 and 2 are corneas with 1–12 or more than 12 non-confluent central guttae, respectively (which we considered to have mild FED); grades 3 and 4 are corneas with confluent guttae of 1–2 mm or 2–5 mm in widest diameter, respectively (which we have considered to have moderate FED); grades 5 and 6 are corneas with >5-mm diameter of confluent guttae or any visible stromal or epithelial edema, respectively (which we have considered to have advanced FED)
Clinically, the Descemet membrane can appear gray and irregular in FED and is best appreciated with broad tangential illumination. With clinical confocal microscopy, Descemet membrane is not visible in normal young subjects, but occasionally becomes visible with increasing age [53]. In FED, guttae are easily visible against the endothelial cell mosaic and, when confluent, appear as voluminous ridges on the posterior corneal surface (Fig. 4.2) [56]. Abnormalities corresponding to the location of Descemet membrane in FED have been described, including a honeycombed appearance and linear structures in oblique sections, although their significance is unknown [56].
4.2.2.2 Endothelial Cells
Corneal endothelial cells form a barrier to solutes and actively transport water and electrolytes [42, 45], and this active pump keeps the normal corneal stroma dehydrated [60]. Endothelial dysfunction is thought to be the primary pathology in FED and is manifested as decreased endothelial cell density or decreased function, or both. Chronic endothelial cell dysfunction results in progressive corneal edema [14, 61], which disrupts the corneal microenvironment [15], and this could affect anterior structures. Recent evidence of anterior corneal changes early in the disease suggests that early endothelial dysfunction may also be responsible for chronic edema, assuming that the anterior corneal changes are not a primary abnormality [5, 85].
In FED, endothelial cells are disrupted by the guttae, which are often lined with a thin layer of cytoplasm, although continuity of the endothelial cell monolayer and the barrier function of the endothelium appear to remain intact until advanced stages of FED. Endothelial cells are lost, presumably by apoptosis; penetrating keratoplasty buttons express DNA fragmentation by TUNEL (terminal deoxynucleotidyl transferase-mediated dUTP nick end labeling) [9]. However, transmission electron microscopy has found little evidence of apoptosis, possibly because of cell decomposition or sampling error [34]. Endothelial cells do not reproduce significantly after trauma or in response to disease, and the endothelium is repaired by cell migration, enlargement, and redistribution [18, 81].
The endothelium in FED can be assessed macroscopically by evaluating the distribution of guttae clinically or in retro-illumination slit lamp photographs (Fig. 4.4) [26, 63]. The corneal endothelium can also be qualitatively examined by specular reflection at the slit lamp or quantitatively examined by specular or confocal microscopy. The hexagonal array of cells in normal endothelia is disrupted by guttae in FED, and these appear dark in confocal and specular images relative to the light cells. In FED, endothelial cells express polymegethism (variable cell size) and pleomorphism (variable cell shape) [10], but their density cannot be determined in the same way as in normal corneas or corneas after keratoplasty because the area of guttae and regional density variation would produce sampling errors [25, 46]. When considering the distribution of guttae, modified methods must be used to assess endothelial cell density. The effective endothelial cell density (ECDe; Fig. 4.5) is an objective measure that estimates the overall cell density from the density of contiguous cells and the fraction of the image covered by guttae (Figs. 4.2 and 4.5). It correlates well with subjective grading of FED [46].


Fig. 4.5
Method of assessing endothelial cell density in Fuchs endothelial dystrophy (FED). Local endothelial cell density measurement in FED by fixed frame, variable frame, or flex center methods results in variable and imprecise estimation of cell density because of regional variation and the presence of guttae (left). To calculate the effective endothelial cell density, local cell density in several areas of contiguous cells that do not include guttae is multiplied by the ratio of the image area that is free of guttae (Rguttae-free) [46]
4.2.3 Corneal Thickness and Curvature
Central corneal thickness has been a simple and helpful measurement in clinical practice to assess corneas with edema. Corneas thicker than 640 μm are usually abnormal, and this thickness was suggested to be an indicator for combined keratoplasty and cataract extraction in the penetrating keratoplasty era [69]. Determining the presence of corneal edema when corneas are thinner than 640 μm can be a challenge when the cornea is not obviously swollen and particularly when the non-edematous (or historical) thickness is unknown. Recent evidence shows that central corneal thickness increases gradually with severity of FED, and corneas are thicker than normal even in mild disease [39, 63]. This is further evidence that corneal changes early in the disease might be the result of chronic edema.
Improvements in Scheimpflug cameras and anterior segment optical coherence tomography (AS-OCT) have enhanced our understanding of corneal curvature of the anterior and posterior surfaces, and the distribution of thickness in normal and abnormal eyes. Normal corneas are thicker vertically than horizontally, which explains against-the-rule astigmatism induced from the posterior corneal surface [74, 86]. In FED, this profile becomes abnormal with bulging of the posterior corneal surface into the anterior chamber because of central edema [13, 41]. Corneal edema in FED changes the normal ellipsoid posterior profile to a more spherical shape, effectively inducing less negative power and loss of normal posterior surface toricity [86]. Similarly, the steep anterior and posterior surface meridians, which are usually oriented vertically in normal corneas [36], are more often oblique or horizontal in FED [86]. These changes may partially explain the hyperopic shift after endothelial keratoplasty and might contribute to inaccurate refractive outcomes after cataract surgery [86].
4.3 Optical Changes
The optical quality of vision can be described in terms of the retinal point spread function, which is the image on the retina of an infinitely small point at infinity [75]. The point spread function normally has a high and narrow central peak (within the central 1° and termed small-angle domain) that decreases to a low and broad peripheral flange (beyond 1° and termed large-angle domain; Fig. 4.6) [75]. Visual acuity is determined by the shape at the center of the point spread function; the sharper the peak, the better the acuity. Abnormalities in the optical media can widen and flatten the central peak and elevate the flange. The center of the point spread function is usually degraded by optical aberrations. Low-order aberrations can be neutralized by spherocylindrical correction, and thus high-order aberrations (HOAs, traditionally thought of as irregular astigmatism) are the primary cause of optical degradation that affects visual acuity. Disability glare corresponds to the large-angle domain of the point spread function and is increased by forward light scatter (retinal straylight) that degrades the contrast of the image [75]. In eyes with spherical intraocular lens implants, changes in the shape of the point spread function can be explained by corneal pathology, and thus in pseudophakic FED patients, optical properties of the cornea can be determined from quality of vision assessed by measuring visual acuity and retinal straylight.


Fig. 4.6
Point spread function. The normal point spread function has a high and narrow central peak within the central 1° (small-angle domain) and a low and broad peripheral flange beyond 1° (large-angle domain). Visual acuity is determined by the shape of the center of the point spread function, which is degraded by high-order aberrations. Disability glare corresponds to the periphery of the point spread function, which is degraded by forward scatter (or straylight). The gray line is the point spread function under low forward scatter conditions; the black line is the point spread function under high forward scatter conditions. This shows that forward scatter has minimal impact on the center of the point spread function and therefore on visual acuity (Reproduced from Fig. 4.5 in McLaren et al. [47])
4.3.1 Small-Angle Domain and High-Order Aberrations
Aberrations originate from local changes in the regularity of a surface or changes in refractive index that refract and redirect a ray of light. In pseudophakic eyes with spherical intraocular lenses, high-order aberrations predominantly arise from the anterior and posterior corneal surfaces and possibly from any lamellar interface (assuming there is a change in refractive index at the interface). Aberrations create wavefront errors that can be detected by Hartman-Shack aberrometry for the whole eye. Corneal surface irregularities can be isolated and measured from elevation maps derived from videokeratoscopy or corneal tomography to generate surface-derived wavefront errors. Wavefront errors are expressed as Zernike polynomials across the optical aperture, and their amplitude is typically expressed as the root-mean-square of the total wavefront error.
Visual acuity is degraded by increased HOAs, especially those at the center of the Zernike pyramid [6]. Not all aberrations affect visual acuity equally, and different combinations of aberrations can result in variable effects on visual acuity (Fig. 4.7) [6, 47]. HOAs originating from the anterior corneal surface can be corrected with rigid contact lenses, and this was an important method of visual rehabilitation in the era of penetrating keratoplasty and still is for anterior corneal irregularity.


Fig. 4.7
Effect of aberrations on visual acuity. Whole eye wavefront errors were measured in pseudophakic eyes after Descemet stripping endothelial keratoplasty (DSEK) by Hartmann-Shack aberrometry and compared to otherwise normal pseudophakic eyes implanted with the same type of intraocular lens [47, 68]. These figures simulate the image of an eye chart on the retina in a patient with the high-order aberrations (HOA) noted. Wavefront errors were increased after DSEK compared to normal pseudophakia. The right column shows simulated eye charts in the patients with median high-order aberrations and with the next higher and next lower high-order aberrations in a study [47]. Note that similar magnitudes of wavefront error that include different combinations of aberrations can have qualitatively different effects on visual acuity because not all aberrations are equal [6] (Reproduced from Fig. 4.6 in McLaren and Patel [47]
Understanding the role of HOAs in FED began with trying to understand incomplete visual rehabilitation after endothelial keratoplasty, for which the most common indication is FED. Few eyes achieved best-corrected Snellen acuity of 20/20 after deep lamellar endothelial keratoplasty (DLEK) [70, 71], and this was attributed to the donor-host lamellar interface. In DLEK, the donor and host stroma were both manually dissected. Transitioning to Descemet stripping endothelial keratoplasty (DSEK), which did not require host stromal dissection and was accompanied by microkeratome preparation of the donor, resulted in improved visual acuity outcomes, and this was attributed to a smoother lamellar interface. Because the anterior corneal surface was not surgically incised and sutures were not used, in contrast to penetrating keratoplasty, it seemed unlikely that HOAs could be a limiting factor for visual acuity after endothelial keratoplasty. This stimulated interest in assessing light scattered by the cornea as a cause of decreased visual acuity after endothelial keratoplasty (see below), especially because some eyes manifested clinical haze at the interface.
In a study that assessed quality of vision and after penetrating and endothelial keratoplasty, the center of the point spread function was significantly and similarly degraded after both procedures [67], suggesting HOAs were indeed elevated after DSEK. This was confirmed by whole eye wavefront aberrometry [68] and studies that examined both corneal surfaces after DSEK (Fig. 4.7) [38, 50, 55, 65, 88]. Apposition of irregular stromal surfaces at the lamellar interface can transmit that irregularity and the consequential aberrations to the posterior corneal surface after DLEK and to a lesser extent after DSEK. In DMEK, the posterior corneal surface should essentially be that of the posterior host stromal surface that is not disrupted during the procedure, and better visual outcomes after DMEK as compared to those after DSEK have been attributed to decreased posterior HOAs [65].
While posterior corneal HOAs are a likely explanation for decreased visual acuity after endothelial keratoplasty, decreased acuity has not been correlated with HOAs from the posterior corneal surface [65, 89] but has been correlated with HOAs from the anterior corneal surface after DSEK and DMEK [38, 55, 65, 89]. Posterior corneal HOAs are of lower magnitude than anterior corneal HOAs because of the smaller change in refractive index at the posterior corneal surface compared to anterior, and it is therefore not surprising that anterior corneal HOAs have a greater effect on visual acuity. More important might be the concept of “parallelism” (Fig. 4.8): posterior corneal HOAs reduce those from the anterior surface in corneas with “parallel” surfaces (normal corneas and penetrating grafts), but when surface “parallelism” is disrupted, such as after DLEK and DSEK (and deep anterior lamellar keratoplasty), posterior HOAs might become additive to anterior HOAs and further decrease acuity [90].


Fig. 4.8
Corneal surface “parallelism.” Representative color maps of anterior and posterior corneal high-order wavefront aberrations in a normal cornea, a cornea after penetrating keratoplasty (PK), and a cornea after Descemet stripping endothelial keratoplasty (DSEK). In normal eyes and eyes after PK, color maps of the anterior surfaces show a reverse pattern with respect to those of the posterior surfaces, indicating that the two surfaces have similar shapes and that posterior aberrations normally counteract anterior aberrations (recognizing that the magnitude of aberrations is much lower from posterior than anterior). After DSEK, the same color reversal pattern is not evident indicating different shapes of the anterior and posterior surfaces and possibly resulting in more total irregularity [90]. Color map scales are not given because they are different for each example to enable illustration of the degree of parallelism in each situation (e.g., the color map scale for the normal eye has been exaggerated relative to the PK eye because the overall magnitude of wavefront error is smaller)
DMEK has gained popularity because of improved visual acuity outcomes compared to DSEK. While the majority of eyes have excellent visual acuity as early as 6 months after DMEK [27, 62, 64, 79], not all of them can be corrected to 20/20 [17]. Even though one would assume that the anterior and posterior corneal surfaces resume a “parallel” relationship after DMEK, the anterior corneal surface can itself be irregular and cause decreased visual acuity [55, 78]. Applying rigid contact lenses has helped improve visual acuity after DMEK, confirming this concept [80].
The irregularity of the anterior corneal surface after endothelial keratoplasty, which does not incise or suture the anterior corneal surface, was unexpected. This suggested that the underlying disease process in eyes with FED might affect the anterior surface [55, 83]. Indeed, anterior corneal aberrations are higher than normal in advanced FED, which is not unexpected in advanced disease because of epithelial or stromal edema [55]. However, even after resolution of corneal edema and at 5 years after endothelial keratoplasty, anterior corneal HOAs remained higher than normal, suggesting that the changes chronically preceded the intervention [55, 85]. Evaluation of a range of severity of FED corneas confirmed that anterior corneal HOAs are increased early in the course of disease before the epithelial surface is clinically disrupted [85], supporting the hypothesis that a chronic state of subclinical edema changes corneal structure in FED.
Posterior corneal HOAs are also increased in the early stages of FED compared to normal [85], and this is likely explained by an irregular surface from endothelial guttae. Of interest is that posterior corneal HOAs at 6 months after DMEK [65] are similar to those in FED prior to keratoplasty and higher than normal [85]. This raises the possibility of chronic posterior stromal changes in FED, although long-term follow-up of posterior HOAs after DMEK is required to characterize this better.
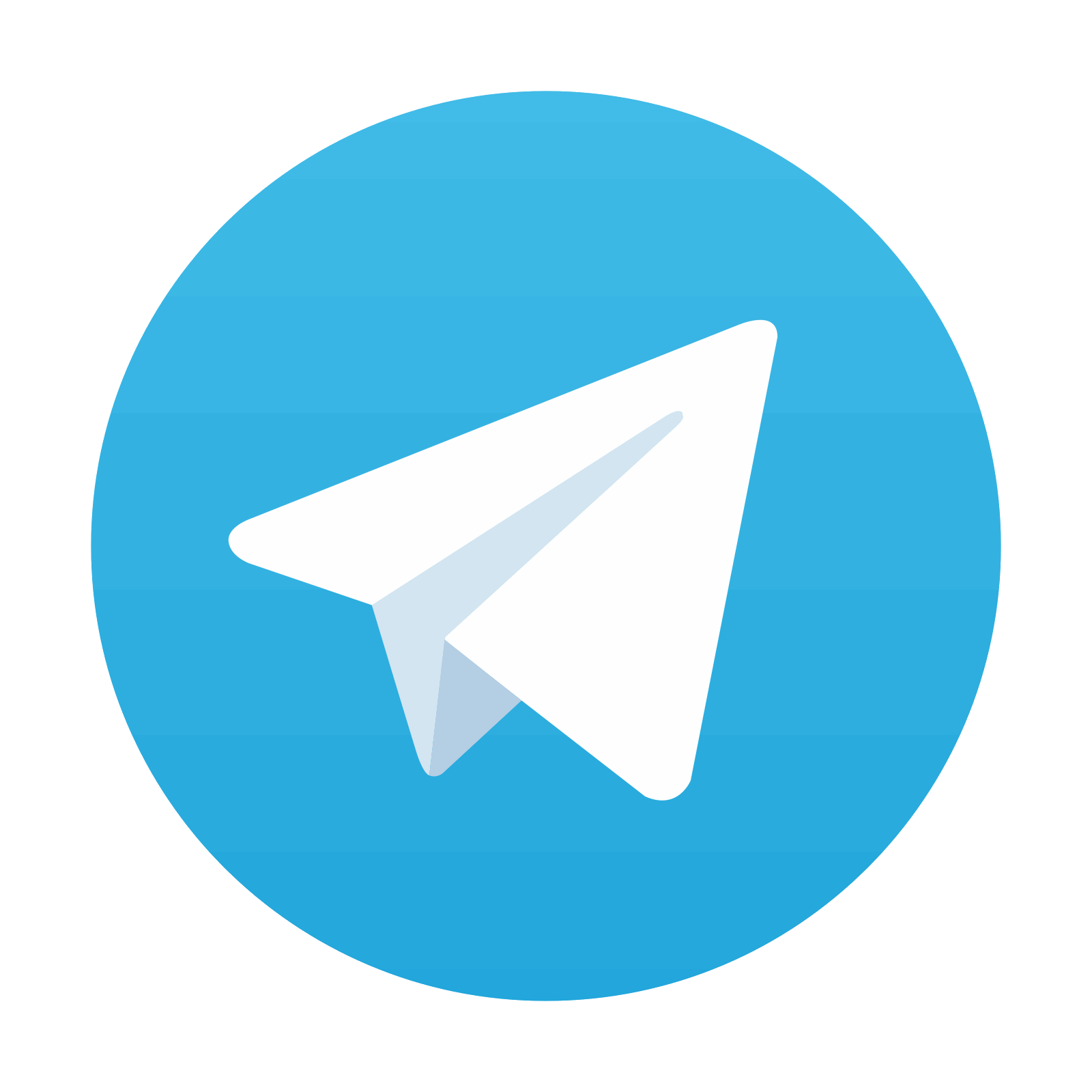
Stay updated, free articles. Join our Telegram channel

Full access? Get Clinical Tree
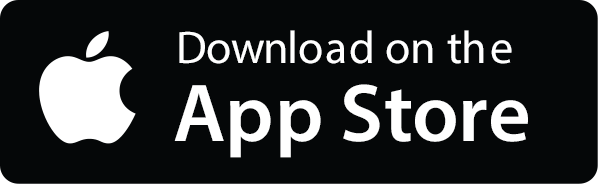
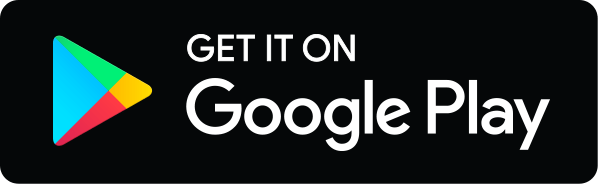