© Springer International Publishing Switzerland 2017
Claus Cursiefen and Albert S. Jun (eds.)Current Treatment Options for Fuchs Endothelial Dystrophy10.1007/978-3-319-43021-8_11. Epidemiology and Genetic Basis of Fuchs Endothelial Corneal Dystrophy
(1)
Cornea & External Disease Division, Wilmer Eye Institute, Johns Hopkins Medical Institutions, 600 N. Broadway, Woods 376, Baltimore, MD 21287, USA
1.1 Introduction
Fuchs endothelial corneal dystrophy (FECD) was first described in 1910 by Austrian ophthalmologist Ernst Fuchs. In his report “Dystrophia Epithelialis Corneae,” Fuchs described a series of 13 patients that developed progressive central corneal clouding with loss of corneal sensation and formation of epithelial vesicles resulting in eventual vision loss [14]. While he gave the name “Dystrophia epithelialis” based on his hypothesis that the primary site of pathology was at the level of the corneal epithelium, Prof. Fuchs understood that the name could later be “replaced by a better one, when the true nature of the disease [was] discerned” [14, 25]. Since his initial description, significant progress has been made over the past century in our understanding of the epidemiology, genetics, and pathophysiology of this endothelial dystrophy.
1.2 Epidemiology
FECD is a progressive disease characterized by the loss of endothelial cells, thickening of Descemet membrane, formation of guttae, and development of corneal edema resulting in a significant decrease in visual acuity. In its most severe manifestation, blindness ensues [32]. In a global survey of corneal transplantation and eye banking by Gain et al., FECD was the primary indication for all corneal transplantations performed worldwide, accounting for 39 %, followed by keratoconus (27 %) and infectious keratitis sequelae (20 %) [16]. Data from the Eye Bank Association of America have similarly identified FECD as the most common indication for corneal transplantation, accounting for 22 % of all corneal transplants in the USA [54].
Clinical progression of FECD is typically graded using the Krachmer grading scale, which is divided from Grade 0–5, with Grade 0 indicating 0–12 central guttae; Grade 1, greater than 12 central non-confluent guttae; Grade 2, 1–2 mm of confluent central guttae; Grade 3, 2–5 mm of confluent central guttae; Grade 4, greater than 5 mm of confluent central guttae; and Grade 5, greater than 5 mm of confluent guttae with stromal or epithelial edema [36]. Limitations to the Krachmer grading scale include interobserver variability in grading as well as the development of stromal thickening or edema in stages other than Grade 5 [11].
Another staging system commonly utilized divides FECD into four stages of clinical progression [1]. In Stage 1, the patient is asymptomatic, but slit-lamp biomicroscopy demonstrates the presence of central guttae, variable amount of pigment on the endothelial surface, and a thickening and gray appearance to Descemet membrane. In Stage 2 of the disease, the patient develops a painless decrease in visual acuity and glare, particularly upon awakening, related to the presence of epithelial and stromal edema. In Stage 3, the patient may experience episodes of pain due to the formation and rupture of epithelial and subepithelial bullae. Lastly, in Stage 4, visual acuity is severely compromised and may be reduced to hand motions with the development of avascular subepithelial scarring which limits bullae formation. At this stage, the patient is generally free of painful episodes.
Based on the time of onset of the disease, FECD is divided into two clinical subtypes: an early onset and late-onset forms. The early onset form of FECD is rare and has been associated with autosomal dominant Q455K, Q455V, and L450W mutations in the gene encoding the alpha 2 subunit of collagen 8 (COL8A2) [4, 18, 40, 49]. COL8A2 is an important structural component of Descemet membrane. Patients with early onset FECD can exhibit changes in the first decade of life, with continued progression over the next two to three decades [21]. In contrast to late-onset FECD, in which there is a strong female predominance, men and women are equally affected in the early onset form, a reflection of the autosomal dominant pattern of inheritance.
Late-onset FECD is more common than the early onset form. In a 1967 study of 1,016 people without any known ocular pathology in Jacksonville, Florida, Lorenzetti et al. reported a 3.9 % prevalence of confluent corneal guttae in individuals aged 40 or older [42]. This estimate was based on slit-lamp examination alone. In the Reykjavik Eye Study, the prevalence of any primary central corneal guttae in Icelandic individuals over the age of 55, based on both slit-lamp and specular microscopy, was 11 % for females and 7 % for males [86]. In the Kumejima Study, the prevalence of any central corneal guttae in Japanese residents age 40 years or older, based on specular microscopy alone, was 4.1 % overall [22]. There was a significant difference in the prevalence of central corneal guttae between Japanese men and women, 2.4 % versus 5.8 %, respectively [22]. Interestingly, a retrospective histopathologic study by Santo et al. found that the frequency of FECD in corneal specimens from Japanese patients undergoing keratectomy or keratoplasty over a 34-year period from 1959 through 1992 was only 0.7 % [66]. The low frequency of FECD among Japanese individuals needing corneal transplantation may in part relate to their significantly higher corneal endothelial cell density as compared to an age-matched American population [43].
Unlike early onset FECD, a female predominance of 2.5–3.5:1 has also been reported in late-onset FECD [2, 13, 31, 86]. Patients with late-onset FECD typically manifest changes by the fifth decade of life, with progression over the next two to three decades [21]. Although the median age of onset for the late-onset form of FECD is at least four decades later than that of the early onset form, both display a similar linear rate of disease progression, with advancement in clinical severity grade occurring on average every 5 years [18].
The use of central corneal thickness (CCT) to grade FECD is challenging given the frequent absence of baseline CCT data and variability of CCT in a population. Additionally, the use of ultrasonic pachymetry to measure CCT is limited by the ability to consistently measure corneal thickness at the same location [45]. However, as suggested by data from Kopplin et al., monitoring of CCT changes in patients may be useful in following FECD progression [35]. In contrast to the subjective morphological clinical grading of FECD, for which interobserver agreement is only moderate, work by Repp et al. has suggested that the ratio of the central corneal thickness to the peripheral corneal thickness at 4 mm from the center (CPTR4), which functions as an internal reference, may serve as an objective and repeatable metric to assess the severity of FECD [58]. While the presence of corneal edema has traditionally only been reflected at the end stage of the Krachmer grading scale [36], the presence of corneal edema is not specific to end-stage disease and can be seen in early clinical grades of FECD, underscoring the importance and incorporation of these data into clinical grading schemes [58].
Data from Fujimoto et al. have further suggested that monitoring of guttae in the peripheral zone, 3.7 mm peripheral to the center, was significantly correlated with disease grade in advanced cases of FECD [15]. The degree of guttae in the central and paracentral zones (0.6 mm from center), which are damaged earlier and more severely in FECD, was not found to be useful in distinguishing between advanced cases (grades 3–5), as these areas had nearly complete guttae formation by this stage [15]. Monitoring guttae formation in the periphery may therefore serve an important role for monitoring disease progression in advanced stages of FECD.
Several risk factors in addition to gender have been associated with the development of central guttae and degree of FECD. In the Reykjavik Eye Study, which was a cross-sectional, random, population-based study of individuals 55 years and older, participants with more than a 20 pack/year smoking history had a 2.2-fold increased risk of development of any primary central guttae [86]. Higher weight and body mass index were additionally associated with a 2.5 and 7 % respective decrease in the risk of any central corneal guttae [86]. In the Fuchs Genetics Multi-Center Study, both female sex and smoking were associated with an increased risk, 34 % and 30 %, respectively, of advanced FECD (grades 4–6) [85]. The association of smoking with increased risk of FECD may be explained by increased generation of reactive oxygen species and oxidative damage in the setting of already impaired antioxidant defense [27, 79]. While associations between FECD and age-related macular degeneration [57] as well as cardiovascular disease [53] and angle-closure glaucoma [56] have been reported, subsequent reports have not been able to confirm such associations [5, 86].
1.3 Genetic Basis of FECD
Fuchs corneal endothelial dystrophy (FECD) is a complex and heterogeneous genetic disorder of the corneal endothelium with variable expressivity and incomplete penetrance. Two genetic techniques – linkage analysis and genome-wide association study (GWAS) – have been used to elucidate the genetic basis for FECD. At this time, five causal genes (TCF4, AGBL1, LOXHD1, SLC4A11, and ZEB1) and four causal loci on chromosomes 5, 9, 13, and 18 have been identified in individuals with late-onset FECD. In addition, mutations in COL8A2 have been shown to cause early onset FECD.
1.3.1 Causal Mutations
1.3.1.1 TCF4
Variations in the transcription factor 4 (TCF4) gene are the most significant genetic risk factors associated with FECD. This gene, located on chromosome 18q21.1, is a member of the E-protein family of basic helix-loop-helix (bHLH) transcription factors that function in the regulation of multiple developmental pathways including neurogenesis and lymphocyte development [7, 17, 38, 51]. TCF4 has a complex gene organization with 34 exons that produce tissue-specific isoforms that regulate both positive and negative gene expressions [67]. The full-length 671-amino acid TCF4 isoform has an N-terminal transcriptional activation domain (AD1), followed by a nuclear localization signal (NLS), a second transcriptional activation domain (AD2), and a C-terminal basic helix-loop-helix domain that mediates dimerization and E-box binding. Alternative splicing at the 5-prime end results in TCF4 proteins with 18 different N-termini. All isoforms have AD2 and bHLH domains but may lack the AD1 domain and/or NLS.
In 2012, Wieben et al. demonstrated a strong genetic association between intronic cytosine-thymine-guanine (CTG) trinucleotide repeats (CTG18.1) in the TCF4 gene of FECD patients [80]. They found that 79 % of FECD patients had greater than 50 repeats in the third intron of the TCF4 gene compared to just 3 % of unaffected control individuals. This trinucleotide repeat (TNR) expansion was found to be highly predictive of the disorder conferring more than a 30-fold increase in the risk for development of FECD in Caucasians. Cosegregation of the triplet repeat expansion with FECD was found in 15 of 29 Caucasian pedigrees with complete penetrance supporting the triplet repeat expansion as being the likely causal genetic variant [69].
Subsequent genetic studies provide evidence that the TCF4 CTG18.1 repeat expansion is the major causal variant across ethnic groups. In a Singapore Chinese cohort, expansion at the CTG18.1 locus was the only variant identified within the TCF4 gene in Fuchs patients suggesting that the expanded CTG18.1 allele was the primary and possibly the sole causal variant at this gene locus in the Chinese population [82]. Genetic analysis of Indian FECD patients also found a highly significant association of CTG18.1 alleles with FECD and a minor association with the single nucleotide polymorphism rs17089887. The study found the disease threshold of 50 trinucleotide repeats present in 34 % of FECD subjects and 5 % of control subjects [52]. Together these studies indicate that expansion of CTG18.1 is likely to be the most significant global cause of FECD. Furthermore, disease severity was found to be greater in FECD cases with the CTG18.1 triplet repeat expansion compared to those without the expansion. The length of CTG triplet repeat also positively correlated with the Krachmer grade of severity. Thus, TCF4 triplet repeat expansion presence and length has predictive clinical and surgical therapeutic implications [69].
While the expansion of the intronic TCF4 allele plays a key role in the development of FECD, the molecular mechanism of the disease has not been established fully. Multiple mechanisms have been proposed by which the expansion in the TCF4 gene may cause susceptibility to FECD. It has been suggested that the expanded allele could interfere with transcription initiation and/or splicing of TCF4 in corneal endothelial cells altering expression levels of specific TCF4 isoforms. TCF4 also plays a key role in epithelial-to-mesenchymal transition through its regulation of zinc finger E-box binding homeobox 1 (ZEB1) protein also known as transcription factor 8 (TCF8) [65]. ZEB1 has been proposed to regulate expression of extracellular matrix components, such as COL8A2, and to induce epithelial-to-mesenchymal transition (EMT) that drives metastatic cancers. The discovery of repeat-associated non-ATG translation in several microsatellite disorders also raises the possibility that the pathogenesis of FECD may also be due to small peptides generated from the poly(CUG)n RNA [8].
More recent studies point to RNA aggregation and toxicity as the basis for endothelial cell death [10, 50]. Accumulated RNA transcripts with expanded repeats can form stable hairpin structures that bind and sequester RNA interacting proteins [74]. Corneal endothelial cells from FECD patients, like cells from patients with the trinucleotide repeat disorder myotonic dystrophy type 1 (DM1), accumulate poly(CUG)n RNA in foci within the nucleus [10, 50]. Poly(CUG)n RNA foci co-localized with and sequestered the mRNA-splicing factor MBNL1 leading to aberrant processing of essential MBNL1-regulated mRNAs including some mRNAs with roles in EMT. The RNA foci and depletion of RNA-binding proteins trigger aberrant splicing and ultimately stimulate apoptosis. Importantly, RNA nuclear foci were not detected in cells from FECD patients who lack the repeat expansion highlighting the central role that CUG RNA nuclear foci play in the pathogenesis of FECD with the CTG18.1 triplet repeat expansion in TCF4 [50]. Tissue-specific pathogenesis in FECD patients may be related to the corneal endothelium’s exposure to an environment subjected to higher levels of oxidative stress. Oxidative stress, previously implicated in the pathogenesis of FECD [3, 27, 79], may cause somatic instability that further contributes to the expansion of the TNRs [10].
1.3.1.2 AGBL1
An additional causal mutation that results in dominant late-onset Fuchs corneal dystrophy was recently identified on the long arm of chromosome 15 at position 25.3, the location of the ATP/GTP-binding protein-like1 (AGBL1) gene [61]. Linkage analysis followed by next-generation sequencing uncovered a heterozygous nonsense mutation (R1028X) and a missense mutation (C990S) in the AGBL1 gene in individuals with FECD. AGBL1 encodes an isoform of cytosolic carboxypeptidase 4 (CCP4), a metallocarboxypeptidase that mediates deglutamylation of target proteins. CCP enzymes catalyze the deglutamylation of long polyglutamate side chains generated by posttranslational polyglutamylation on proteins such as tubulins and myosin light chain kinase (MYLK) [64]. Studies in Purkinje cells obtained from mice that lack a functional CCP1 isoform display microtubule hyperglutamylation that leads to neurodegeneration [78]. These findings demonstrate that regulation of the length of the polyglutamate side chains on tubulin plays a role in neuronal cell survival. Further study is needed to determine if mutations in AGBL1 identified in patients with FECD also result in microtubule hyperglutamylation and if AGBL1 mutations play a role in the survival of corneal endothelial cells. Functional analysis demonstrated that AGBL1 interacts specifically with the FECD-associated protein TCF4 and not TCF8, and that AGBL1 mutations diminish that interaction [61].
1.3.1.3 LOXHD1
The lipoxygenase homology domain-containing 1 (LOXHD1) gene encodes a highly conserved protein consisting entirely of polycystin/lipoxygenase/alpha-toxin (PLAT) domains, thought to facilitate protein targeting to the plasma membrane. The cytogenetic location of the LOXHD1 gene is on chromosome 18q21.1. In 2012, Riazuddin et al. identified LOXHD1 as a causal gene within a family whose disease was previously linked to the undefined FCD2 locus [60]. The LOXHD1 gene is not located in the FCD2 locus region, and expanded linkage analysis and sequencing of a targeted region of chromosome 18 uncovered a missense mutant allele in LOXHD1 that caused progressive hearing loss as the causal variant leading to Fuchs dystrophy in this pedigree. A screen of a cohort of >200 sporadic FECD-affected individuals identified an additional 15 heterozygous missense mutations not found in over 800 control chromosomes. Mutations were modeled to the surface of the protein and were indicative of impaired protein-protein interactions. Expression of LOXHD1 mutant alleles in cells produced prominent cytoplasmic aggregates similar to what is seen in the corneal phenotype. Amino acid substitutions found in the protein due to these rare alleles in LOXHD1 could explain the pathogenesis of FECD and provide an understanding of the mechanism by which mutations in the same locus can produce diverse phenotypes.
1.3.1.4 SLC4A11
The SLC4A11 gene, located on chromosome 20p12, was reported to encode a voltage-regulated, electrogenic sodium-coupled borate cotransporter essential for borate homeostasis, cell growth, and cell proliferation [55]. However, the molecular function of SLC4A11 remains to be clearly elucidated. Recent studies indicate that neither bicarbonate nor borate is a substrate for SLC4A11, but it has been shown to be a NH3/H+ cotransporter with unique properties [84]. Mutations in SLC4A11 have been associated with a number of endothelial corneal dystrophies including recessive congenital hereditary endothelial dystrophy 2 (CHED2), corneal endothelial dystrophy with sensorineural deafness (Harboyan syndrome), and dominant late-onset Fuchs endothelial corneal dystrophy [9, 62, 76, 77].
A series of heterozygous mutations of the SLC4A11 gene are associated with late-onset FECD [62, 70, 77]. In endothelial cells, the transporter is located in the basolateral membrane and was shown to facilitate transmembrane water movement [75]. FECD causal mutations expressed in cells display defective cell surface localization with correlated endoplasmic reticulum accumulation by biochemical assays and confocal immunolocalization [77]. SLC4A11 knockout mice did not show any abnormalities in the endothelium and Descemet membrane with the primary phenotypic change observed in the cornea being an increase in the height of basal epithelial cells [41].
1.3.1.5 ZEB1
ZEB1 is a zinc finger E-box binding homeobox 1 transcription factor, also known as TCF8, located at chromosome 10p11.22. ZEB1 was first shown to play a role in transcriptional repression of interleukin 2 [71, 81] and later found to be important for the induction of epithelial-mesenchymal transition (EMT) [83]. Pathogenic ZEB1 mutations were first identified as a cause of posterior polymorphous corneal dystrophy-3 (PPCD3) [37]. Later ZEB1 mutations were found to be associated with FECD when Mehta et al. screened 74 unrelated Chinese individuals with FECD for ZEB1 mutations [46]. A novel variant, p.N696S, was present in only one of the FECD cases and was not present in any of the other FECD cases or in 93 control individuals [46]. A second casual mutation in ZEB1 was uncovered by Riazuddin et al. in a large multigenerational family whose disease also linked to the FCD4 locus [63]. Four additional ZEB1 pathogenic mutations were identified in 384 unrelated FECD-affected individuals by sequence analysis [63]. Comparison of ZEB1 mutations supports a genotype-phenotype correlation where FECD causal mutations are missense mutations in exons of ZEB1 at sites that are moderately to highly evolutionarily conserved in vertebrates, and mutations associated with PPCD3 are more deleterious frameshift, nonsense, or lost-start-codon mutations [39]. In vivo studies in zebra fish embryos showed that two of five FECD causal mutations could partially rescue developmental abnormalities caused by morpholino oligonucleotide knockdown of endogenous ZEB1. The other three variants demonstrated phenotypes identical to morpholino injection alone, while wild-type mRNA completely rescued the phenotype [23]. Expression of ZEB1 nonsense and missense mutations that cause PPCD3 and FECD, respectively, in a corneal endothelial cell line support that haploinsufficiency is a cause of PPCD3. Some of the PPCD3 causative mutations expressed truncated ZEB1 proteins that display decreased protein levels and/or impaired cellular localization. In contrast, FECD-associated ZEB1 missense mutations did not significantly alter protein levels or nuclear localization. The mechanisms by which ZEB1 missense mutations lead to FECD remain to be elucidated [6].
1.3.2 FECD Loci Identified by Genetic Linkage Analysis
An early locus (FCD1) linked to late-onset FECD was localized on chromosome 13 to a 26.4-Mb interval between 13pTel and 13q12.13 in a large four-generation Caucasian pedigree that included 13 affected and three unaffected individuals from three generations [73]. Females were observed with the expected 2:1 sex ratio in affected individuals. Of note, two children in this family whose parents were both affected demonstrated clinical signs of late-onset FECD at a young age. Sundin et al. also identified a second locus, FCD2, at chromosome 18q21.2–18q21.32 using linkage analysis of three large families [72]. However, the disease-linked haplotype was different in each family, suggesting that there may be heterogeneity of this locus. FECD in the families associated with chromosome 18 exhibited an autosomal dominant inheritance biased toward women, with 27 affected women and 19 affected men within the three families. In addition, 10 % of individuals with the disease haplotype were unaffected clinically, suggesting a complex mode of inheritance associated with this locus. A third locus, FCD3, localized to 5q33.1–5q35.2 in a three-generation family [59]. FECD in this family was present in eight females and two males. The FCD4 locus was identified by Riazuddin et al. in the region 9p22.1–9p24.1 [63]. Additional mutations in TCF8 were also discovered in some of these affected family members suggesting interaction between two pathologic alleles in this disease. Additional loci have been identified which may contain alternative rare FECD mutations [12].
FCD1 is linked to the most severe and rapidly progressing form of the disease with individuals experiencing a 24 % annual increase in the number of guttae versus a 5 % annual increase for FCD2. FCD3 causes the mildest form of the disease that progresses more slowly than both FCD1- and FCD2-linked diseases [23]. Cosegregation of the rare, late-onset FCD4 haplotype on chromosome 9p with missense mutations of TCF8 results in severe FECD with poor prognosis [63].
1.3.3 COL8A2 Early Onset FECD
In 2001, Biswas et al. studied a multigenerational family with an early onset, autosomal dominant form of FECD and discovered a single nucleotide missense mutation at position 1364 of the coding sequence of the α2 collagen VIII (COL8A2) gene [4]. This nucleotide change results in a glutamine to lysine (Q455K) substitution in the triple helical domain of the COL8A2 protein. This mutation was the first characterization of the molecular basis of any corneal endothelial dystrophy and the first pathogenic variant of COL8A2 in humans [4]. A second autosomal dominant COL8A2 mutation in families with early onset FECD was uncovered in 2005 [18]. This transversion, also located in the collagen repeat domain of the COL8A2 protein, replaces leucine with tryptophan at residue 450 (L450W). A third heterozygous mutation, resulting in a glutamine to valine substitution at amino acid 455 (Q455V), was found in patients of Korean pedigrees with FECD and not in unaffected individuals. Replacement of a polar glutamine with a nonpolar valine is suggested to disrupt the interaction of COL8A2 and COL8A1 proteins in the hexagonal lattice structure of Descemet membrane (DM) [49]. The female-to-male ratio was found to be 1:1 for COL8A2 mutations, in contrast to late-onset FECD pedigrees where the disease was more prevalent in females with a female-to-male ratio of 2.5:1 [18].
Type VIII collagen is comprised of α1 and α2 polypeptide chains [20, 24]. Collagen VIII is synthesized, processed, and secreted by corneal endothelial cells and is the major protein of DM [28]. Type VIII collagens contribute to assembly and structural integrity of the hexagonal matrix that serves to support the endothelium. In addition, studies suggest collagen VIII also contributes to cell differentiation and plays a role in determining cell phenotype [68]. In early onset FECD associated with the L450W mutation, an excess of both α1 and α2 collagen VIII proteins are deposited, increasing the thickness of the anterior banded layer of DM more than three times that found in normal DM [19]. The increase in COL8A2 protein is accompanied by increased levels of collagen IV, fibronectin, and laminin that contribute to the increase in membrane thickness [19, 44].
Jun et al. created and analyzed transgenic mice carrying the Col8a2 Q455K mutation [26]. At 5 and 10 months of age, mutant mice began to display hallmark FECD features seen in human disease, including variations in cell size, deviation from the normal hexagonal shape, cell loss, and the presence of DM guttae. Ultrastructural analysis revealed the presence of extremely dilated endoplasmic reticulum (ER), pointing to the activation of ER stress and unfolded protein response. Further biochemical studies confirmed unfolded protein response (UPR) activation and UPR-associated apoptosis in the mutant corneal endothelium [26]. They concluded that the Q455K mutation in COL8A2 causes FECD through a mechanism involving the UPR and UPR-associated apoptosis. Features of Col8a2 L450W transgenic mice have been described [47]. These mice displayed a milder corneal endothelial phenotype than that of homozygous Q455K mice yet still exhibited the hallmarks of FECD. Both mutants displayed dilated rough ER and upregulation of UPR pathway genes and proteins. Reverse transcriptase polymerase chain reaction (RT-PCR) analysis of corneal endothelial cells from L450W and Q455K mutant mice revealed upregulation of DNA damage-regulated autophagy modulator protein 1 (DRAM1), a lysosomal membrane autophagy marker. RT-PCR of human FECD endothelium provides evidence for upregulation of DRAM1 relative to autopsy control corneas. Taken together, these findings suggest that altered autophagy contributes to FECD pathogenesis. While mutations in COL8A2 are not associated with late-onset FECD, the shared features of the early and late-onset forms of the disease suggest that the study of COL8A2 mutations may provide insights into pathogenesis and potential therapeutic directions.
1.4 Future Therapies
Since the first description of Fuchs endothelial corneal dystrophy over one century ago, corneal transplantation has remained the only definitive treatment for this disease. To date, medical treatments for FECD have been aimed at reducing corneal edema and associated blurred vision. The use of 5 % sodium chloride eye drops or ointment to draw fluid out of the cornea, as well as directing warm air toward the cornea from a hair dryer at arm’s distance to facilitate evaporation of fluid, has assisted with transient symptomatic visual improvement. Over the past few decades, however, significant strides have been made in furthering our understanding of the pathophysiological mechanisms underlying FECD and have highlighted several potentially novel areas of pharmacological treatment.
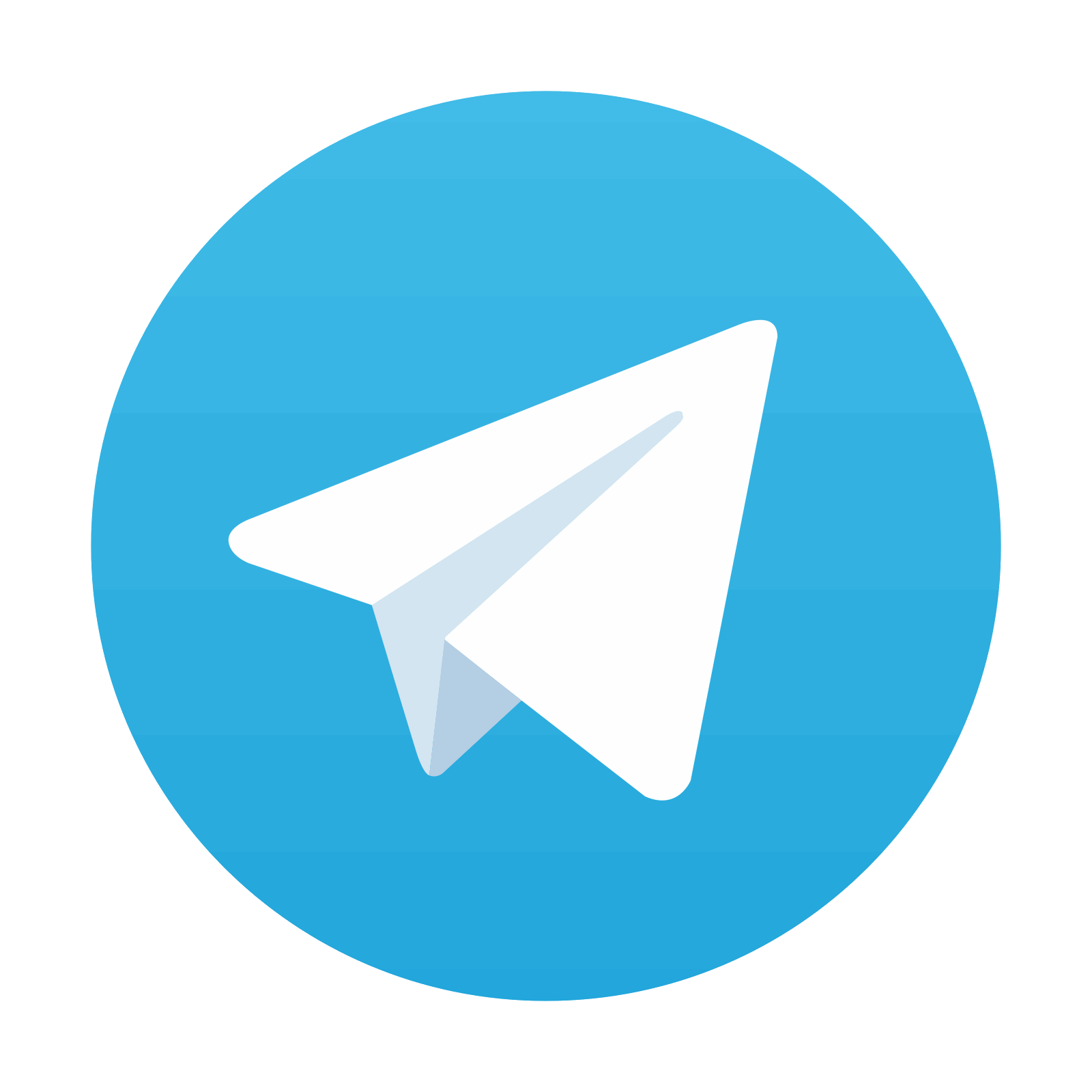
Stay updated, free articles. Join our Telegram channel

Full access? Get Clinical Tree
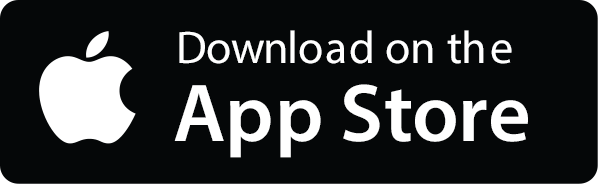
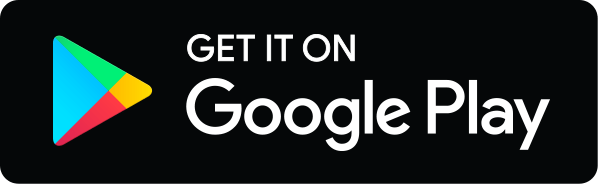